Academia.edu no longer supports Internet Explorer.
To browse Academia.edu and the wider internet faster and more securely, please take a few seconds to upgrade your browser .
Enter the email address you signed up with and we'll email you a reset link.
- We're Hiring!
- Help Center
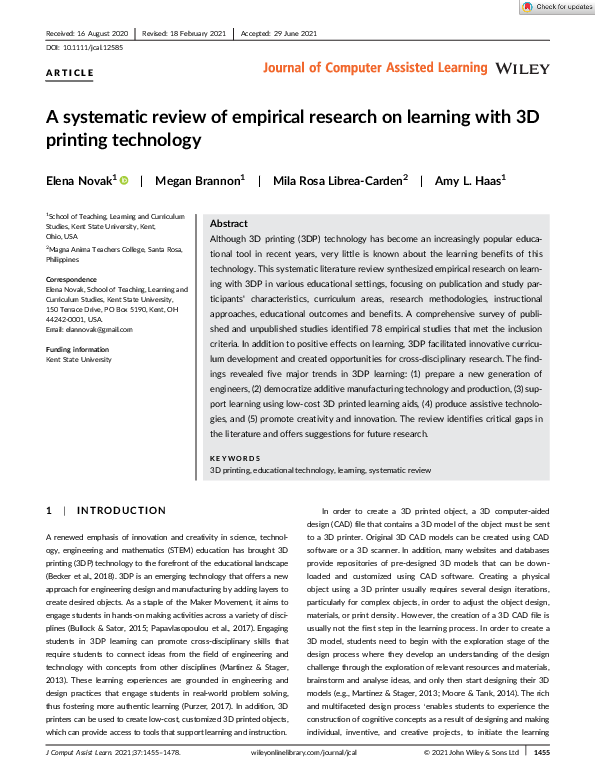

A systematic review of empirical research on learning with 3D printing technology

2021, Journal of Computer-Assisted Learning
Although 3D printing (3DP) technology has become an increasingly popular educational tool in recent years, very little is known about the learning benefits of this technology. This systematic literature review synthesized empirical research on learning with 3DP in various educational settings, focusing on publication and study participants' characteristics, curriculum areas, research methodologies, instructional approaches, educational outcomes and benefits. A comprehensive survey of published and unpublished studies identified 78 empirical studies that met the inclusion criteria. In addition to positive effects on learning, 3DP facilitated innovative curriculum development and created opportunities for cross-disciplinary research. The findings revealed five major trends in 3DP learning: (1) prepare a new generation of engineers, (2) democratize additive manufacturing technology and production, (3) support learning using low-cost 3D printed learning aids, (4) produce assistive technologies, and (5) promote creativity and innovation. The review identifies critical gaps in the literature and offers suggestions for future research.
Related Papers
Additive Manufacturing
The emergence of additive manufacturing and 3D printing technologies is introducing industrial skills deficits and opportunities for new teaching practices in a range of subjects and educational settings. In response, research investigating these practices is emerging across a wide range of education disciplines, but often without reference to studies in other disciplines. Responding to this problem, this article synthesizes these dispersed bodies of research to provide a state-of-the-art literature review of where and how 3D printing is being used in the education system. Through investigating the application of 3D printing in schools, universities, libraries and special education settings, six use categories are identified and described: (1) to teach students about 3D printing; (2) to teach educators about 3D printing; (3) as a support technology during teaching; (4) to produce artefacts that aid learning; (5) to create assistive technologies; and (6) to support outreach activities. Although evidence can be found of 3D printing-based teaching practices in each of these six categories, implementation remains immature, and recommendations are made for future research and education policy.
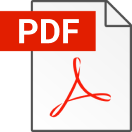
Eusebio Reyero
Tod Colegrove
Rapid Prototyping technology enables the active construction of new knowledge in a way that may be a good fit for the academic library; beyond simply an opportunity to to lead the way technologically on campus, the addition of such resources may enable students and faculty to leverage the multidisciplinary skills and competencies needed to innovate and compete in today's rapidly changing environment. A brief review of the 3D printer selection process at UNR and a discussion of the current state of the 3D printer market will be covered. Is rapid prototyping a new multidisciplinary literacy poised to enable and transform learning and knowledge creation across the Sciences and Engineering on campus? The DeLaMare Science & Engineering Library is actively exploring the question; in this session the impact and reception of the library's recent introduction of multiple 3D printers and scanners will be presented, including real-life examples drawn from users' experience.
Sustainable Current Approaches in Architectural Science and Technology
Myint Swe Khine and Nagla Ali (Eds.), Integrating 3D printing into Teaching and Learning: Practitioners’ Perspective
Elena Novak
3D printing technology is a powerful learning tool that can involve students in active learning, design thinking, and problem solving. It creates opportunities for integrating science, engineering, technology and mathematics with other disciplines. In this chapter, we describe the benefits of 3D printing technology for teaching science and discuss a theoretical framework for designing 3D printing problem- and project-based learning in science followed by practical recommendations for creating 3D printing instructional units that can be integrated into a formal curriculum. In conclusion, we provide an example of a 3D printing project that was implemented with preservice elementary teachers in a science methods course.
International Journal of Art & Design Education
Marjo Virnes
International Journal of Computer Applications
Christos Drosos
Chelsea Schelly
Open-source 3D printing provides a cost efficient means of STEM education.These technologies can also empower student-driven engaged learning.Report on workshop for science educators to build 3D printers for their classes.Teacher workshop augmented with online instructional and visual tools.Results indicate transformative potential of these technologies in the classroom.3-D printing technologies have the potential to improve both Science, Technology, Engineering, and Mathematics (STEM) education and Career and Technical Education (CTE), as well as integrating these two educational emphases and providing opportunities for cross-curriculum engagement. The objective of this study is to investigate the potential of open-source (OS) technologies in an educational setting, given the combination of economic constraints affecting all educational environments and the ability of OS design to profoundly decrease the cost of technological tools and technological innovation.This paper reports on a 3-day workshop augmented with online instructional and visual tools designed for middle school and high school level educators from a wide array of disciplines (including traditional science, math, and engineering as well as computer, shop, and art). Teachers (n=22) submitted applications to participate in the workshop, the workshop was observed for both evaluation and research, teachers participated in focus groups (n=2) during the workshop in order to discuss their interest in OS 3-D printing technology and its potential role in their classrooms, and teachers completed a voluntary post-workshop survey and responded to follow-up after printers were in the classroom for one year.During the workshop teachers built 3-D printers using OS technologies that they were then able to take back to their schools and into their classrooms.Through workshops augmented with online instructional and visual tools designed to provide facilitated yet self-directed engagement with a new, relatively unknown, and relatively complex technology, paired teacher teams were able to successfully build and use RepRap 3-D printers based on OS design in just three days.Here, we discuss both what the teachers learned and what we learned from the teachers regarding the potential for educators to construct OS 3-D printing technologies as a tool of empowering and transformative education.Open-source 3-D printing technologies have the potential to improve education through a sense of empowerment resulting from active participation, as well as through cross-curriculum engagement.
Education Sciences
Khalifa Harib
Three-dimensional (3D) printing can revolutionize the way products have been designed and manufactured. This necessitates engineering graduates equipped with the knowledge and skills of 3D printing. As a result, the educational aspects of 3D printing have earned a great deal of attention. Nevertheless, to teach 3D printing in an undergraduate engineering degree program, an outcomes-oriented approach integrating engineering design, object visualization/digitization, and 3D printing domains can be used. Accordingly, this study presents a tutorial development method to teach undergraduate engineering students the knowledge and skills of 3D printing. The method integrates the abovementioned domains maintaining a hierarchy among the seven ABET-prescribed outcomes. The hierarchy organizes the outcomes into three levels (primary, secondary, and tertiary). The presented method is implemented by introducing a tutorial where a spur gear-pinion pair is designed, visualized, digitized, and 3D p...
Book Chapter
3D printing technology is an emerging educational technology that is becoming increasingly available in schools, public libraries, museums, and higher education institutions. Oftentimes, 3D printers are underutilized because instructors have a limited experience with this technology and do not know how to integrate it into curricula. This chapter describes how 3D printing technology can be (1) introduced in a science teacher preparation program as a means of engaging prospective elementary teachers in active, collaborative, problem-based learning and (2) integrated into an existing science curriculum. We designed a 3D Printing Science Project to model for prospective teachers a lesson that they could implement with their future students in the elementary science classroom. After completing the project, prospective teachers reported a moderately high usefulness and ease of use of 3D printing technology and significantly higher design thinking abilities and attitudes toward science and teaching science. An analysis of participants' project reflections, classroom discussions, and 3D printed objects provided a further insight into their collaborative design experiences.
RELATED PAPERS
International Journal of Applied Earth Observation and Geoinformation
Nontembeko Dudeni-Tlhone
International Journal of Research in Business and Social Science (2147- 4478)
Willy Muturi
ScienceAsia
Somchit Eiam-Ong
Journal of the Society of Powder Technology, Japan
Akira Ohgami
Marcos Antonio
Jurnal Biologi Tropis
Putri Alfianti Sriwinahyu
ACTA ZOOLÓGICA MEXICANA (N.S.)
Erick Alexander contreras Ruiz
Biotechnology for Biofuels
Somayeh Farzad
Nguyen-thong Dang
INSANILLAHIA AUDIA FAJAR
Nature Communications
davide giuseppe sangiovanni
Journal of Antimicrobial Chemotherapy
Mette Skriver
F1000Research
Niranjan Konduri
Russian Linguistics
Elmira Zhamaletdinova
Clara Eka Putri
Medicentro Electronica
Jorge Luis Quiros Hernandez
1比1定做aston毕业证书 阿斯顿大学毕业证学位证书样板原版一模一样
Temas em Educação: entre questões recorrentes e possibilidades
Pimenta Cultural
Blucher Engineering Proceedings
Janaina Ruffoni
Industrial Crops and Products
Berta Heinzmann
RELATED TOPICS
- We're Hiring!
- Help Center
- Find new research papers in:
- Health Sciences
- Earth Sciences
- Cognitive Science
- Mathematics
- Computer Science
- Academia ©2024
Organizational adoption of 3D printing technology: a semisystematic literature review
Journal of Manufacturing Technology Management
ISSN : 1741-038X
Article publication date: 10 December 2020
Issue publication date: 17 December 2021
Three-dimensional (3D) printing (3DP) offers a promising value proposition across multiple manufacturing industries. Despite the variety of production benefits the technology entails, its rate of adoption is still low compared to industry forecasts. In face of this challenge, industry as well as academia requires more information and guidance. This review aims to examine the characteristics of the existing body of research on the organizational adoption of 3DP as well as its underlying theoretical concepts. The most common criteria driving adoption will be derived, such as to facilitate the managerial decision-making process. Pathways for future research will be presented.
Design/methodology/approach
This study underlies a bibliometric literature review and additionally applies content analysis to systematically investigate the existing body of research and group decision criteria along the four major pillars of strategic decision-making.
The contributions of this paper are threefold. First, the bibliometric analysis reveals interesting aspects of the existing body of research. The most prominent characteristics of the contemporary literature are reflected along descriptive indicators, such as industry, method, model, origin, research outlet or adoption drivers, thus granting relevant insights into academia and practice. Second, the most notable adoption models are carefully analyzed on their inherent attributes and their application fit for the context of organizational 3DP adoption. Findings, for instance, revealed the dominance of diffusion of innovation (DOI) across the existing body of research and divulge that this construct is generally applied in combination with user-centered decision frameworks to yield more precise results. Third, an ample range of opportunities for future research are detected and thoroughly explained. Among others, the authors identified a clear lack of information on the impact environmental variables and contingency factors exerted on the organizational adoption of 3DP. Guidance in relation to the sourcing of industry data, usage of adoption frameworks and avenues for future scientific projects is supplied.
Originality/value
This study represents the first semi-systematic literature review on the organizational adoption of 3DP. Thus, it not only offers a valuable evaluation guide for potential adopters but also determines a future research agenda.
- Decision-making
- Manufacturing technology
- Organizational change
- Additive manufacturing
- 3D printing
- Technology implementation
Ukobitz, D.V. (2021), "Organizational adoption of 3D printing technology: a semisystematic literature review", Journal of Manufacturing Technology Management , Vol. 32 No. 9, pp. 48-74. https://doi.org/10.1108/JMTM-03-2020-0087
Emerald Publishing Limited
Copyright © 2020 2020, Desiree Valeria Ukobitz
Published by Emerald Publishing Limited. This article is published under the Creative Commons Attribution (CC BY 4.0) licence. Anyone may reproduce, distribute, translate and create derivative works of this article (for both commercial and non-commercial purposes), subject to full attribution to the original publication and authors. The full terms of this licence may be seen at http://creativecommons.org/licences/by/4.0/legalcode 1 Department of Psychology, University of Konstanz, Konstanz, Germany 2 Department of Psychology, New York University, New York, USA 3 Institute of Psychology, Leuphana University Lüneburg, Lüneburg, Germany
1. Introduction
What is the current body of research regarding organizational adoption of 3DP in terms of descriptive indicators?
Which adoption theory and underlying decision criteria are most frequently applied for explaining organizational adoption of 3DP and what do we learn from these studies?
Where do we still lack knowledge, and therefore, which future research opportunities can be identified?
Existing literature reviews merely analyze publications on 3DP in terms of their frequency and count across different disciplines ( Gupta and Dhawan 2018 ). While the global research output in the field of 3DP amounted to 7300 papers in 2016, work examining 3DP from a management-science perspective is far more limited. Gupta and Dhawan (2018) in their assessment of 3DP research output encountered 372 publications on 3DP from the fields of business, accounting and management (2007–2016). Unfortunately, this study does not examine the underlying content in depth and as such does not generate learnings for theory and practice. Until to date, management science has not yet contemplated any review of literature on the organizational adoption of 3DP, i.e. the decision-making processes leading to 3DP adoption in firms. Knowledge on the adoption decision is yet fragmented, and unified sources of information to support the organizational decision-making process are scarce. As this area of investigation matures, the consolidation of existing knowledge on the underlying phenomenon is required more than ever. In an effort to fill this gap, this study aimed to examine the existing body of research on organizational 3DP adoption by means of a bibliometric analysis of predefined performance indicators, as well as a critical review of the theoretic constructs was employed to understand the phenomenon. Untapped opportunities of research are defined, and pathways for future studies are determined.
Until to date, the empiric evidence required to conduct a comprehensive literature review on the organizational adoption of 3DP was insufficient. However, during the last five years initial research exploring 3DP adoption through qualitative and quantities methods (e.g. Oettmeier and Hoffman, 2017 ; Schniederjans, 2017 ) evolved and granted first results on the distinct criteria affecting intrafirm adoption. Although the majority of qualitative studies employed explorative approaches to generate knowledge on this new field of research, recent work applied quantitative methods based on large empiric samples. These empiric analyses either applied firm-centered adoption models such as the technology–environment–organization (TOE) framework by Tornatzky and Fleischer (1990) (e.g. Yeh and Chen, 2018a ), the diffusion of innovation (DOI) theory by Rogers (1983) (e.g. Marak et al. , 2019 ) or user-centered decision frameworks, such as the unified theory of acceptance and usage of technology (UTAUT) by Venkatesh et al. (2003) (e.g. Schniederjans and Yalcin, 2018 ) or the technology acceptance model (TAM) by Davis (1985) (e.g. Chaudhuri et al. , 2018 ) to examine organizational decision-making. These models contrast not only in terms of the dependent- and independent variables but also in the underlying units of analysis. While some constructs study the action of adoption ( Tsai and Yeh, 2019 ), others merely examine the intention to adopt ( Oettmeier and Hofmann, 2017 ). Besides this characteristic, the dimensions impacting the adoption decision differ to a great extent. While the research study employing user-centered models emphasizes the impact of the individual decision-maker characteristics on the adoption decision ( Steenhuis and Pretorius, 2016 ), firm-centered models highlight the influence of external stakeholders ( Tsai and Yeh, 2019 ). Hence, a holistic overview of adoption criteria and drivers among firms would require analyzing the phenomenon from multiple theoretic angles.
The contributions of this paper are threefold. First, the bibliometric analysis reveals interesting aspects of the existing body of research. The most prominent characteristics of contemporary literature are reflected along descriptive indicators, such as industry, method, model, origin, research outlet or adoption drivers, thus granting relevant insights into academia and practice. Second, the most notable adoption models are carefully analyzed on their inherent attributes and their application fit for the context of organizational 3DP adoption. Findings, for instance, revealed the dominance of DOI across the existing body of research and divulge that this construct is generally applied in combination with user-centered decision frameworks to yield more precise results. Third, an ample range of opportunities for future research are detected and thoroughly explained. Among others, we identified a clear lack of information on the impact environmental variables and contingency factors exerted on the organizational adoption of 3DP. Guidance in relation to the sourcing of industry data, usage of adoption frameworks and avenues for future scientific projects is supplied.
The rest of this review is structured as follows. Section 2 provides an overview of the theoretical background underlying this investigation. The state of the art of 3DP is introduced, and the most commonly applied theoretic models for the adoption of technology are presented. Section 3 discloses the research methodology and explains the data collection and analysis process. Then, the descriptive results are presented and both quantitative findings from the reviewed publications, as well as the results from the latent content analysis, are demonstrated. Section 4 and 5 synthesize the results and discuss future research pathways. Finally, implications and limitations are presented.
2. Theoretical background
2.1 organizational vs consumer 3dp.
3DP has proliferated in the last 15 years among society and has gained vast attention, both, on an individual as well as on an organizational level. 3DP adoption among consumers ( Steenhuis and Pretorius, 2016 ), either as a facilitator to home fabrication ( Anastasiadou and Vettese, 2019 ) or as entrepreneurial starter kit ( Gartner et al. , 2015 ), has found increased awareness among research. Extensive media coverage and decreasing printer costs amplified the diffusion of 3DP on a consumer level. Hence, decision drivers and benefits of 3DP for individual consumers are widely studied ( Fox, 2014 ). The organizational adoption of 3DP, on the other hand, displays a rather untapped area of investigation. 3DPsystems and prices vary drastically depending on their purpose of usage, i.e. desktop or industrial application. Thus, decision-making for the adoption of 3DP on a firm level represents a complex process that involves the allocation of elevated financial and human resources, as well as organizational risks. A much broader range of decision drivers need to be considered for technology adoption on a firm level than on a user level ( Schniederjans, 2017 ). Turbulent market dynamics and firm-internal structures constitute only some of the complexities that need to be contemplated for the organizational adoption of novel technologies ( Tornatzky and Fleischer, 1990 ). Further investigation on this evolving field of research is required to support the managerial decision-making process and as such promotes the diffusion of 3DP among industry.
2.2 Understanding the technology adoption process
Multiple theoretical frameworks have been developed over time to study and understand the phenomenon of technology adoption from an empirical point of view. Depending on the unit upon analysis, these theories either observe adoption from an organizational or an individual perspective. To understand the phenomenon of organizational 3DPT adoption, this paper studies six theories of technology adoption. These frameworks have been selected based on their abundance as well as acceptance among technology adoption research. Among the most commonly discussed models are the theory of planned behavior (TPB) ( Ajzen, 1985 ), the TAM ( Davis, 1985 ), the UTAUT ( Venkatesh et al. , 2003 ), the TEO model ( Tornatzky and Fleischer, 1990 ), the DOI theory ( Rogers, 1983 ) and the institutional theory (IT) ( DiMaggio and Powell, 1983 ; Scott, 1995 ). Table 1 provides an overview of these adoption theories, their aim, drivers, dependent variable, applicability for organizational adoption, applicability among 3DP research as well as references. Dependent variables vary from intention to adopt to adoption behavior, thus emphasizing either actual or potential 3DP adoption.
2.2.1 Theory of planned behavior
The TPB aims to explain and predict human behavior , i.e. action. TPB assumes a behavioral intention prior to the actual behavior and hypothesizes that this intention is influenced by the individual’s attitude toward the behavior , the individual’s subjectively perceived norm of what should be done ( subjective norm ) and the individual’s degree of control over the factors influencing the behavior ( perceived behavioral control ) ( Ajzen, 1985 ). Although TPB was not designed to study technology-related decisions, the theory still sets the basis for major studies on human behavior and as such contributes to extended research on technology adoption among individual members of society. TPB explains the intention to adopt rather than the actual adoption behavior; thus, the time frame between intention and behavior is unknown. Both Schniederjans and Jalcin (2018) as well as Chatzoglou and Michailidou (2019) in their research on the organizational adoption of 3DP have included elements of TPB, such as to understand management’s attitude toward using 3DP. Both scientists studied the managerial intention to adopt 3DP by means of the managers’ attitude toward and perception of the technology. A major limitation of TPB is the lack of account for environmental or economical dimensions of influence on the intention to adopt 3DP. As such, TPB merely represents a tool to predict managerial behavior prior to actual adoption. Research on the organizational adoption of 3DPT has employed TPB only in combination with other adoption models, contemplating additionally for technological, organizational or environmental dimensions (e.g. DOI, TAM and UTAUT).
2.2.2 Technology acceptance model
The TAM aims to predict how users accept and employ technology and draws on behavioral aspects of TPB. TAM hypothesizes that technology usage is influenced by the attitude toward technology usage and consequently the intention to use the technology . The model claims that an individual’s attitude toward technology usage is derived from the user’s perceived ease of use (PEOU) and perceived usefulness (PU) of the technology, and it further acknowledges that external variables influence and moderate the relationship between PU and PEOU ( Davis, 1985 ). The body of research on technology adoption often criticizes the limited predictability of TAM and thus expanded the model to TAM II, including the variables of social influence and cognitive instrumental processes ( Venkatesh and Davis, 2000 ). While the users’ perceived ease of use as well as the perceived usefulness of 3DP represent helpful dimensions in understanding acceptance or rejection of 3DP, they do not explain actual adoption. Commonly labeled as intention-behavior gap, intention is an insufficient prerequisite for a successful action ( Sheeran and Webb, 2016 ). Similar to TPB, TAM I and II represent models that aim to analyze the adoption intention of individual members of society as opposed to organizations. TAM does not take environmental and organizational drivers, such as market dynamics or human resources, into consideration. As such, contemporary research on organizational 3DP adoption has employed TAM mostly in combination with the DOI theory ( Rogers, 1983 ), such as to constitute for the lack of context ( Wang et al. , 2016 ; Oettmeier and Hofmann, 2017 ; Marak et al. , 2019 ). Contemporary research often employs TAM to better comprehend the 3DP adoption intentions among top-management members ( Schniederjans and Yalcin, 2018 ). So far only TAM I has been studied by the underlying body of literature on organizational 3DP adoption.
2.2.3 Unified theory of acceptance and usage of technology
The unified theory of acceptance is based on various aspects of the aforementioned models and aims to explain the behavior of people in their use of technology. UTAUT is acknowledged as one of the most complete frameworks for predicting adoption behavior among individual members of society. UTAUT proposes that usage intention and facilitating conditions directly influence technology usage . Furthermore, the model suggests that the technology usage intention is directly determined by three key constructs ( performance expectancy, effort expectancy and social influence ). Additionally, four moderator variables ( gender, age, experience and voluntariness of use ) impact the relationship between the different exogenous and endogenous variables ( Venkatesh et al. , 2003 ). The increased amount of moderator variables is frequently criticized as artificially improving UTAUTs predictability ( Dwivedi et al. , 2019 ). Similar to TAM, UTAUT is also suffering from the intention-behavior gap, thereby examining intention to use a technology rather than its actual adoption. Alike TPB and TAM, UTAUT may also be applied to study the individual behavior of managers in organizations; however, it does not consider the impact of technology adoption on the organization from a holistic point of view. Despite the inclusion of social influence (e.g. society) and facilitating conditions (e.g. resources) as independent variables defining an individual's intention to adopt, organizational dynamics (e.g. competitors and human resources) and external dimensions (e.g. market and environment) are not contemplated. Hence, to reliably examine the phenomenon of organizational 3DPT adoption, research combined UTAUT with the DOI theory ( Rogers, 1983 ) ( Schniederjans, 2017 ; Marak et al. , 2019 ).
2.2.4 Diffusion of innovation theory
The DOI theory analyzes how, why and at what rate new ideas or technology diffuse among a social system over time ( Rogers, 1983 ). DOI contributes the adoption decision to innovation-specific criteria and suggests that decision-makers undergo a thorough evaluation of the technology´s characteristics, both firm internal as well as external. As such, DOI theorizes that a technology’s relative advantage for the adopting organization, its compatibility with existing technological structures, its perceived complexity , the observability of technology-induced success as well as its anteceding triability all impact the adoption decision ( Rogers, 1983 ). As opposed to TPB, TAM and UTAUT, DOI acknowledges the context upon which technology adoption-decisions are taken and as such constitutes a proper theory for analyzing organizational technology adoption. DOI represents the most frequently applied method for examining the adoption of 3DP in organizations ( Oettmeier and Hofmann, 2017 ; Schniederjans, 2017 ; Chaudhuri et al. , 2018 ; Marak et al. , 2019 ). As DOI aims to understand the DOI among a social system over time, the theory focuses on the bigger picture of adoption rather than emphasizing on multiple distinct firm-internal and external drivers. Scientists employ this method to obtain a broad overview of the determinants of 3DP adoption in firms ( Chatzoglou and Michailidou, 2019 ). While the theory already comprehends a vast range of drivers for organizational technology adoption, still insufficient emphasis is put on the impact of environmental factors ( Hsu et al. , 2006 ), such as stakeholders influence, industry infrastructure or governmental regulation. Especially when examining the adoption of 3DP, environmental dimensions constitute major decision drivers (e.g. governmental funding/subsidies and commercial partner infrastructure). Moreover, research on 3DP adoption frequently combines DOI with individual adoption models (TAM and UTAUT), such as to dive deeper into understanding of individual decision-maker’s motivations ( Wang et al. , 2016 ). All in all, DOI is well-suited for generating an overview on the drivers for 3DP adoption in firms; however, if more profound insights are required, further specific dimensions need to be added to the model (e.g. environmental, organizational and individual) to obtain profound insights.
2.2.5 Technology–organization–environment framework
The TOE framework identifies three crucial aspects that influence technology adoption within an organization. TEO argues that technology- organizational- and environmental-related factors drive adoption. Technological factors describe the perceived characteristics of the technology in terms of its relative advantage for the organization and compatibility with existing structures. Organizational-related motives refer to internal characteristics such as firm size, financial and human resources, internal structure and future vision as well as outlook. Ultimately, environmental factors define all firm-external drivers such as industry dynamics, competitors, trading partners and authorities ( Tornatzky and Fleischer, 1990 ). As opposed to the aforementioned models, TEO examines the technology adoption decision rather than the mere intent of adoption. TEO is frequently employed to examine the adoption of radical technology and also found initial application in the literature on the adoption of 3DP (e.g. Yeh and Chen, 2018a ). The framework is consistent with DOI in terms of its general drivers, however complements the model through its emphasis on the environmental context ( Wang et al. , 2016 ). TEO, on the contrary to DOI, delves deep into the impact of industry stakeholders, governmental entities, market trends or legislation on the organizational adoption decision. Although TEO does not specify decision-maker characteristics to the extent of DOI, TPB, TAM or UTAUT, the management’s experience, vision and support receive attention in the variables constituting the organizational context. The literature on the organizational adoption of 3DP has employed TEO on its own. While no combinations with other adoption models have yet been conducted in this area of research, Tsai and Yeh (2019) and Yeh and Chen (2018) have added the independent variable of 3DP cost to the construct. As TOE has been developed specifically to examine complex technology adoption decisions from an organizational perspective, it emphasizes all processes and variables that impact the adopting entity as part of an industrial ecosystem. As such, extended research on technology adoption manifested that TEO is more appropriate to analyze intrafirm adoption than DOI ( Hsu et al. , 2006 ).
2.2.6 Institutional theory
IT argues that firm-external pressures lead to organizational actions and behavior ( DiMaggio and Powell, 1983 ). The scientific field of innovation and technology management frequently draws back to sociology and as such to IT to observe the phenomenon of isomorphism in decision-making (e.g. Teo et al. , 2003 ). IT hypothesizes that coercive, normative, and mimetic isomorphic pressures exerted by an organization's environment influence firm’s internal decisions. While coercive forces result from trading partner behavior, normative pressure is exerted from industry authorities and mimesis arises from competitor actions ( DiMaggio and Powell, 1983 ; Scott, 1995 ). As such, IT acknowledges that organizational decisions are not only driven by performance goals (e.g. relative advantage) but also by social and cultural factors. To emphasize the influence of stakeholder dynamics on adoption decisions, research on radical technology, frequently applies IT in combination with other adoption models (e.g. Yoon and George, 2013 ; Cao et al. , 2014 ). In order to highlight the impact of institutional pressures exerted on the adopting entity (e.g. from trading partners, competitors and institutions), the literature oftentimes proposes integrating IT in the context of environment as proposed by TEO ( Soares Aguiar and Palma-dos-Reos, 2008 ; Oliveira and Martins, 2011 ). Until to date, only one investigation examined the organizational adoption of 3DP by means of IT ( Schniederjans and Yalcin, 2018 ). Although IT constituted one of many models that have been employed by Schniederjans and Yalcin´s (2018) study, compelling evidence was found for the impact of isomorphic pressures on the adoption of 3DP in organizations. Unfortunately, no quantitative research has yet analyzed the 3DP adoption from an institutional lens. IT embodies a powerful approach to delve into the frequently overlooked impact of institutional forces on technology adoption decisions.
3. Research methodology
What is the current body of research regarding organizational adoption of 3DP in terms of descriptive indicators (methods and models applied, industries investigated, research activity along country and time, research outlet and frequency of drivers)?
Which adoption theory and underlying decision criteria are most frequently applied for explaining organizational adoption of 3DP, and what do we learn from these studies?
Where do we still lack knowledge, and therefore which future research opportunities can be identified in the field of organizational adoption of 3DP.
3.1 Description of analysis process
Once the purpose of the present research was established, the literature selection process was initiated. Screening for inclusion criteria was developed to identify the most appropriate literature for analysis ( Fink, 2014 ). The analysis commenced with an extensive keyword search to source relevant literature studies on the adoption of 3DP technologies. The databases employed were Business Source Complete, Science Direct and Web of Science. The predefined criteria were applied to reduce the search to the most relevant publications in the field of organizational 3DP adoption. Afterward, the articles were quantitatively analyzed on their year of publication, publication outlet, authorship and origin, underlying research methods and applied theoretical frameworks as well as industries upon investigation. This was followed by the content analysis of the underlying drivers for 3DP adoption. The information was coded and synthesized to a higher level of dimensions based on the TOE framework ( Tornatzky and Fleischer, 1990 ).
3.2 Search and selection process
The focus of this paper lies on the understanding of the organizational decision-making process for the adoption of 3DP. Thus, the following keyword string was generated to conduct the search across the three aforementioned databases: (“ 3D printing ” OR “ additive manufacturing ” ) AND (“ adoption ” OR “ decision ” OR “ usage ” OR “ application ” ) . While it was set as a prerequisite for the selected titles to include either the term 3DP or additive manufacturing , the keywords invariably had to include the label adoption OR decision OR usage OR application . The keyword diffusion was not included on purpose, as it represents the macro phenomenon of how many entities of the population have already adopted the technology over time. The search identified a total of 594 fully available articles.
To assure high quality and applicability of the research articles prespecified inclusion criteria were applied to the literature search ( Fink, 2014 ). First, the years of publication were restricted from 2010 to March 2020, as hardly any empiric research on the adoption of 3DP resulted before that year. The number of articles reduced to 483. Second, the search was narrowed down to peer-reviewed articles such as to guarantee scientific rigor of articles (401 articles). Next, we limited the search to English-only publications, thereby reducing the body of literature to 392. Fourth, we reduced the selection to articles originating from management journals only, excluding chemistry, biomedical and in-depth engineering outlets as these hardly draw attention to organizational decision-making processes. In total, 85 articles matched all the criteria and were selected for further content analysis. In the fifth step, we scanned the abstracts of all obtained articles on their fit for the underlying research. Research studies that did not strictly emphasize the organizational process of adopting 3DP and associated decision drivers were excluded. First, we omitted articles discussing the adoption of 3DP among individuals rather than organizations (49). Second, while articles based on quantitative research methods had to apply at least one adoption model, papers based on qualitative analysis had to discuss either drivers, barriers or other factors impacting the organizational adoption decision. As such, and for example, articles analyzing the impact of 3DP on supply chain and inventory or articles examining the adoption, advantages and disadvantages of different 3DP techniques in organizations were removed. In conclusion, 25 publications were identified and selected for this review. We conducted a backward search of all 53 articles to study the referenced literature for any further research ( Levy and Ellis, 2006 ). A total of two further studies were detected, summing up to a final total of 27 papers. In total, two additional research papers that would not have passed the predefined screening criteria have been identified by informal sources and included due to the fact that their results were of interest for the study, making a total of 29 articles. Next, the final selection of papers was evaluated on their quality ( Fink, 2014 ). Qualitative and quantitative research was treated differently. While quantitative research was assessed on the underlying data collection methodology as well as reliability and validity of results, qualitative research was evaluated on the explicitness, comprehensiveness and reproducibility of the employed empirical methodology. No papers were found to lack reliability or empiric evidence. Figure 1 graphically illustrates the selection process and reveals corresponding data.
3.3 Data extraction and analysis
To conduct the bibliometric literature review as well as the underlying content analysis, data needed to be extracted in a systematic manner. Qualitative and quantitative research had to be treated differently ( Rousseau et al. , 2008 ). To further proceed with the analysis, a spreadsheet database was generated. The bibliometric results of the selected articles were thoroughly examined, and the following information was retrieved: article title, authors, location and affiliation, journal, date of publication, keywords, research type, research method, theoretical framework and industry. Microsoft Excel was employed to synthesize and visually represent the recaptured data. Next, data were extracted for the content analysis. In this step, the adoption criteria were obtained from quantitative and qualitative studies. In terms of quantitative research, only those criteria that tested significant on the adoption decision were selected. Qualitative studies were subjected to individual researcher’s judgment; thus, the most frequently cited and evidenced decision criteria were extracted. For the sake of analysis, the selected articles were systematically analyzed, and data were coded according to predefined schemes. The TEO framework was employed to schematize the criteria underlying the organizational adoption of 3DP. Retrieved data were categorized following a deductive category approach and organized in terms of technology-, organization- and environment-specific drivers. The coding process was carried out in MAXQDA v.12. Finally, the results were quantitatively analyzed on their frequencies.
4. Descriptive results
4.1 trend among publications in time.
The literature review confirmed the novelty of the field of 3DP adoption for academia. As shown in Figure 2 , research activity was relatively thin before 2015. The topic first received attention in 2013, however hardly continued in the scientific radar for the next three years. The data observe that the adoption of 3DP from a business and innovation science perspective gained increased interest from 2015 onwards and peaked in 2018. This trend resonates with the overall rate of the technology’s diffusion among industry. Before 2010, 3DP was used mainly by the high-tech sector for rapid prototyping or concept proof. As a result of increased media exposure, the emergence of sophisticated 3DP suppliers as well as decreasing printer costs, the technology has heavily started to enter more mainstream industry after 2010 ( Sculpteo, 2019 ). Thus, empiric data on adoption behavior and trends became available only some years after.
4.2 Publication outlet
Figure 3 illustrates the most frequently employed journals in the field of 3DP adoption. Most articles have been published in Journal of Manufacturing Technology Management (5). An equal number of papers were found in Technology Forecasting and Social Change, International Journal for Production Economics and International Journal for Production Research (3). In total, two publications brought to our awareness by informal sources have been obtained through university databases (doctoral and master thesis). The remaining publications pertained to highly specific journals in the field of innovation management or manufacturing. These findings agree with Bradford’s law observing that a core of journals produces approximately a third of all articles ( Eyers and Potter, 2015 ).
4.3 Authorship and location
In total, 71 authors contributed to the identified literature on 3DP adoption. Approximately 45% of the identified body of research was elaborated by two authors and an additional 21% by three authors. Single-authored articles contributed only to 17% of the grand total, followed by contributions from four and five researchers. Only four out of 29 papers were developed through crosscountry collaborations and mostly constituted interEuropean research alliances. The primary authors country of residence was a selected as main location. The adoption of 3DP was analyzed by academics across various countries; however, it flourished in the USA and Europe.
4.4 Research methods and theoretical framing
The selected articles were furthermore examined on their underlying research methods. Figure 4 illustrates the distribution of publications based on the methodology employed as well as the year published. As visible, the early years of investigation were characterized by explorative research, conducted either through desk research, semistructured interviews or case studies. As a result of the topic's novelty and the consequent lack of empirical evidence, the most commonly employed research method is the in-depth interview with a total number of 12 publications. Once 3DP adoption diffused among industry, the first quantitative data became available and allowed survey-based research methods to consolidate in 2019. Moreover, the first empirical results derived through adoption theories emerged in 2016 along with quantitative studies. Desk research appeared consequently throughout the last ten years, indicating a consistent diffusion of 3DP across organizations.
Figure 5 illustrates the adoption models that have been employed to analyze the organizational adoption of 3DP along the different research methods. The DOI framework was the most employed theory among the underlying body of research, tightly followed by the technology acceptance model. The UTAUT was resulted as the third most-frequently applied model among publications. The TEO model as well as the TPB were both employed by an equal number of publications. Only one paper considered IT for analyzing 3DP adoption. While 76.5% of all quantitative research papers were based on their analysis on specific adoption models, only 47.7% of qualitative papers did so. Half of the publications represent explorative approaches that did not employ adoption theories. In total, eight publications combined indicators and aspects from multiple models. While six papers combined the TAM and the DOI theory, three articles merged DOI and UTAUT. Thus, DOI, UTAUT and TAM represent the most combined concepts among the underlying body of research.
4.5 Industries upon analysis
All reviewed publications based on qualitative and quantitative research methods focused their empirical analyses either on one or multiple industries ( Figure 6 ). Only four articles emphasized their investigation on a single industry, and one-fourth of these articles applied a qualitative research approach. The remaining publications under review, especially all quantitative research papers, revealed data corresponding to multiple industries. This may be the result of the novelty of the topic and still the limited amount of information on specific industries. In total, 15 articles investigated the organizational adoption of 3DP in an industrial manufacturing setting. These publications however did not state any further sub-categorization. The transport industry (automotive and aerospace) was analyzed by one-third of all articles, closely followed by the consumer good industry (textile, jewelry, furniture and sports equipment) and the health and medical sector. Additional settings upon analysis were represented by the electronic, chemical and construction industry. One study can analyze several industries.
4.6 3DP adoption criteria
This section provides a categorization of the criteria influencing the adoption of 3DP and highlights the most interesting learnings associated with these decision drivers. While an ample range of theories have been applied to study 3DP adoption (see Figure 5 ), the underlying research aims to display the most recurrent adoption criteria along the TEO framework, as it represents one of the most appropriate models for analyzing intrafirm technology adoption ( Hsu et al. , 2006 ). As described in section 3.3 , the underlying extraction process differed among qualitative and quantitative studies. Out of the quantitative studies, only those criteria that proved significant in the empirical analysis were identified and counted for the underlying research. In the qualitative studies, those criteria were selected/counted because the authors of the studies found those to be the most influencing ones. As suggested by Weber (1990) criteria were coded as words. Following a deductive approach ( Neuendorf, 2017 ), the criteria were extracted and schematized along three dimensions. The technology context includes all factors that relate to the benefits and barriers, and the technology per se represents for the organization in terms of performance, impact and agility. The organizational context defines all company-internal aspects that impact the adoption decision, both positively as well as negatively. As such, these criteria refer to structural requirements (i.e. size, budget and processes) as well as to organizational readiness (i.e. top management support, technology readiness and experience). Finally, the environmental context describes all firm-external factors that impact the adoption decision. Validity is established through data triangulation, as different sources of data have been reviewed to develop the analysis ( Neuendorf, 2017 ). Table 2 illustrates the critical factors for adoption along three categories and indicates their frequency among the publications selected for this literature review.
4.6.1 Technology context
As observed in Table 2 , technology context represents the most prominent category of adoption drivers with a frequency of 167. Investment costs ( f = 16) represent the most dominant barriers to adoption in terms of frequency. Literature points out high technology acquisition costs, unexpected maintenance costs as well as increased material pricing as important aspects of 3DP adoption (e.g. Yeh and Chen, 2018 ). This is followed by concerns on the technological maturity of 3DP ( f = 13). In this context, the scientific community commonly observes the lack of standardization among printers and output quality (e.g. Weller et al. , 2015 ). In total, six articles emphasized the slowness of the production process that seems rather uncompetitive when compared to traditional manufacturing methods (e.g. Fontana et al. , 2019 ). The review reveals that the technology-specific benefits still outweigh the barriers in terms of frequency among articles. The majority of papers emphasize the possibility to accelerate time to market ( f = 16) through 3DP and elaborates on its positive impact on lead- and ramp-up times as well as manufacturing cycles (e.g. Schniederjans, 2017 ). The opportunity to simplify supply chains ( f = 11), thereby lowering inventory and production steps, as well as skipping tooling and molding functions, represents a further highly quoted driver for 3DP adoption (e.g. Oettmeier and Hofmann, 2017 ). Among the most prominent criteria for 3DP, adoption is the ability to customize products for end users ( f = 9) (e.g, Murmura and Bravi, 2018 ). Cohen (2014) states that 3DP allows the mass customization of up to 200 products a time. The reduction of the environmental impact ( f = 9) through the additive manufacturing technique (zero waste), the possibility to experience absolute design freedom ( f = 9) and create complex products or the option to manufacture small production batches ( f = 4), among others, represent further benefits driving 3DP adoption (e.g. Marak et al. , 2019 ). Interestingly, the limitation in terms of size of printed products and printers per se was only pointed out twice (e.g. Weller et al. , 2015 ). While the organizational context observes the importance of skilled human resources, only one publication mentioned software usage (CAD) as a barrier to 3DP adoption in organizations ( Garza, 2016 ).
4.6.2 Organizational context
Organizational drivers represent the second most important category for adoption among the reviewed literature ( f = 56). The most frequently quoted aspect for 3DP adoption among investigated cases is the organizational readiness ( f = 12). Among others, organizational readiness refers to the firms' willingness to adopt 3DP, its experience with similar technology and the degree of internal rejection (e.g. Candi and Beltagui, 2019 ). The existence of skilled workforce and the concomitant necessity of reskilling existing workforce ( f = 11) exhibit the second most mentioned factor impacting 3DP adoption from an organizational perspective (e.g. Chaudhuri et al. , 2018 ). Furthermore, it seems of utmost importance to evaluate the technologies compatibility ( f = 9) with existing production systems as well as the overall fit with the company’s overall mission and structure (e.g. Tsai and Yeh, 2019 ). The literature also repeatedly acknowledges the support and experience of top management teams ( f = 7) as well as the importance of a dynamic organizational culture ( f = 5 ) for3DP adoption (e.g. Mellor et al. , 2014 ). Some articles further emphasized the importance of the alignment among firm-internal departments ( f = 4), such as manufacturing and IT for 3DP adoption. An increased company size was found to be both, a promoter as well as an inhibitor of organizational 3DP adoption (e.g. Kianian et al. , 2016 ). Steenhuis et al. (2020) manifested the impact of company age and location on the adoption behavior.
4.6.3 Environmental criteria
Although, highly significant, environmental decision criteria appear less frequently among the 3DP literature and only represent a total of 31 quotes in this research. This results from the limited application of adoption models emphasizing firm-external decision drivers such as TEO or IT, among the underlying body of literature. Oettmeier and Hoffman (2017) emphasize the impact of coercive forces ( f = 5) exerted by trading partners, competitors ( f = 3) as well as the overall effect of social influence ( f = 5) on the adoption decision. Existing research repeatedly acknowledges market and technology turbulence ( f = 4 ) as well as the overall competitiveness of the industrial environment as adoption triggers (e.g. Candi and Beltagui, 2019 ). Various papers referred to facilitating conditions ( f = 5) (governmental or regulatory support and training initiatives) as incentive for 3DP adoption (e.g. Oettmeier and Hofmann, 2017 ). The readiness of the 3DP supplier landscape, in terms of number of technology and material vendors ( f = 4), also appears to play an important role for organizational technology adoption ( Tsai and Yeh, 2019 ). Furthermore, compliance with evolving market trends and expectations ( f = 2) was found as a driver to adoption ( Yeh and Chen, 2018a ).
5. Discussion of results
Although the organizational adoption of 3DP has gained increased scientific interest from 2015 onwards, our findings show that this field is still in its infancy. This understanding resonates with the overall diffusion of 3DP technology among industry ( Wohlers Associates, 2019 ). Until to date, research has been dominated by qualitative methods, such as to generate a common understanding of the topic and yield first explorative results on the factors driving 3DP adoption in organizations. Quantitative research activity was commenced in 2016, along with the availability of industry data, and since then it is employed in multiple adoption models to study the phenomenon in an empiric manner.
5.1 Theoretic constructs
While the most commonly applied adoption theory across the underlying body of research was the DOI, almost all studies combined this model with at least with one additional construct (TPB, UTAUT or TAM. TPB, UTAUT and TAM are user-centered decision models that emphasize the perception and characteristics of an individual toward an action, i.e. technology adoption. We discovered that, besides analyzing the impact of the technology per se (e.g. relative advantage) through DOI, this specific combination stresses the influence of top management on the adoption decision. The dimensions driving technology adoption among firms however differ vastly from user-centered adoption processes. Thus, they require a more holistic approach to analyze not only the technology and the individual decision-maker but also the organizational and environmental characteristics a firm is immersed into. The preceeding theoretic constructs, however, hardly study the adoption decision from an institutional angle. The TOE model and the IT on the other hand contribute organizational decision-making to environmental factors of influence. Even though the body of literature on the organizational adoption of radical technologies frequently emphasizes the impact of firm-external conditions on the adoption decision ( Wang et al. , 2010 ; Cao et al. , 2014 ), this topic has hardly experienced any discussion in the field of 3DP.
5.2 Data characteristics and origin
Our analysis suggests that as opposed to DOI, TPB, UTAUT and TAM, TEO and IT require industry-specific data to yield most adequate results. Industry homogeneity across the sample is a prerequisite to obtain conclusive and generalizable results on the impact of industry dynamics on technology decision-making. Due to the novelty of the field, industry data are still premature. Most quantitative studies have acquired their data from organizations pertaining to multiple industries. Only four out of 29 papers have employed a single-industry approach. Such heterogeneous results neither allow to identify differences in organizational behavior across industries nor to propose industry-specific adoption plans. The limited rate of diffusion across industries ( Steenhuis et al. , 2020 ) and the associated lack of information represent a major impediment for investigating the phenomenon from an holistic angle. We conclude that corporate adoption decisions ultimately have to be examined from an environmental, organizational, and technological point of view. We propose TEO as the most complete option for analyzing the phenomenon of 3DP adoption in organizations. Besides the integration of industry specific variables, TEO also represents the only model that analyzes the action of adopting a technology rather than the intent to adopt. This again is a reflection of existent industry data and sample characteristics. While research based on DOI, TPB, UTAUT and TAM included 3DP adopters and nonadopters in their sampling process, TEO emphasizes only those cases that have already taken a decision. Studying adoption, as opposed to hypothetical adoption, allows to draw conclusions on the actual impact of firm-internal and external drivers on the adoption decision.
5.3 Adoption drivers
Content analysis furthermore revealed that the technological dimensions, as compared to the organizational or environmental dimensions, of influence have received most attention among the scientific community. More than half of all researched articles recognized the significance of technology-related adoption drivers, such as the ability of 3DP in accelerating time to market, its ease of use and the associated simplification of supply chains. Furthermore, a third of all articles acknowledged the firm’s overall innovativeness, the existence of skilled work and characteristics of top-management as critical decision drivers. Contrarily, hardly any discussion occurred on the influence of organizational contingency factors (e.g. firm size and firm age) on adoption. Additionally, overall information on the impact of market trends, facilitating conditions, institutional pressures or supplier landscape is limited. While some articles discuss the effect of external pressures on the adopting entity ( Oettmeier and Hofmann, 2017 ; Schniederjans, 2017 ; Tsai and Yeh, 2019 ), no differentiation is made among its source of emission. According to IT, pressure is exerted from competitors, trading partners and authorities. The impact of isomorphism on the adoption of radical manufacturing technology is well known in the contemporary literature (e.g. Kuan and Chau, 2001 ; Alshamaila et al. , 2013 ), however understudied in the field of 3DP.
We conclude that the lack of industry data is limiting both, the extent of quantitative research methods across the field of organizational 3DP adoption as well as the application of integral theoretical constructs. This results in a polarized set of findings, with vast knowledge being generated on the benefits and barriers of the technology for the organization, but relatively little information on the impact of a firm’s environment or contingency factors exert on the adoption decision was obtained. Findings of interest for the managerial audience are yet limited, as hardly any in-depth information on specific industries was generated so far, neither through survey nor case studies. Moreover, most existent results emphasized the intent of adoption rather than the experience of already adopters.
6. Opportunities for future research
The underlying work detected various untapped areas of research; opportunities for future investigation are highlighted in the following paragraphs.
6.1 Maturity of industry data
In-depth analysis of the organizational adoption of 3DP and underlying drivers requires more and specialized industry data, both from (1) specific industries as well as from (2) 3DP adopters. First, future research should emphasize on the action of adoption rather than the intent, and thus investigate adoption behavior among organizations that have already integrated 3DP into their manufacturing processes. This would not only support in validating past hypothesis but also induce more generalizable results on 3DP adoption. Second, as adoption behavior varies across industries, data from single industries, either quantitatively or qualitatively (industry case study) derived, would allow to analyze the impact of the industrial environment on the adoption decision. Results from single industries would constitute a useful tool for comparing 3DP diffusion and adoption characteristics across industries and moreover facilitate decision-making for future adopters.
6.2 Characteristics of adopters vs nonadopters
We recommend future studies to compare the characteristics of adopting and nonadopting organizations from an empirical perspective. The frequency analysis revealed that organizational readiness represents one of the most crucial drivers for adoption. A comparison among adopters and nonadopters would yield valuable insights into how to prepare firms for technology adoption. Also the experience of top management with the technology seems of utmost importance for successful organizational adoption. Here future research could investigate the impact of top management experience on 3DP usage by means of user-centered models (UTAUT and TAM).
6.3 Emphasis on the environmental context
The underlying research has placed little emphasis on the impact of firm-external factors on the organizational adoption decision. The environmental context represents only 12% of overall quotes in our content analysis. Hardly any research studied the impact that stakeholders have on organizational adoption behavior. Governmental and regulatory support, i.e. subsidies or educational initiatives or restrictions, i.e. environmental legislation, IP rights, received even less attention among literature studies. As such, profound analyses of the impact of institutional drivers (industry stakeholders: competitors, trading partners and government) on the adoption decision through IT would represent a fruitful area of research. Future studies might also conduct semistructured interviews with regulatory institutions to understand how contemporary regulation and legislation promotes or inhibits the diffusion of 3DP among industries.
6.4 Impact of contingency factors
While the impact of organizational characteristics, such as technology readiness and existence of skilled workforce, was frequently validated among existing research, contingency criteria were hardly investigated. Firm origin, size and age may be of utmost importance for technology adoption. Future research could aim to answer the question of whether firm age impacts the technology adoption decision, thus whether incumbent firms are more likely to adopt 3DP technology than new entrants. Furthermore, a macro study may be conducted to holistically analyze the phenomenon, applying TEO and integrating contingency factors as independent rather than moderator variables.
6.5 Metaanalysis on adoption drivers
While the content analysis revealed that the relative advantage offered by 3DP represents a significant adoption driver across almost all quantitative studies, other drivers appear to depend on the organizational and environmental context the firm is immersed into. Until today, no metaanalysis has yet been conducted to study the relevance of single adoption criteria across the quantitative studies on the organizational adoption of 3DP. Future research by means of metaanalysis could support the existing body of research in generalizing and validating existent findings.
7. Implications and limitations
This study thoroughly reviewed the existing body of research on the adoption of 3DP and generated valuable insights into both academia as well as management. Our findings support this growing scientific community in encountering untapped areas of research and channeling upcoming scientific projects. The bibliometric analysis of literature conveys an overview of the existing body of research, patterns and opportunities. Additionally, the critical analysis of contemporary adoption models, as well as their inherent characteristics, allows to better understand the interplay between results and theory, thus supporting future research in selecting the most appropriate adoption model. As such, the underlying review represents a valuable base of information, theory and sources for any empirical work on 3DP. Our results also offer relevant insights for practice, especially managerial decision-makers on the verge of technology adoption. The adoption drivers revealed in our study represent appropriate factors of consideration for manufacturing managers during the decision-making process. Furthermore, our results generate awareness on the effect 3DP adoption exerts on the different areas of an organization, thus supporting management in appraising the impact of technology integration in a holistic manner. Hence, the underlying work can serve as a guide for decision-making and as support in evaluating all possible implications of 3DP adoption in organizational environment.
Even though an ample range of literature was examined to develop the underlying review, and the screening criteria employed were developed in an inclusive way, there may still exist literature of interest that has not been included. Furthermore, it is paramount to acknowledge that due the nature of content analysis, the prominence of the adoption criteria is measured in terms of frequencies as opposed to their effective impact on the phenomenon under analysis. Thus, content analysis cannot serve to generalize the impact of particular drivers on the adoption of 3DP. To measure the impact, the obtained results would require to be quantitatively tested.
Publication selection strategy and final selection
Distribution of publications from 2010 to 2020
Distribution of publications across journals
Number of publications per year and per research method
Theoretical perspectives used in publications
Industries upon analysis (multiple industries per publication)
Adoption theories
3DP adoption drivers in organizations
Note(s) : * Relevant authors but not exclusive
Ajzen , I. ( 1985 ), From Intentions to Actions: A Theory of Planned Behavior. Action and ControlFrom Cognition to Behavior , Springer-Verlag , New York .
Alshamaila , Y. , Papagiannidis , S. and Li , F. ( 2013 ), “ Cloud computing adoption by SMEs in the north east of England: a multi-perspective framework ”, Journal of Enterprise Information Management , Vol. 26 , pp. 250 - 275 , doi: 10.1108/17410391311325225 .
Anastasiadou , C. and Vettese , S. ( 2019 ), “ From souvenirs to 3D printed souvenirs. Exploring the capabilities of additive manufacturing technologies in (re)-framing tourist souvenirs ”, Tourism Management , Vol. 71 , pp. 428 - 442 .
Attaran , M. ( 2017 ), “ The rise of 3-D printing: the advantages of additive manufacturing over traditional manufacturing ”, Business Horizons , Kelley School of Business, Indiana University , Vol. 60 No. 5 , pp. 677 - 688 , doi: 10.1016/j.bushor.2017.05.011 .
Candi , M. and Beltagui , A. ( 2019 ), “ Effective use of 3D printing in the innovation process ”, Technovation , Vols 80–81 , November 2017 , pp. 63 - 73 , doi: 10.1016/j.technovation.2018.05.002 .
Cao , D. , Li , H. and Wang , G. ( 2014 ), “ Impacts of isomorphic pressures on BIM adoption in construction projects ”, Journal of Construction Engineering and Management , Vol. 140 No. 12 , pp. 1 - 9 , doi: 10.1061/(ASCE)CO.1943-7862.0000903 .
Chatzoglou , P. and Michailidou , V. ( 2019 ), “ A survey on the 3D printing technology readiness to use ”, International Journal of Production Research , Vol. 57 No. 8 , pp. 2585 - 2599 , doi: 10.1080/00207543.2019.1572934 .
Chaudhuri , A. , Rogers , H. , Soberg , P. and Pawar , K. ( 2018 ), “ The role of service providers in 3D printing adoption ”, Industrial Management and Data Systems , Vol. 119 No. 6 , pp. 1189 - 1205 , doi: 10.1108/IMDS-08-2018-0339 .
Cohen , D. ( 2014 ), “ Fostering mainstream adoption of industrial 3D printing: understanding the benefits and promoting organizational readiness ”, 3D Printing and Additive Manufacturing , Vol. 1 No. 2 , pp. 62 - 69 , doi: 10.1089/3dp.2014.0007 .
Davis , F. ( 1985 ), A Technology Acceptance Model for Empirically Testing New End-User Information Systems : Theory and Results , Massachusetts Institute of Technology , available at: http://hdl.handle.net/1721.1/15192 .
DiMaggio , P. and Powell , W. ( 1983 ), “ The iron cage revisited: institutional isomorphism and collective rationality in organizational fields ”, American Sociological Review , Vol. 48 , pp. 147 - 160 , available at: http://10.0.9.3/2095101 .
Dwivedi , Y. , Rana , N. , Jeyaraj , A. , Clement , M. and Williams , M. ( 2019 ), “ Re-examining the unified theory of acceptance and use of technology (UTAUT): towards a revised theoretical model ”, Information Systems Frontiers , Information Systems Frontiers , Vol. 21 No. 3 , pp. 719 - 734 , doi: 10.1007/s10796-017-9774-y .
Eyers , D.R. and Potter , A.T. ( 2015 ), “ E-commerce channels for additive manufacturing: an exploratory study ”, Journal of Manufacturing Technology Management , Vol. 26 No. 3 , pp. 390 - 411 , doi: 10.1108/JMTM-08-2013-0102 .
Fink , A. ( 2014 ), Conducting Research Literature Reviews: From the Internet to Paper , [2014] , 4th ed. , SAGE , Thousand Oaks, CA , available at: https://search.library.wisc.edu/catalog/9910206565302121 .
Fontana , F. , Klahn , C. and Meboldt , M. ( 2019 ), “ Value-driven clustering of industrial additive manufacturing applications ”, Journal of Manufacturing Technology Management , Vol. 30 No. 2 , pp. 366 - 390 , doi: 10.1108/JMTM-06-2018-0167 .
Fox , S. ( 2014 ), “ Third wave do-it-yourself (DIY): potential for presumption, innovation, and entrepreneurship by local populations in regions without industrial manufacturing infrastructure ”, Technology in Society , Vol. 39 , pp. 18 - 30 .
Gartner ( 2019 ), “ Hype cycle ”, available at: https://www.gartner.com/en/documents/3947508/hype-cycle-for-3d-printing-2019 .
Gartner , J. , Maresch , D. and Fink , M. ( 2015 ), “ The potential of additive manufacturing for technology entrepreneurship: an integrative technology assessment ”, Creativity and Innovation Management , Vol. 24 No. 4 , pp. 585 - 600 .
Garza , J. ( 2016 ), Understanding the Adoption of Additive Manufacturing , Massachusetts Institute of Technology, available at: http://hdl.handle.net/1721.1/110892 .
Gupta , B.M. and Dhawan , S.M. ( 2018 ), “ Three dimensional (3D) printing: a scientometric assessment of global publications output during 2007-16 ”, DESIDOC Journal of Library and Information Technology , Vol. 38 No. 4 , pp. 238 - 245 , doi: 10.14429/djlit.38.4.12300 .
Hsu , P.-F. , Kraemer , K.L. and Dunkle , D. ( 2006 ), “ Determinants of E-business use in U.S. Firms ”, Routledge , International Journal of Electronic Commerce , Vol. 10 No. 4 , pp. 9 - 45 , doi: 10.2753/JEC1086-4415100401 .
Jirsák , P. and Brunet-Thornton , R. ( 2019 ), “ Perspectives of operational additive manufacturing case studies from the Czech aerospace industry ”, Journal of Eastern European and Central Asian Research , Vol. 6 No. 1 , pp. 179 - 180 , doi: 10.15549/jeecar.v6i1.273 .
Kianian , B. , Tavassoli , S. , Larsson , T. and Diegel , O. ( 2016 ), “ The adoption of additive manufacturing technology in Sweden ”, Elsevier B.V. , Procedia CIRP , Vol. 40 , pp. 7 - 12 , doi: 10.1016/j.procir.2016.01.036 .
Kuan , K. and Chau , P. ( 2001 ), “ A perception-based model for EDI adoption in small businesses using a technology-organization-environment framework ”, Information and Management , Vol. 38 No. 8 , pp. 507 - 521 , doi: 10.1016/S0378-7206(01)00073-8 .
Levy , Y. and Ellis , T. ( 2006 ), “ A systems approach to conduct an effective literature review in support of information systems research ”, Informing Science , Vol. 9 , pp. 181 - 211 , doi: 10.28945/479 .
Long , Y. , Pan , J. , Zhang , Q. and Hao , Y. ( 2017 ), “ 3D printing technology and its impact on Chinese manufacturing ”, International Journal of Production Research , Vol. 55 No. 5 , pp. 1488 - 1497 , doi: 10.1080/00207543.2017.1280196 .
Marak , Z. , Tiwari , A. and Tiwari , S. ( 2019 ), “ Adoption of 3D printing technology: an innovation diffusion theory perspective ”, International Journal of Innovation , Vol. 7 No. 1 , pp. 87 - 103 , doi: 10.5585/iji.v7i1.393 .
Martinsuo , M. and Luomaranta , T. ( 2018 ), “ Adopting additive manufacturing in SMEs: exploring the challenges and solutions ”, Journal of Manufacturing Technology Management , Vol. 29 No. 6 , pp. 937 - 957 , doi: 10.1108/JMTM-02-2018-0030 .
Mayring , P. ( 2000 ), Qualitative Inhaltsanalyse. Grundlagen und Techniken , Deutscher Studien Verlag , Weinheim .
Mellor , S. , Hao , L. and Zhang , D. ( 2014 ), “ Additive manufacturing: a framework for implementation ”, International Journal of Production Economics , Vol. 149 , pp. 194 - 201 , doi: 10.1016/j.ijpe.2013.07.008 .
Murmura , F. and Bravi , L. ( 2018 ), “ Additive manufacturing in the wood-furniture sector: sustainability of the technology, benefits and limitations of adoption ”, Journal of Manufacturing Technology Management , Vol. 29 No. 2 , pp. 350 - 371 , doi: 10.1108/JMTM-08-2017-0175 .
Neuendorf , K. ( 2017 ), The Content Analysis Guidebook , 2nd ed. , SAGE Publications , Los Angeles .
Niaki , M. and Nonino , F. ( 2017 ), “ Impact of additive manufacturing on business competitiveness: a multiple case study ”, Journal of Manufacturing Technology Management , Vol. 28 No. 1 , pp. 56 - 74 , doi: 10.1108/JMTM-01-2016-0001 .
Oettmeier , K. and Hofmann , E. ( 2017 ), “ Additive manufacturing technology adoption: an empirical analysis of general and supply chain-related determinants ”, Springer , Berlin Heidelberg , Journal of Business Economics , Vol. 87 No. 1 , pp. 97 - 124 , doi: 10.1007/s11573-016-0806-8 .
Oliveira , T. and Martins , M. ( 2011 ), “ Literature review of information technology adoption models at firm level ”, The Electronic Journal Information Systems Evaluation , Vol. 14 No. 1 , pp. 110 - 121 .
Rogers , E.M. ( 1983 ), Diffusion of Innovation , 3rd ed. , Free Press , New York .
Rousseau , D. , Manning , J. and Denyer , D. ( 2008 ), “ Evidence in management and organizational science: assembling the field's full weight of scientific knowledge through syntheses ”, The Academy of Management Annals , Vol. 2 No. 1 , pp. 475 - 515 , doi: 10.1080/19416520802211651 .
Rylands , B. , Böhme , T. , Gorkin , R. , Fan , J. and Birtchnell , T. ( 2016 ), “ The adoption process and impact of additive manufacturing on manufacturing systems ”, Journal of Manufacturing Technology Management , Vol. 27 No. 7 , pp. 969 - 989 , doi: 10.1108/JMTM-12-2015-0117 .
Sandström , C. ( 2015 ), “ Adopting 3D Printing for manufacturing – the case of the hearing aid industry ”, Adopting 3D Printing for Manufacturing – The Case of the Hearing Aid Industry , No. 262 , pp. 1 - 20 .
Sandström , C. ( 2016 ), “ The non-disruptive emergence of an ecosystem for 3D Printing - insights from the hearing aid industry's transition 1989-2008 ”, Elsevier , Technological Forecasting and Social Change , Vol. 102 , pp. 160 - 168 , doi: 10.1016/j.techfore.2015.09.006 .
Schniederjans , D. ( 2017 ), “ Adoption of 3D-printing technologies in manufacturing: a survey analysis ”, Elsevier , International Journal of Production Economics , Vol. 183 No. November 2016 , pp. 287 - 298 , doi: 10.1016/j.ijpe.2016.11.008 .
Schniederjans , D. and Yalcin , M. ( 2018 ), “ Perception of 3D-printing: analysis of manufacturing use and adoption ”, Rapid Prototyping Journal , Vol. 24 No. 3 , pp. 510 - 520 , doi: 10.1108/RPJ-04-2017-0056 .
Scott , R. ( 1995 ), Institutions and Organizations , S. Publications , Thousan Oaks , doi: 10.3917/mana.172.0136 .
Sculpteo ( 2019 ), “ The state of 3D printing ”, available at: https://info.sculpteo.com/the-state-of-3d-printing-report ( accessed: 5 January 2020 ).
Sheeran , P. and Webb , T. ( 2016 ), “ The intention–behavior gap ”, Social and Personality Psychology Compass , Vol. 10 No. 9 , pp. 503 - 518 , doi: 10.1111/spc3.12265 .
Snyder , H. ( 2019 ), “ Literature review as a research methodology: an overview and guidelines ”, Journal of Business Research , Vol. 104 , March , pp. 333 - 339 , doi: 10.1016/j.jbusres.2019.07.039 .
Soares Aguiar , A. and Palma-dos-Reos , A. ( 2008 ), “ Why do firms adopt E-procurement systems? Using logistic regression to empirically test a conceptual model ”, IEEE Transactions on Engineering Management , Vol. 55 No. 1 , pp. 120 - 133 .
Steenhuis , H.H. and Pretorius , L. ( 2016 ), “ Consumer additive manufacturing or 3D printing adoption: an exploratory study ”, Journal of Manufacturing Technology Management , Vol. 27 No. 7 , pp. 990 - 1012 , doi: 10.1108/JMTM-01-2016-0002 .
Steenhuis , H. , Fang , X. and Ulusemre , T. ( 2020 ), “ Global diffusion of innovation during the fourth industrial revolution: the case of additive manufacturing or 3D printing ”, International Journal of Innovation and Technology Management , Vol. 17 No. 1 , pp. 1 - 34 , doi: 10.1142/S0219877020500054 .
Teo , H.H. , Wei , K. and Benbasat , I. ( 2003 ), “ Predicting intention to adopt interorganizational linkages: an institutional perspective ”, MIS Quarter , Vol. 27 No. 1 , pp. 19 - 49 . available at: https://doi:10.2307/30036518 .
Tornatzky , L. and Fleischer , M. ( 1990 ), The Processes of Technological Innovation , Lexington Books , Lexington, MA .
Tranfield , D. , Denyer , D. and Smart , P. ( 2003 ), “ Towards a methodology for developing evidence-informed management knowledge by means of systematic review* introduction: the need for an evidence-informed approach ”, British Journal of Management , Vol. 14 , pp. 207 - 222 .
Tsai , C. and Yeh , C. ( 2019 ), “ Understanding the decision rules for 3D printing adoption ”, Technology Analysis and Strategic Management , Vol. 31 No. 9 , pp. 1104 - 1117 .
Venkatesh , V. and Davis , F. ( 2000 ), “ A theoretical extension of the technology acceptance model: four longitudinal field studies ”, Management Science , Vol. 46 No. 2 , pp. 186 - 204 .
Venkatesh , V. , Morris , M. , Davis , G. and Davis , F. ( 2003 ), “ User acceptance of information technology: toward a unified view ”, MIS Quarterly , Vol. 27 No. 3 , pp. 425 - 478 , available at: https://www.jstor.org/stable/30036540 .
Wang , Y. , Wang , Y. and Yang , Y. ( 2010 ), “ Understanding the determinants of RFID adoption in the manufacturing industry ”, Elsevier , Technological Forecasting and Social Change , Vol. 77 No. 5 , pp. 803 - 815 , doi: 10.1016/j.techfore.2010.03.006 .
Wang , Q. , Sun , X. , Cobb , S. , Lawson , G. and Sharples , S. ( 2016 ), “ 3D printing system: an innovation for small-scale manufacturing in home settings? – early adopters of 3D printing systems in China ”, International Journal of Production Research , Vol. 54 No. 20 , pp. 6017 - 6032 , doi: 10.1080/00207543.2016.1154211 .
Weber , R. ( 1990 ), Basic Content Analysis , 2nd ed. , SAGE Publications , California .
Weller , C. , Kleer , R. and Piller , F.T. ( 2015 ), “ Economic implications of 3D printing: market structure models in light of additive manufacturing revisited ”, International Journal of Production Economics , Elsevier , Vol. 164 , pp. 43 - 56 , doi: 10.1016/j.ijpe.2015.02.020 .
Westphal , M. ( 2018 ), The Promise of the Future: 3D Printing in Manufacturing , University of the Rockies, available at: https://0-search-proquest-com.biblioteca-ils.tec.mx/pqdtglobal/docview/2129666791/fulltextPDF/926FFF4E187C4A47PQ/1?accountid=11643 .
Wohlers Associates ( 2019 ), Wohlers Report 2019, 3D Printing and Additive Manufacturing State of the Industry, Annual Worldwide Progress Report , Wohler Associates , Fort Collins .
Wong , G. , Greenhalgh , T. , Westhorp , G. , Buckingham , J. and Pawson , R. ( 2013 ), “ RAMESES publication standards: meta-narrative reviews ”, Journal of Advanced Nursing , Vol. 69 No. 5 , pp. 987 - 1004 , doi: 10.1111/jan.12092 .
Yeh , C. and Chen , Y. ( 2018a ), “ Critical success factors for adoption of 3D printing ”, Technological Forecasting and Social Change , Vol. 132 , June 2017 , pp. 209 - 216 , doi: 10.1016/j.techfore.2018.02.003 .
Yeh , C. and Chen , Y. ( 2018b ), “ Technological forecasting and social change critical success factors for adoption of 3D printing ”, Technological Forecasting and Social Change , Vol. 132 , January , pp. 209 - 216 , doi: 10.1016/j.techfore.2018.02.003 .
Yoon , T. and George , J. ( 2013 ), “ Why aren't organizations adopting virtual worlds? ”, Computers in Human Behavior , Vol. 29 No. 3 , pp. 772 - 790 , doi: 10.1016/j.chb.2012.12.003 .
Further reading
Dwivedi , G. , Srivastava , S.K. and Srivastava , R.K. ( 2017 ), “ Analysis of barriers to implement additive manufacturing technology in the Indian automotive sector ”, International Journal of Physical Distribution and Logistics Management , Vol. 47 No. 10 , pp. 972 - 991 , doi: 10.1108/IJPDLM-07-2017-0222 .
Khorram Niaki , M. and Nonino , F. ( 2017 ), “ Additive manufacturing management: a review and future research agenda ”, International Journal of Production Research , Taylor & Francis , Vol. 55 No. 5 , pp. 1419 - 1439 , doi: 10.1080/00207543.2016.1229064 .
Kunovjanek , M. and Reiner , G. ( 2019 ), “ How will the diffusion of additive manufacturing impact the raw material supply chain process? ”, International Journal of Production Research , Taylor & Francis , Vol. 58 No. 5 , pp. 1 - 15 , doi: 10.1080/00207543.2019.1661537 .
Maresch , D. and Gartner , J. ( 2018 ), “ Make disruptive technological change happen - the case of additive manufacturing ”, Elsevier , (June 2017) , Technological Forecasting and Social Change , p. 119216 , doi: 10.1016/j.techfore.2018.02.009 .
Oettmeier , K. and Hofmann , E. ( 2016 ), “ Impact of additive manufacturing technology adoption on supply chain management processes and components ”, Journal of Manufacturing Technology Management , Vol. 27 No. 7 , pp. 944 - 968 , doi: 10.1108/JMTM-12-2015-0113 .
Corresponding author
Related articles, we’re listening — tell us what you think, something didn’t work….
Report bugs here
All feedback is valuable
Please share your general feedback
Join us on our journey
Platform update page.
Visit emeraldpublishing.com/platformupdate to discover the latest news and updates
Questions & More Information
Answers to the most commonly asked questions here
Advertisement
A comprehensive review on 3D printing advancements in polymer composites: technologies, materials, and applications
- Critical Review
- Published: 31 May 2022
- Volume 121 , pages 127–169, ( 2022 )
Cite this article
- Praveenkumara Jagadeesh 1 ,
- Madhu Puttegowda 2 ,
- Sanjay Mavinkere Rangappa ORCID: orcid.org/0000-0001-8745-9532 1 ,
- Karfidov Alexey 3 ,
- Sergey Gorbatyuk 3 ,
- Anish Khan 4 ,
- Mrityunjay Doddamani 5 &
- Suchart Siengchin 1
2858 Accesses
24 Citations
1 Altmetric
Explore all metrics
3D printing is a constantly expanding technology that represents one of the most exciting and disruptive production possibilities available today. This technology has gained global recognition and garnered considerable attention in recent years. However, technological breakthroughs, particularly in the field of material science, continue to be the focus of research, particularly in terms of future advancements. The 3D printing techniques are employed for the manufacturing of advanced multifunctional polymer composites due to their mass customization, freedom of design, capability to print complex 3D structures, and rapid prototyping. The advantages of 3D printing with multipurpose materials enable solutions in challenging locations such as outer space and extreme weather conditions where human involvement is not possible. Each year, numerous research papers are published on the subject of imbuing composites with various capabilities such as magnetic, sensing, thermal, embedded circuitry, self-healing, and conductive qualities by the use of innovative materials and printing technologies. This review article discusses the various 3D printing techniques used in the manufacture of polymer composites, the various types of reinforced polymer composites (fibers, nanomaterials, and particles reinforcements), the characterization of 3D printed parts, and their applications in a various industries. Additionally, this review discussed the limitations of 3D printing processes, which may assist future researchers in increasing the utility of their works and overcoming the shortcomings of previous works. Additionally, this paper discusses processing difficulties, anisotropic behavior, stimuli-responsive characteristics (shape memory and self-healing materials), CAD constraints, layer-by-layer appearance, and void formation in printed composites. Eventually, the promise of maturing technology is discussed, along with recommendations for research activities that are desperately required to realize the immense potential of operational 3D printing.
This is a preview of subscription content, log in via an institution to check access.
Access this article
Price includes VAT (Russian Federation)
Instant access to the full article PDF.
Rent this article via DeepDyve
Institutional subscriptions
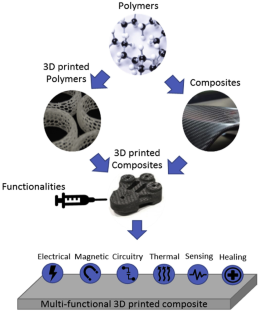
Similar content being viewed by others
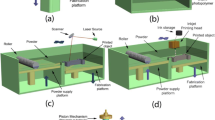
A review on 3D printed matrix polymer composites: its potential and future challenges
Processing, Applications, and Challenges of 3D Printed Polymer Nanocomposites
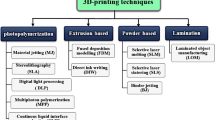
Particle-Reinforced Polymer Matrix Composites (PMC) Fabricated by 3D Printing
Availability of data and material.
Not applicable.
Code availability
Schubert C, Van Langeveld MC, Donoso LA (2014) Innovations in 3D printing: a 3D overview from optics to organs. Br J Ophthalmol 98:159–161. https://doi.org/10.1136/bjophthalmol-2013-304446
Article Google Scholar
Jiménez M, Romero L, Domínguez IA, Espinosa MDM, Dominguez M (2019) Additive manufacturing technologies: an overview about 3D printing methods and future prospects. Complexity. https://doi.org/10.1155/2019/9656938
Dawood A, Marti BM, Sauret-Jackson V, Darwood A (2015) 3D printing in dentistry. Br Dent J 219:521–529. https://doi.org/10.1038/sj.bdj.2015.914
Gopinathan J, Noh I (2018) Recent trends in bioinks for 3D printing. Biomater Res 22–11. https://doi.org/10.1186/s40824-018-0122-1
Oropallo W, Piegl LA (2016) Ten challenges in 3D printing. Eng Comput 32:135–148. https://doi.org/10.1007/s00366-015-0407-0
Chia HN, Wu BM (2015) Recent advances in 3D printing of biomaterials. J Biol Eng 9:1–14. https://doi.org/10.1186/s13036-015-0001-4
Lee JY, An J, Chua CK (2017) Fundamentals and applications of 3D printing for novel materials. Appl Mater Today 7:120–133. https://doi.org/10.1016/j.apmt.2017.02.004
Shahrubudin N, Lee TC, Ramlan R (2019) An overview on 3D printing technology: technological, materials, and applications. Procedia Manuf 35:1286–1296. https://doi.org/10.1016/j.promfg.2019.06.089
MacDonald E, Wicker R (2016) Multiprocess 3D printing for increasing component functionality. Science 80:353. https://doi.org/10.1126/science.aaf2093
Chen Z, Li Z, Li J, Liu C, Lao C, Fu Y, He Y (2019) 3D printing of ceramics: a review. J Eur Ceram Soc 39:661–687. https://doi.org/10.1016/j.jeurceramsoc.2018.11.013
Gross BC, Erkal JL, Lockwood SY, Chen C, Spence DM (2014) Evaluation of 3D printing and its potential impact on biotechnology and the chemical sciences. Anal Chem 86:3240–3253. https://doi.org/10.1021/ac403397r
Liu Z, Zhang M, Bhandari B, Wang Y (2017) 3D printing: printing precision and application in food sector. Trends Food Sci Technol 69:83–94. https://doi.org/10.1016/j.tifs.2017.08.018
Ambrosi A, Pumera M (2016) 3D-printing technologies for electrochemical applications. Chem Soc Rev 45:2740–2755. https://doi.org/10.1039/c5cs00714c
Ford S, Minshall T (2019) Invited review article: where and how 3D printing is used in teaching and education. Addit Manuf 25:131–150. https://doi.org/10.1016/j.addma.2018.10.028
Yan Q, Dong H, Su J, Han J, Song B, Wei Q, Shi Y (2018) A review of 3D printing technology for medical applications. Engineering 4:729–742. https://doi.org/10.1016/j.eng.2018.07.021
Bose S, Vahabzadeh S, Bandyopadhyay A (2013) Bone tissue engineering using 3D printing. Mater Today 16:496–504. https://doi.org/10.1016/j.mattod.2013.11.017
Bourell D, Kruth JP, Leu M, Levy G, Rosen D, Beese AM, Clare A (2017) Materials for additive manufacturing. CIRP Ann - Manuf Technol 66:659–681. https://doi.org/10.1016/j.cirp.2017.05.009
Tengsuthiwat J, Sanjay MR, Siengchin S, Pruncu CI (2020) 3D-MID technology for surface modification of polymer-based composites: a comprehensive review. Polymers 12:1408. https://doi.org/10.3390/polym12061408
Aimar A, Palermo A, Innocenti B (2019) The role of 3D printing in medical applications: a state of the art. J Healthc Eng 2019(5340616):10
Google Scholar
Chimene D, Lennox KK, Kaunas RR, Gaharwar AK (2016) Advanced bioinks for 3D printing: a materials science perspective. Ann Biomed Eng 44:2090–2102. https://doi.org/10.1007/s10439-016-1638-y
Compton BG, Lewis JA (2014) 3D-printing of lightweight cellular composites. Adv Mater 26:5930–5935. https://doi.org/10.1002/adma.201401804
Giannopoulos AA, Mitsouras D, Yoo SJ, Liu PP, Chatzizisis YS, Rybicki FJ (2016) Applications of 3D printing in cardiovascular diseases. Nat Rev Cardiol 13:701–718. https://doi.org/10.1038/nrcardio.2016.170
Tack P, Victor J, Gemmel P, Annemans L (2016) 3D-printing techniques in a medical setting: a systematic literature review. Biomed Eng Online 15:1–21. https://doi.org/10.1186/s12938-016-0236-4
Valot L, Martinez J, Mehdi A, Subra G (2019) Chemical insights into bioinks for 3D printing. Chem Soc Rev 48:4049–4086. https://doi.org/10.1039/c7cs00718c
Tümer EH, Erbil HY (2021) Extrusion-based 3d printing applications of pla composites: A review. Coatings 11:1–42. https://doi.org/10.3390/coatings11040390
Bekas DG, Hou Y, Liu Y, Panesar A (2019) 3D printing to enable multifunctionality in polymer-based composites: a review. Compos Part B Eng 179:107540. https://doi.org/10.1016/j.compositesb.2019.107540
Ngo TD, Kashani A, Imbalzano G, Nguyen KT, Hui D (2018) Additive manufacturing (3D printing): a review of materials, methods, applications and challenges. Compos Part B Eng 143:172–196. https://doi.org/10.1016/j.compositesb.2018.02.012
Skylar-Scott MA, Mueller J, Visser CW, Lewis JA (2019) Voxelated soft matter via multimaterial multinozzle 3D printing. Nature 575:330–335. https://doi.org/10.1038/s41586-019-1736-8
Guvendiren M, Molde J, Soares RMD, Kohn J (2016) Designing biomaterials for 3D printing. ACS Biomater Sci Eng 2:1679–1693. https://doi.org/10.1021/acsbiomaterials.6b00121
Khoo ZX, Teoh JEM, Liu Y, Chua CK, Yang S, An J, Yeong WY (2015) 3D printing of smart materials: a review on recent progresses in 4D printing. Virtual Phys Prototyp 10:103–122. https://doi.org/10.1080/17452759.2015.1097054
Gebler M, Schoot Uiterkamp AJM, Visser C (2014) A global sustainability perspective on 3D printing technologies. Energy Policy 74:158–167. https://doi.org/10.1016/j.enpol.2014.08.033
AbouHashem Y, Dayal M, Savanah S, Štrkalj G (2015) The application of 3D printing in anatomy education. Med Educ Online 20:1–4. https://doi.org/10.3402/meo.v20.29847
Martin JH, Yahata BD, Hundley JM, Mayer JA, Schaedler TA, Pollock TM (2017) 3D printing of high-strength aluminium alloys. Nature 549:365–369. https://doi.org/10.1038/nature23894
Yuk H, Lu B, Lin S, Qu K, Xu J, Luo J, Zhao X (2020) 3D printing of conducting polymers. Nat Commun 11:4–11. https://doi.org/10.1038/s41467-020-15316-7
Sun J, Zhou W, Huang D, Fuh JY, Hong GS (2015) An overview of 3D printing technologies for food fabrication. Food Bioprocess Technol 8:1605–1615. https://doi.org/10.1007/s11947-015-1528-6
Hamilton CA, Alici G, in het Panhuis M (2018) 3D printing vegemite and marmite: redefining breadboards. J Food Eng 220:83–88. https://doi.org/10.1016/j.jfoodeng.2017.01.008
Lei D, Yang Y, Liu Z, Chen S, Song B, Shen A, You Z (2019) A general strategy of 3D printing thermosets for diverse applications. Mater Horizons 6:394–404. https://doi.org/10.1039/c8mh00937f
Seyednejad H, Gawlitta D, Kuiper RV, de Bruin A, van Nostrum CF, Vermonden T, Hennink WE (2012) In vivo biocompatibility and biodegradation of 3D-printed porous scaffolds based on a hydroxyl-functionalized poly(ε-caprolactone). Biomaterials 33:4309–4318. https://doi.org/10.1016/j.biomaterials.2012.03.002
Horn TJ, Harrysson OLA (2012) Overview of current additive manufacturing technologies and selected applications. Sci Prog 95:255–282. https://doi.org/10.3184/003685012X13420984463047
Wegst UGK, Bai H, Saiz E (2015) Bioinspired structural materials. Nat Mater 14:23–36. https://doi.org/10.1038/nmat4089
Zhu C, Han TYJ, Duoss EB, Golobic AM, Kuntz JD, Spadaccini CM, Worsley MA (2015) Highly compressible 3D periodic graphene aerogel microlattices. Nat Commun 6:1–8. https://doi.org/10.1038/ncomms7962
Schniederjans DG (2017) Adoption of 3D-printing technologies in manufacturing: a survey analysis. Int J Prod Econ 183:287–298. https://doi.org/10.1016/j.ijpe.2016.11.008
Godoi FC, Prakash S, Bhandari BR (2016) 3d printing technologies applied for food design: status and prospects. J Food Eng 179:44–54. https://doi.org/10.1016/j.jfoodeng.2016.01.025
Zhou LY, Fu J, He Y (2020) A review of 3D printing technologies for soft polymer materials. Adv Funct Mater 30:1–38. https://doi.org/10.1002/adfm.202000187
Karakurt I, Lin L (2020) 3D printing technologies: techniques, materials, and post-processing. Curr Opin Chem Eng 28:134–143. https://doi.org/10.1016/j.coche.2020.04.001
Nyberg EL, Farris AL, Hung BP, Dias M, Garcia JR, Dorafshar AH, Grayson WL (2017) 3D-printing technologies for craniofacial rehabilitation, reconstruction, and regeneration. Ann Biomed Eng 45:45–57. https://doi.org/10.1007/s10439-016-1668-5
Wang Y, Xu Z, Wu D, Bai J (2020) Current status and prospects of polymer powder 3D printing technologies. Materials (Basel). https://doi.org/10.3390/ma13102406
Kumar S (2003) Selective laser sintering: a qualitative and objective approach. Jom 55:43–47. https://doi.org/10.1007/s11837-003-0175-y
Fina F, Goyanes A, Gaisford S, Basit AW (2017) Selective laser sintering (SLS) 3D printing of medicines. Int J Pharm 529:285–293. https://doi.org/10.1016/j.ijpharm.2017.06.082
Praveenkumara J, HN VS, Madhu P, Gowda Y, Rangappa SM, Khan MR, Khan I, Siengchin S (2021) A comprehensive review on the effect of synthetic filler materials on fiber-reinforced hybrid polymer composites. J Text Inst 1(9). https://doi.org/10.1080/00405000.2021.1920151
Charoo NA, Barakh Ali SF, Mohamed EM, Kuttolamadom MA, Ozkan T, Khan MA, Rahman Z (2020) Selective laser sintering 3D printing–an overview of the technology and pharmaceutical applications. Drug Dev Ind Pharm 46:869–877. https://doi.org/10.1080/03639045.2020.1764027
Mokrane A, Boutaous M, Xin S (2018) Process of selective laser sintering of polymer powders: modeling, simulation, and validation. Comptes Rendus - Mec 346:1087–1103. https://doi.org/10.1016/j.crme.2018.08.002
Martinez PR, Goyanes A, Basit AW, Gaisford S (2017) Fabrication of drug-loaded hydrogels with stereolithographic 3D printing. Int J Pharm 532:313–317. https://doi.org/10.1016/j.ijpharm.2017.09.003
Mukhtarkhanov M, Perveen A, Talamona D (2020) Application of stereolithography based 3D printing technology in investment casting. Micromachines. https://doi.org/10.3390/mi11100946
Hull C (2012) On stereolithography Virtual Phys Prototyp 7:177. https://doi.org/10.1080/17452759.2012.723409
Manapat JZ, Chen Q, Ye P, Advincula RC (2017) 3D printing of polymer nanocomposites via stereolithography. Macromol Mater Eng 302:1–13. https://doi.org/10.1002/mame.201600553
Ge Q, Li Z, Wang Z, Kowsari K, Zhang W, He X, Zhou J, Fang NX (2020) Projection micro stereolithography based 3D printing and its applications. Int J Extrem Manuf 2:0–13
Lee MP, Cooper GJT, Hinkley T, Gibson GM, Padgett MJ, Cronin L (2015) Development of a 3D printer using scanning projection stereolithography. Sci Rep. https://doi.org/10.1038/srep09875
Mazzanti V, Malagutti L, Mollica F (2019) FDM 3D printing of polymers containing natural fillers: a review of their mechanical properties. Polymers (Basel). https://doi.org/10.3390/polym11071094
Wasti S, Adhikari S (2020) Use of biomaterials for 3D printing by fused deposition modeling technique: a review. Front Chem 8:1–14. https://doi.org/10.3389/fchem.2020.00315
Melocchi A, Uboldi M, Maroni A, Foppoli A, Palugan L, Zema L, Gazzaniga A (2020) 3D printing by fused deposition modeling of single- and multi-compartment hollow systems for oral delivery – a review. Int J Pharm 579:119155. https://doi.org/10.1016/j.ijpharm.2020.119155
Nguyen VH, Huynh TN, Nguyen TP, Tran TT (2020) Single and multi-objective optimisation of processing parameters for fused deposition modelling in 3D printing technology. Int J Automot Mech Eng 17:7542–7551. https://doi.org/10.15282/IJAME.17.1.2020.03.0558
Das SC, Ranganathan R, Murugan N (2018) Effect of build orientation on the strength and cost of PolyJet 3D printed parts. Rapid Prototyp J 24:832–839. https://doi.org/10.1108/RPJ-08-2016-0137
Tee YL, Peng C, Pille P, Leary M, Tran P (2020) PolyJet 3D printing of composite materials: experimental and modelling approach. Jom 72:1105–1117. https://doi.org/10.1007/s11837-020-04014-w
Meisel NA, Elliott AM, Williams CB (2015) A procedure for creating actuated joints via embedding shape memory alloys in PolyJet 3D printing. J Intell Mater Syst Struct 26:1498–1512. https://doi.org/10.1177/1045389X14544144
Gokuldoss PK, Kolla S, Eckert J (2017) Additive manufacturing processes: selective laser melting, electron beam melting and binder jetting-selection guidelines. Materials (Basel). https://doi.org/10.3390/ma10060672
Baumers M, Tuck C, Wildman R, Ashcroft I, Hague R (2017) Shape complexity and process energy consumption in electron beam melting: a case of something for nothing in additive manufacturing? J Ind Ecol 21:S157–S167. https://doi.org/10.1111/jiec.12397
Billiet T, Vandenhaute M, Schelfhout J, Van Vlierberghe S, Dubruel P (2012) A review of trends and limitations in hydrogel-rapid prototyping for tissue engineering. Biomaterials 33:6020–6041. https://doi.org/10.1016/j.biomaterials.2012.04.050
Luo Y, Lode A, Wu C, Chang J, Gelinsky M (2015) Alginate/nanohydroxyapatite scaffolds with designed core/shell structures fabricated by 3D plotting and in situ mineralization for bone tissue engineering. ACS Appl Mater Interfaces 7:6541–6549. https://doi.org/10.1021/am508469h
Murphy SV, Atala A (2014) 3D bioprinting of tissues and organs. Nat Biotechnol 32:773–785. https://doi.org/10.1038/nbt.2958
Vaithilingam J, Simonelli M, Saleh E, Senin N, Wildman RD, Hague RJ, Tuck CJ (2017) Combined inkjet printing and infrared sintering of silver nanoparticles using a swathe-by-swathe and layer-by-layer approach for 3-dimensional structures. ACS Appl Mater Interfaces 9:6560–6570. https://doi.org/10.1021/acsami.6b14787
Saleh E, Zhang F, He Y, Vaithilingam J, Fernandez JL, Wildman R, Tuck C (2017) 3D inkjet printing of electronics using UV conversion. Adv Mater Technol 2:1–8. https://doi.org/10.1002/admt.201700134
Cooperstein I, Layani M, Magdassi S (2015) 3D printing of porous structures by UV-curable O/W emulsion for fabrication of conductive objects. J Mater Chem C 3:2040–2044. https://doi.org/10.1039/c4tc02215g
Rosenthal M, Henneberger C, Gutkes A, Bues CT (2018) Liquid deposition modeling: a promising approach for 3D printing of wood. Eur J Wood Prod 76:797–799. https://doi.org/10.1007/s00107-017-1274-8
Saari M, Cox B, Richer E, Krueger PS, Cohen AL (2015) Fiber encapsulation additive manufacturing: an enabling technology for 3D printing of electromechanical devices and robotic components. 3D Print Addit Manuf 2:32–39. https://doi.org/10.1089/3dp.2015.0003
Leong KF, Liu D, Chua CK (2014) Tissue engineering applications of additive manufacturing. In: Hashmi S, Batalha GF, Van Tyne CJ, Yilbas B. (eds) Comprehensive Materials Processing, Elsevier, 251-264, ISBN 9780080965338. https://doi.org/10.1016/B978-0-08-096532-1.01010-4
Jagadeesh P, Ningappa VSH, Puttegowda M, Girijappa YGT, Rangappa SM, Khan MR, Khan I, Siengchin S (2021) Pongamia pinnata shell powder filled sisal/kevlar hybrid composites: physicomechanical and morphological characteristics. Polym Compos 42:4434–4447. https://doi.org/10.1002/pc.26160
Jaisingh Sheoran A, Kumar H (2020) Fused Deposition modeling process parameters optimization and effect on mechanical properties and part quality: Review and reflection on present research. Mater Today Proc 21:1659–1672. https://doi.org/10.1016/j.matpr.2019.11.296
Gay P, Blanco D, Pelayo F, Noriega A, Fernández P (2015) Analysis of factors influencing the mechanical properties of flat PolyJet manufactured parts. Procedia Eng 132:70–77. https://doi.org/10.1016/j.proeng.2015.12.481
Kadry H, Wadnap S, Xu C, Ahsan F (2019) Digital light processing (DLP)3D-printing technology and photoreactive polymers in fabrication of modified-release tablets. Eur J Pharm Sci 135:60–67. https://doi.org/10.1016/j.ejps.2019.05.008
Arefin AME, Khatri NR, Kulkarni N, Egan PF (2021) Polymer 3D printing review: materials, process, and design strategies for medical applications. Polymers (Basel) 13:1–24. https://doi.org/10.3390/polym13091499
Guo N, Leu MC (2013) Additive manufacturing: technology, applications and research needs. Front Mech Eng 8:215–243. https://doi.org/10.1007/s11465-013-0248-8
Love LJ, Kunc V, Rios O, Duty CE, Elliott AM, Post BK, Blue CA (2014) The importance of carbon fiber to polymer additive manufacturing. J Mater Res 29:1893–1898. https://doi.org/10.1557/jmr.2014.212
Yang D, Zhang H, Wu J, McCarthy ED (2021) Fibre flow and void formation in 3D printing of short-fibre reinforced thermoplastic composites: an experimental benchmark exercise. Addit Manuf 37:101686. https://doi.org/10.1016/j.addma.2020.101686
Cheng P, Wang K, Chen X, Wang J, Peng Y, Ahzi S, Chen C (2021) Interfacial and mechanical properties of continuous ramie fiber reinforced biocomposites fabricated by in-situ impregnated 3D printing. Ind Crops Prod 170:113760. https://doi.org/10.1016/j.indcrop.2021.113760
Long H, Wu Z, Dong Q, Shen Y, Zhou W, Luo Y, Dong X (2019) Mechanical and thermal properties of bamboo fiber reinforced polypropylene/polylactic acid composites for 3D printing. Polym Eng Sci 59:E247–E260. https://doi.org/10.1002/pen.25043
Gupta A, Hasanov S, Fidan I (2019) Processing and characterization of 3d-printed polymer matrix composites reinforced with discontinuous fibers. In: Solid Freeform Fabrication 2019: Proceedings of the 30th Annual International Solid Freeform Fabrication Symposium - An Additive Manufacturing Conference, SFF 2019. pp 1054–1066
Ming Y, Zhang S, Han W, Wang B, Duan Y, Xiao H (2020) Investigation on process parameters of 3D printed continuous carbon fiber-reinforced thermosetting epoxy composites. Addit Manuf 33:101184. https://doi.org/10.1016/j.addma.2020.101184
Blok LG, Longana ML, Yu H, Woods BKS (2018) An investigation into 3D printing of fibre reinforced thermoplastic composites. Addit Manuf 22:176–186. https://doi.org/10.1016/j.addma.2018.04.039
Tian X, Liu T, Yang C, Wang Q, Li D (2016) Interface and performance of 3D printed continuous carbon fiber reinforced PLA composites. Compos Part A Appl Sci Manuf 88:198–205. https://doi.org/10.1016/j.compositesa.2016.05.032
Rahman MA, Islam MZ, Gibbon L, Ulven CA, La Scala JJ (2021) 3D printing of continuous carbon fiber reinforced thermoset composites using UV curable resin. Polym Compos 42:5859–5868. https://doi.org/10.1002/pc.26266
Le Duigou A, Barbé A, Guillou E, Castro M (2019) 3D printing of continuous flax fibre reinforced biocomposites for structural applications. Mater Des 180:107884. https://doi.org/10.1016/j.matdes.2019.107884
Ivey M, Melenka GW, Carey JP, Ayranci C (2017) Characterizing short-fiber-reinforced composites produced using additive manufacturing. Adv Manuf Polym Compos Sci 3:81–91. https://doi.org/10.1080/20550340.2017.1341125
Mohammadizadeh M, Imeri A, Fidan I, Elkelany M (2019) 3D printed fiber reinforced polymer composites - structural analysis. Compos Part B Eng 175:107112. https://doi.org/10.1016/j.compositesb.2019.107112
Akasheh F, Aglan H (2019) Fracture toughness enhancement of carbon fiber–reinforced polymer composites utilizing additive manufacturing fabrication. J Elastomers Plast 51:698–711. https://doi.org/10.1177/0095244318817867
Invernizzi M, Natale G, Levi M, Turri S, Griffini G (2016) UV-assisted 3D printing of glass and carbon fiber-reinforced dual-cure polymer composites. Materials (Basel). https://doi.org/10.3390/MA9070583
Dong K, Liu L, Huang X, Xiao X (2020) 3D printing of continuous fiber reinforced diamond cellular structural composites and tensile properties. Compos Struct 250:112610. https://doi.org/10.1016/j.compstruct.2020.112610
Balla VK, Tadimeti JGD, Kate KH, Satyavolu J (2020) 3D printing of modified soybean hull fiber/polymer composites. Mater Chem Phys 254:123452. https://doi.org/10.1016/j.matchemphys.2020.123452
Prajapati AR, Dave HK, Raval HK (2021) Effect of fiber volume fraction on the impact strength of fiber reinforced polymer composites made by FDM process. Mater Today Proc 44:2102–2106. https://doi.org/10.1016/j.matpr.2020.12.262
Li N, Li Y, Liu S (2016) Rapid prototyping of continuous carbon fiber reinforced polylactic acid composites by 3D printing. J Mater Process Technol 238:218–225. https://doi.org/10.1016/j.jmatprotec.2016.07.025
Wang P, Zou B, Ding S, Huang C, Shi Z, Ma Y, Yao P (2020) Preparation of short CF/GF reinforced PEEK composite filaments and their comprehensive properties evaluation for FDM-3D printing. Compos Part B Eng 198:108175. https://doi.org/10.1016/j.compositesb.2020.108175
Dickson AN, Barry JN, McDonnell KA, Dowling DP (2017) Fabrication of continuous carbon, glass and Kevlar fibre reinforced polymer composites using additive manufacturing. Addit Manuf 16:146–152. https://doi.org/10.1016/j.addma.2017.06.004
Yavas D, Zhang Z, Liu Q, Wu D (2021) Interlaminar shear behavior of continuous and short carbon fiber reinforced polymer composites fabricated by additive manufacturing. Compos Part B Eng 204:108460. https://doi.org/10.1016/j.compositesb.2020.108460
Sang L, Han S, Li Z, Yang X, Hou W (2019) Development of short basalt fiber reinforced polylactide composites and their feasible evaluation for 3D printing applications. Compos Part B Eng 164:629–639. https://doi.org/10.1016/j.compositesb.2019.01.085
Mosleh N, Rezadoust AM, Dariushi S (2021) Determining process-window for manufacturing of continuous carbon fiber-reinforced composite using 3D-printing. Mater Manuf Process 36:409–418. https://doi.org/10.1080/10426914.2020.1843664
Torrado Perez AR, Roberson DA, Wicker RB (2014) Fracture surface analysis of 3D-printed tensile specimens of novel ABS-based materials. J Fail Anal Prev 14:343–353. https://doi.org/10.1007/s11668-014-9803-9
Hector Sandoval J, Wicker RB (2006) Functionalizing stereolithography resins: effects of dispersed multi-walled carbon nanotubes on physical properties. Rapid Prototyp J 12:292–303. https://doi.org/10.1108/13552540610707059
Palaganas JO, Palaganas NB, Ramos LJI, David CPC (2019) 3D printing of covalent functionalized graphene oxide nanocomposite via stereolithography. ACS Appl Mater Interfaces 11:46034–46043. https://doi.org/10.1021/acsami.9b12071
Fantino E, Chiappone A, Calignano F, Fontana M, Pirri F, Roppolo I (2016) In situ thermal generation of silver nanoparticles in 3D printed polymeric structures. Materials (Basel) 9:21–23. https://doi.org/10.3390/ma9070589
Wang Y, Lei M, Wei Q, Wang Y, Zhang J, Guo Y, Saroia J (2020) 3D printing biocompatible l-Arg/GNPs/PLA nanocomposites with enhanced mechanical property and thermal stability. J Mater Sci 55:5064–5078. https://doi.org/10.1007/s10853-020-04353-8
Spinelli G, Lamberti P, Tucci V, Kotsilkova R, Ivanov E, Menseidov D, Kuzhir P (2019) Nanocarbon/poly(lactic) acid for 3D printing: Effect of fillers content on electromagnetic and thermal properties. Materials (Basel). https://doi.org/10.3390/ma12152369
Nonato RC, Mei LHI, Bonse BC, Chinaglia EF, Morales AR (2019) Nanocomposites of PLA containing ZnO nanofibers made by solvent cast 3D printing: production and characterization. Eur Polym J 114:271–278. https://doi.org/10.1016/j.eurpolymj.2019.02.026
Prashantha K, Roger F (2017) Multifunctional properties of 3D printed poly(lactic acid)/graphene nanocomposites by fused deposition modeling. J Macromol Sci Part A Pure Appl Chem 54:24–29. https://doi.org/10.1080/10601325.2017.1250311
Tambrallimath V, Keshavamurthy R, Bavan SD, Patil AY, Khan Y, Badruddin IA, Kamangar S (2021) Mechanical properties of pc-abs-based graphene-reinforced polymer nanocomposites fabricated by fdm process. Polymers (Basel). https://doi.org/10.3390/polym13172951
Huang B, He H, Meng S, Jia Y (2019) Optimizing 3D printing performance of acrylonitrile-butadiene-styrene composites with cellulose nanocrystals/silica nanohybrids. Polym Int 68:1351–1360. https://doi.org/10.1002/pi.5824
Gnanasekaran K, Heijmans T, van Bennekom S, Woldhuis H, Wijnia S, De With G, Friedrich H (2017) 3D printing of CNT- and graphene-based conductive polymer nanocomposites by fused deposition modeling. Appl Mater Today 9:21–28. https://doi.org/10.1016/j.apmt.2017.04.003
Coppola B, Cappetti N, Di Maio L, Scarfato P, Incarnato L (2017) Layered silicate reinforced polylactic acid filaments for 3D printing of polymer nanocomposites. IEEE 3rd International Forum on Research and Technologies for Society and Industry 3–6. https://doi.org/10.1109/RTSI.2017.8065892
Postiglione G, Natale G, Griffini G, Levi M, Turri S (2015) Conductive 3D microstructures by direct 3D printing of polymer/carbon nanotube nanocomposites via liquid deposition modeling. Compos Part A Appl Sci Manuf 76:110–114. https://doi.org/10.1016/j.compositesa.2015.05.014
Chen Q, Mangadlao JD, Wallat J, De Leon A, Pokorski JK, Advincula RC (2017) 3D printing biocompatible polyurethane/poly(lactic acid)/graphene oxide nanocomposites: anisotropic properties. ACS Appl Mater Interfaces 9:4015–4023. https://doi.org/10.1021/acsami.6b11793
Wei H, Cauchy X, Navas IO, Abderrafai Y, Chizari K, Sundararaj U, Therriault D (2019) Direct 3D printing of hybrid nanofiber-based nanocomposites for highly conductive and shape memory applications. ACS Appl Mater Interfaces 11:24523–24532. https://doi.org/10.1021/acsami.9b04245
Viskadourakis Z, Perrakis G, Symeou E, Giapintzakis J, Kenanakis G (2019) Transport properties of 3D printed polymer nanocomposites for potential thermoelectric applications. Appl Phys A Mater Sci Process. https://doi.org/10.1007/s00339-019-2469-0
Garcia Rosales CA, Garcia Duarte MF, Kim H, Chavez L, Hodges D, Mandal P, Tseng TLB (2018) 3D printing of shape memory polymer (SMP)/carbon black (CB) nanocomposites with electro-responsive toughness enhancement. Mater Res Express 5:065704. https://doi.org/10.1088/2053-1591/aacd53
Sanatgar RH, Campagne C, Nierstrasz V (2017) Investigation of the adhesion properties of direct 3D printing of polymers and nanocomposites on textiles: effect of FDM printing process parameters. Appl Surf Sci 403:551–563. https://doi.org/10.1016/j.apsusc.2017.01.112
Weng Z, Wang J, Senthil T, Wu L (2016) Mechanical and thermal properties of ABS/montmorillonite nanocomposites for fused deposition modeling 3D printing. Mater Des 102:276–283. https://doi.org/10.1016/j.matdes.2016.04.045
Guo Y, Zuo X, Xue Y, Tang J, Gouzman M, Fang Y, Rafailovich MH (2020) Engineering thermally and electrically conductive biodegradable polymer nanocomposites. Compos Part B Eng 189:107905. https://doi.org/10.1016/j.compositesb.2020.107905
Isakov DV, Lei Q, Castles F, Stevens CJ, Grovenor CRM, Grant PS (2016) 3D printed anisotropic dielectric composite with meta-material features. Mater Des 93:423–430. https://doi.org/10.1016/j.matdes.2015.12.176
Shemelya CM, Rivera A, Perez AT, Rocha C, Liang MIN, Yu X, Roberson DA (2015) Mechanical, electromagnetic, and X-ray shielding characterization of a 3D printable tungsten–polycarbonate polymer matrix composite for space-based applications. J Electron Mater 44:2598–2607. https://doi.org/10.1007/s11664-015-3687-7
Chung H, Das S (2006) Processing and properties of glass bead particulate-filled functionally graded nylon-11 composites produced by selective laser sintering. Mater Sci Eng A 437:226–234. https://doi.org/10.1016/j.msea.2006.07.112
Kalsoom U, Peristyy A, Nesterenko PN, Paull B (2016) A 3D printable diamond polymer composite: a novel material for fabrication of low cost thermally conducting devices. RSC Adv 6:38140–38147. https://doi.org/10.1039/c6ra05261d
Kokkinis D, Schaffner M, Studart AR (2015) Multimaterial magnetically assisted 3D printing of composite materials. Nat Commun. https://doi.org/10.1038/ncomms9643
Aw YY, Yeoh CK, Idris MA, Teh PL, Elyne WN, Hamzah KA, Sazali SA (2018) Effect of printing parameters on tensile, dynamic mechanical, and thermoelectric properties of FDM 3D printed CABS/ZnO composites. Materials (Basel). https://doi.org/10.3390/ma11040466
Zheng H, Zhang J, Lu S, Wang G, Xu Z (2006) Effect of core-shell composite particles on the sintering behavior and properties of nano-Al2O3/polystyrene composite prepared by SLS. Mater Lett 60:1219–1223. https://doi.org/10.1016/j.matlet.2005.11.003
Shuai CJ, Mao ZZ, Han ZK, Peng SP (2014) Preparation of complex porous scaffolds via selective laser sintering of poly(vinyl alcohol)/calcium silicate. J Bioact Compat Polym 29:110–120. https://doi.org/10.1177/0883911514522570
Zgalat-Lozynskyy OB, Matviichuk OO, Tolochyn OI, Ievdokymova OV, Zgalat-Lozynska NO, Zakiev VI (2021) Polymer materials reinforced with silicon nitride particles for 3D printing. Powder Metall Met Ceram 59:515–527. https://doi.org/10.1007/s11106-021-00189-2
Chueh YH, Wei C, Zhang X, Li L (2020) Integrated laser-based powder bed fusion and fused filament fabrication for three-dimensional printing of hybrid metal/polymer objects. Addit Manuf 31:100928. https://doi.org/10.1016/j.addma.2019.100928
Nabipour M, Akhoundi B (2021) An experimental study of FDM parameters effects on tensile strength, density, and production time of ABS/Cu composites. J Elastomers Plast 53:146–164. https://doi.org/10.1177/0095244320916838
Aqzna SS, Yeoh CK, Idris MS, Teh PL, bin Hamzah KA, Aw YY, Atiqah TN (2018) Effect of different filler content of ABS–zinc ferrite composites on mechanical, electrical and thermal conductivity by using 3D printing. J Vinyl Addit Technol 24:E217–E229. https://doi.org/10.1002/vnl.21640
Dizon JRC, Chen Q, Valino AD, Advincula RC (2019) Thermo-mechanical and swelling properties of three-dimensional-printed poly (ethylene glycol) diacrylate/silica nanocomposites. MRS Commun 9:209–217. https://doi.org/10.1557/mrc.2018.188
Liu W, Wu N, Pochiraju K (2018) Shape recovery characteristics of SiC/C/PLA composite filaments and 3D printed parts. Compos Part A Appl Sci Manuf 108:1–11. https://doi.org/10.1016/j.compositesa.2018.02.017
Quill TJ, Smith MK, Zhou T, Baioumy MGS, Berenguer JP, Cola BA, Bougher TL (2018) Thermal and mechanical properties of 3D printed boron nitride – ABS composites. Appl Compos Mater 25:1205–1217. https://doi.org/10.1007/s10443-017-9661-1
Tan JC, Low HY (2018) Embedded electrical tracks in 3D printed objects by fused filament fabrication of highly conductive composites. Addit Manuf 23:294–302. https://doi.org/10.1016/j.addma.2018.06.009
Palmero EM, Casaleiz D, de Vicente J, López-Vidal S, Ramiro E, Bollero A (2019) Composites based on metallic particles and tuned filling factor for 3D-printing by fused deposition modeling. Compos Part A Appl Sci Manuf 124:105497. https://doi.org/10.1016/j.compositesa.2019.105497
Hamzah KA, Yeoh CK, Noor MM, Teh PL, Aw YY, Sazali SA, Wan Ibrahim WMA (2022) Mechanical properties and thermal and electrical conductivity of 3D printed ABS-copper ferrite composites via 3D printing technique. J Thermoplast Compos Mater 35:3–16. https://doi.org/10.1177/0892705719869405
Yang F, Zeng J, Long H, Xiao J, Luo Y, Gu J, Dong X (2020) Micrometer copper-zinc alloy particles-reinforced wood plastic composites with high gloss and antibacterial properties for 3d printing. Polymers (Basel). https://doi.org/10.3390/polym12030621
Wu Y, Cao Y, Wu Y, Li D (2020) Mechanical properties and gamma-ray shielding performance of 3D-printed. Materials 13:4475. https://doi.org/10.3390/ma13204475
Liu M, Chiang SW, Chu X, Li J, Gan L, He Y, Du H (2020) Polymer composites with enhanced thermal conductivity via oriented boron nitride and alumina hybrid fillers assisted by 3-D printing. Ceram Int 46:20810–20818. https://doi.org/10.1016/j.ceramint.2020.05.096
Yang L, Wang L, Chen Y (2020) Solid-state shear milling method to prepare PA12/boron nitride thermal conductive composite powders and their selective laser sintering 3D-printing. J Appl Polym Sci 137:1–13. https://doi.org/10.1002/app.48766
Aw YY, Yeoh CK, Idris MA, Teh PL, Elyne WN, Hamzah KA, Sazali SA (2018) Influence of filler precoating and printing parameter on mechanical properties of 3D printed acrylonitrile butadiene styrene/zinc oxide composite. Polym Plast Technol Eng 58:1–13. https://doi.org/10.1080/03602559.2018.1455861
Dawoud M, Taha I, Ebeid SJ (2018) Strain sensing behaviour of 3D printed carbon black filled ABS. J Manuf Process 35:337–342. https://doi.org/10.1016/j.jmapro.2018.08.012
Castles F, Isakov D, Lui A, Lei Q, Dancer CEJ, Wang Y, Grant PS (2016) Microwave dielectric characterisation of 3D-printed BaTiO3/ABS polymer composites. Sci Rep 6:1–8. https://doi.org/10.1038/srep22714
Leigh SJ, Purssell CP, Bowen J, Hutchins DA, Covington JA, Billson DR (2011) A miniature flow sensor fabricated by micro-stereolithography employing a magnetite/acrylic nanocomposite resin. Sensors Actuators A Phys 168:66–71. https://doi.org/10.1016/j.sna.2011.03.058
Jabr RA (2005) Application of geometric programming to transformer design. IEEE Trans Magn 41:4261–4269. https://doi.org/10.1109/TMAG.2005.856921
Shokrollahi H, Janghorban K (2007) Influence of additives on the magnetic properties, microstructure and densification of Mn-Zn soft ferrites. Mater Sci Eng B Solid-State Mater Adv Technol 141:91–107. https://doi.org/10.1016/j.mseb.2007.06.005
Grau-Crespo R, Al-Baitai AY, Saadoune I, De Leeuw NH (2010) Vacancy ordering and electronic structure of γ-Fe2O 3 (maghemite): a theoretical investigation. J Phys Condens Matter. https://doi.org/10.1088/0953-8984/22/25/255401
Bollig LM, Hilpisch PJ, Mowry GS, Nelson-Cheeseman BB (2017) 3D printed magnetic polymer composite transformers. J Magn Magn Mater 442:97–101. https://doi.org/10.1016/j.jmmm.2017.06.070
Khatri B, Lappe K, Noetzel D, Pursche K, Hanemann T (2018) A 3D-printable polymer-metal soft-magnetic functional composite-development and characterization. Materials (Basel). https://doi.org/10.3390/ma11020189
Zhang J, Zhao S, Zhu M, Zhu Y, Zhang Y, Liu Z, Zhang C (2014) 3D-printed magnetic Fe3O4/MBG/PCL composite scaffolds with multifunctionality of bone regeneration, local anticancer drug delivery and hyperthermia. J Mater Chem B 2:7583–7595. https://doi.org/10.1039/c4tb01063a
Yue C, Li M, Liu Y, Fang Y, Song Y, Xu M, Li J (2021) Three-dimensional printing of cellulose nanofibers reinforced PHB/PCL/Fe3O4 magneto-responsive shape memory polymer composites with excellent mechanical properties. Addit Manuf 46:102146. https://doi.org/10.1016/j.addma.2021.102146
Schmitz DP, Ecco LG, Dul S, Pereira ECL, Soares BG, Barra GMO, Pegoretti A (2018) Electromagnetic interference shielding effectiveness of ABS carbon-based composites manufactured via fused deposition modelling. Mater Today Commun 15:70–80. https://doi.org/10.1016/j.mtcomm.2018.02.034
Khamis AM, Abbas Z, Azis RAS, Mensah EE, Alhaji IA (2021) Effects of recycled fe2o3 nanofiller on the structural, thermal, mechanical, dielectric, and magnetic properties of ptfe matrix. Polymers (Basel). https://doi.org/10.3390/polym13142332
Palmero EM, Rial J, de Vicente J, Camarero J, Skårman B, Vidarsson H, Bollero A (2018) Development of permanent magnet MnAlC/polymer composites and flexible filament for bonding and 3D-printing technologies. Sci Technol Adv Mater 19:465–473. https://doi.org/10.1080/14686996.2018.1471321
Ligon SC, Liska R, Stampfl J, Gurr M, Mülhaupt R (2017) Polymers for 3D printing and customized additive manufacturing. Chem Rev 117:10212–10290. https://doi.org/10.1021/acs.chemrev.7b00074
Jariwala D, Sangwan VK, Lauhon LJ, Marks TJ, Hersam MC (2013) Carbon nanomaterials for electronics, optoelectronics, photovoltaics, and sensing. Chem Soc Rev 42:2824–2860. https://doi.org/10.1039/c2cs35335k
Ghoshal S (2017) Polymer/carbon nanotubes (CNT) nanocomposites processing using additive manufacturing (three-dimensional printing) technique: an overview. Fibers. https://doi.org/10.3390/fib5040040
Last BJ, Thouless DJ (1971) Percolation theory and electrical conductivity. Phys Rev Lett 27:1719–1721. https://doi.org/10.1103/PhysRevLett.27.1719
De Leon AC, Chen Q, Palaganas NB, Palaganas JO, Manapat ARC (2016) High performance polymer nanocomposites for additive manufacturing applications. React Funct Polym 103:141–155. https://doi.org/10.1016/j.reactfunctpolym.2016.04.010
Wei X, Li D, Jiang W, Gu Z, Wang X, Zhang Z, Sun Z (2015) 3D printable graphene composite. Sci Rep 5:1–7. https://doi.org/10.1038/srep11181
Asp LE, Greenhalgh ES (2014) Structural power composites. Compos Sci Technol 101:41–61. https://doi.org/10.1016/j.compscitech.2014.06.020
Leng J, Lan X, Liu Y, Du S (2011) Shape-memory polymers and their composites: stimulus methods and applications. Prog Mater Sci 56:1077–1135. https://doi.org/10.1016/j.pmatsci.2011.03.001
He M, Zhao Y, Wang B, Xi Q, Zhou J, Liang Z (2015) 3D printing fabrication of amorphous thermoelectric materials with ultralow thermal conductivity. Small 11:5889–5894. https://doi.org/10.1002/smll.201502153
Chizari K, Arjmand M, Liu Z, Sundararaj U, Therriault D (2017) Three-dimensional printing of highly conductive polymer nanocomposites for EMI shielding applications. Mater Today Commun 11:112–118. https://doi.org/10.1016/j.mtcomm.2017.02.006
Sezer HK, Eren O (2019) FDM 3D printing of MWCNT re-inforced ABS nano-composite parts with enhanced mechanical and electrical properties. J Manuf Process 37:339–347. https://doi.org/10.1016/j.jmapro.2018.12.004
Rymansaib Z, Iravani P, Emslie E, Medvidović-Kosanović M, Sak-Bosnar M, Verdejo R, Marken F (2016) All-polystyrene 3D-printed electrochemical device with embedded carbon nanofiber-graphite-polystyrene composite conductor. Electroanalysis 28:1517–1523. https://doi.org/10.1002/elan.201600017
Bin Hamzah HH, Keattch O, Covill D, Patel BA (2018) The effects of printing orientation on the electrochemical behaviour of 3D printed acrylonitrile butadiene styrene (ABS)/carbon black electrodes. Sci Rep 8:1–8. https://doi.org/10.1038/s41598-018-27188-5
Ivanov E, Kotsilkova R, Xia H, Chen Y, Donato RK, Donato K, Angelov V (2019) PLA/Graphene/MWCNT composites with improved electrical and thermal properties suitable for FDM 3D printing applications. Appl Sci. https://doi.org/10.3390/app9061209
Sayyar S, Bjorninen M, Haimi S, Miettinen S, Gilmore K, Grijpma D, Wallace G (2016) UV cross-linkable graphene/poly(trimethylene carbonate) composites for 3D printing of electrically conductive scaffolds. ACS Appl Mater Interfaces 8:31916–31925. https://doi.org/10.1021/acsami.6b09962
Compton BG, Hmeidat NS, Pack RC, Heres MF, Sangoro JR (2018) Electrical and mechanical properties of 3D-printed graphene-reinforced epoxy. Jom 70:292–297. https://doi.org/10.1007/s11837-017-2707-x
Yuan S, Zheng Y, Chua CK, Yan Q, Zhou K (2018) Electrical and thermal conductivities of MWCNT/polymer composites fabricated by selective laser sintering. Compos Part A Appl Sci Manuf 105:203–213. https://doi.org/10.1016/j.compositesa.2017.11.007
Gojny FH, Wichmann MHG, Fiedler B, Kinloch IA, Bauhofer W, Windle AH, Schulte K (2006) Evaluation and identification of electrical and thermal conduction mechanisms in carbon nanotube/epoxy composites. Polymer (Guildf) 47:2036–2045. https://doi.org/10.1016/j.polymer.2006.01.029
Chowdhury RA, Hosur MV, Nuruddin M, Tcherbi-Narteh A, Kumar A, Boddu V, Jeelani S (2015) Self-healing epoxy composites: preparation, characterization and healing performance. J Mater Res Technol 4:33–43. https://doi.org/10.1016/j.jmrt.2014.10.016
Kim SY, Jones AR, Sottos NR, White SR (2017) Manufacturing of unidirectional glass/epoxy prepreg with microencapsulated liquid healing agents. Compos Sci Technol 153:190–197. https://doi.org/10.1016/j.compscitech.2017.10.017
Moll JL, Jin H, Mangun CL, White SR, Sottos NR (2013) Self-sealing of mechanical damage in a fully cured structural composite. Compos Sci Technol 79:15–20. https://doi.org/10.1016/j.compscitech.2013.02.006
Bekas DG, Baltzis D, Paipetis AS (2017) Nano-reinforced polymeric healing agents for vascular self-repairing composites. Mater Des 116:538–544. https://doi.org/10.1016/j.matdes.2016.12.049
Pang JWC, Bond IP (2005) A hollow fibre reinforced polymer composite encompassing self-healing and enhanced damage visibility. Compos Sci Technol 65:1791–1799. https://doi.org/10.1016/j.compscitech.2005.03.008
Kostopoulos V, Kotrotsos A, Tsantzalis S, Tsokanas P, Loutas T, Bosman AW (2016) Toughening and healing of continuous fibre reinforced composites by supramolecular polymers. Compos Sci Technol 128:84–93. https://doi.org/10.1016/j.compscitech.2016.03.021
Postiglione G, Alberini M, Leigh S, Levi M, Turri S (2017) Effect of 3D-printed microvascular network design on the self-healing behavior of cross-linked polymers. ACS Appl Mater Interfaces 9:14371–14378. https://doi.org/10.1021/acsami.7b01830
Aïssa B, Therriault D, Haddad E, Jamroz W (2012) Self-healing materials systems: overview of major approaches and recent developed technologies. Adv Mater Sci Eng. https://doi.org/10.1155/2012/854203
Nadgorny M, Xiao Z, Connal LA (2017) 2D and 3D-printing of self-healing gels: design and extrusion of self-rolling objects. Mol Syst Des Eng 2:283–292. https://doi.org/10.1039/c7me00023e
Almutairi MD, Aria AI, Thakur VK, Khan MA (2020) Self-healing mechanisms for 3D-printed polymeric structures: from lab to reality. Polymers (Basel) 12:1–27. https://doi.org/10.3390/polym12071534
Wu T, Gray E, Chen B (2018) A self-healing, adaptive and conductive polymer composite ink for 3D printing of gas sensors. J Mater Chem C 6:6200–6207. https://doi.org/10.1039/c8tc01092g
Sanders P, Young AJ, Qin Y, Fancey KS, Reithofer MR, Guillet-Nicolas R, Chin JM (2019) Stereolithographic 3D printing of extrinsically self-healing composites. Sci Rep 9:1–6. https://doi.org/10.1038/s41598-018-36828-9
Bi H, Ren Z, Ye G, Sun H, Guo R, Jia X, Xu M (2020) Fabrication of cellulose nanocrystal reinforced thermoplastic polyurethane/polycaprolactone blends for three-dimension printing self-healing nanocomposites. Cellulose 27:8011–8026. https://doi.org/10.1007/s10570-020-03328-x
Sanei SHR, Popescu D (2020) 3d-printed carbon fiber reinforced polymer composites: a systematic review. J Compos Sci. https://doi.org/10.3390/jcs4030098
Brenken B, Barocio E, Favaloro A, Kunc V, Pipes RB (2018) Fused filament fabrication of fiber-reinforced polymers: a review. Addit Manuf 21:1–16. https://doi.org/10.1016/j.addma.2018.01.002
Zhang J, Wang J, Dong S, Yu X, Han B (2019) A review of the current progress and application of 3D printed concrete. Compos Part A Appl Sci Manuf 125:105533. https://doi.org/10.1016/j.compositesa.2019.105533
Christiyan KGJ, Chandrasekhar U, Venkateswarlu K (2016) A study on the influence of process parameters on the mechanical properties of 3D printed ABS composite. IOP Conf Ser Mater Sci Eng. https://doi.org/10.1088/1757-899X/114/1/012109
Esposito Corcione C, Gervaso F, Scalera F, Padmanabhan SK, Madaghiele M, Montagna F, Maffezzoli A (2019) Highly loaded hydroxyapatite microsphere/ PLA porous scaffolds obtained by fused deposition modelling. Ceram Int 45:2803–2810. https://doi.org/10.1016/j.ceramint.2018.07.297
Mohammadizadeh M, Fidan I, Allen M, Imeri A (2018) Creep behavior analysis of additively manufactured fiber-reinforced components. Int J Adv Manuf Technol 99:1225–1234. https://doi.org/10.1007/s00170-018-2539-z
Liao G, Li Z, Cheng Y, Xu D, Zhu D, Jiang S, Zhu Y (2018) Properties of oriented carbon fiber/polyamide 12 composite parts fabricated by fused deposition modeling. Mater Des 139:283–292. https://doi.org/10.1016/j.matdes.2017.11.027
Sodeifian G, Ghaseminejad S, Yousefi AA (2019) Preparation of polypropylene/short glass fiber composite as fused deposition modeling (FDM) filament. Results Phys 12:205–222. https://doi.org/10.1016/j.rinp.2018.11.065
Dul S, Fambri L, Pegoretti A (2016) Fused deposition modelling with ABS-graphene nanocomposites. Compos Part A Appl Sci Manuf 85:181–191. https://doi.org/10.1016/j.compositesa.2016.03.013
Ding S, Zou B, Wang P, Ding H (2019) Effects of nozzle temperature and building orientation on mechanical properties and microstructure of PEEK and PEI printed by 3D-FDM. Polym Test 78:105948. https://doi.org/10.1016/j.polymertesting.2019.105948
Yu R, Yang X, Zhang Y, Zhao X, Wu X, Zhao T, Huang W (2017) Three-dimensional printing of shape memory composites with epoxy-acrylate hybrid photopolymer. ACS Appl Mater Interfaces 9:1820–1829. https://doi.org/10.1021/acsami.6b13531
Lewicki JP, Rodriguez JN, Zhu C, Worsley MA, Wu AS, Kanarska Y, King MJ (2017) 3D-printing of meso-structurally ordered carbon fiber/polymer composites with unprecedented orthotropic physical properties. Sci Rep 7:1–14. https://doi.org/10.1038/srep43401
Yu T, Zhang Z, Song S, Bai Y, Wu D (2019) Tensile and flexural behaviors of additively manufactured continuous carbon fiber-reinforced polymer composites. Compos Struct 225:111147. https://doi.org/10.1016/j.compstruct.2019.111147
Ning F, Cong W, Hu Y, Wang H (2017) Additive manufacturing of carbon fiber-reinforced plastic composites using fused deposition modeling: Effects of process parameters on tensile properties. J Compos Mater 51:451–462. https://doi.org/10.1177/0021998316646169
Tao Y, Wang H, Li Z, Li P, Shi SQ (2017) Development and application of wood flour-filled polylactic acid composite filament for 3d printing. Materials (Basel) 10:1–6. https://doi.org/10.3390/ma10040339
Ayrilmis N, Kariz M, Kwon JH, Kitek Kuzman M (2019) Effect of printing layer thickness on water absorption and mechanical properties of 3D-printed wood/PLA composite materials. Int J Adv Manuf Technol 102:2195–2200. https://doi.org/10.1007/s00170-019-03299-9
Hao W, Liu Y, Zhou H, Chen H, Fang D (2018) Preparation and characterization of 3D printed continuous carbon fiber reinforced thermosetting composites. Polym Test 65:29–34. https://doi.org/10.1016/j.polymertesting.2017.11.004
Kariz M, Sernek M, Obućina M, Kuzman MK (2018) Effect of wood content in FDM filament on properties of 3D printed parts. Mater Today Commun 14:135–140. https://doi.org/10.1016/j.mtcomm.2017.12.016
Caminero MÁ, Chacón JM, García-Plaza E, Núñez PJ, Reverte JM, Becar JP (2019) Additive manufacturing of PLA-based composites using fused filament fabrication: effect of graphene nanoplatelet reinforcement on mechanical properties, dimensional accuracy and texture. Polymers (Basel). https://doi.org/10.3390/polym11050799
Melenka GW, Cheung BKO, Schofield JS, Dawson MR, Carey JP (2016) Evaluation and prediction of the tensile properties of continuous fiber-reinforced 3D printed structures. Compos Struct 153:866–875. https://doi.org/10.1016/j.compstruct.2016.07.018
Skorski MR, Esenther JM, Ahmed Z, Miller AE, Hartings MR (2016) The chemical, mechanical, and physical properties of 3D printed materials composed of TiO2-ABS nanocomposites. Sci Technol Adv Mater 17:89–97. https://doi.org/10.1080/14686996.2016.1152879
Wang P, Zou B, Ding S, Lei LI, Huang C (2021) Effects of FDM-3D printing parameters on mechanical properties and microstructure of CF/PEEK and GF/PEEK. Chinese J Aeronaut 34:236–246. https://doi.org/10.1016/j.cja.2020.05.040
Zhu D, Ren Y, Liao G, Jiang S, Liu F, Guo J, Xu G (2017) Thermal and mechanical properties of polyamide 12/graphene nanoplatelets nanocomposites and parts fabricated by fused deposition modeling. J Appl Polym Sci 134:1–13. https://doi.org/10.1002/app.45332
Ferreira RTL, Amatte IC, Dutra TA, Bürger D (2017) Experimental characterization and micrography of 3D printed PLA and PLA reinforced with short carbon fibers. Compos Part B Eng 124:88–100. https://doi.org/10.1016/j.compositesb.2017.05.013
Goh GD, Dikshit V, Nagalingam AP, Goh GL, Agarwala S, Sing SL, Yeong WY (2018) Characterization of mechanical properties and fracture mode of additively manufactured carbon fiber and glass fiber reinforced thermoplastics. Mater Des 137:79–89. https://doi.org/10.1016/j.matdes.2017.10.021
Liu Z, Lei Q, Xing S (2019) Mechanical characteristics of wood, ceramic, metal and carbon fiber-based PLA composites fabricated by FDM. J Mater Res Technol 8:3743–3753. https://doi.org/10.1016/j.jmrt.2019.06.034
Jiang D, Smith DE (2017) Anisotropic mechanical properties of oriented carbon fiber filled polymer composites produced with fused filament fabrication. Addit Manuf 18:84–94. https://doi.org/10.1016/j.addma.2017.08.006
Spoerk M, Savandaiah C, Arbeiter F, Traxler G, Cardon L, Holzer C, Sapkota J (2018) Anisotropic properties of oriented short carbon fibre filled polypropylene parts fabricated by extrusion-based additive manufacturing. Compos Part A Appl Sci Manuf 113:95–104. https://doi.org/10.1016/j.compositesa.2018.06.018
Christ JF, Aliheidari N, Ameli A, Pötschke P (2017) 3D printed highly elastic strain sensors of multiwalled carbon nanotube/thermoplastic polyurethane nanocomposites. Mater Des 131:394–401. https://doi.org/10.1016/j.matdes.2017.06.011
Dul S, Fambri L, Pegoretti A (2018) Filaments production and fused deposition modelling of ABS/carbon nanotubes composites. Nanomaterials. https://doi.org/10.3390/nano8010049
Hymas DM, Arle MA, Singer F, Shooshtari AH, Ohadi MM (2017) Enhanced air-side heat transfer in an additively manufactured polymer composite heat exchanger. Proceedings of the 16th InterSociety Conference on Thermal and Thermomechanical Phenomena in Electronic Systems, ITherm 2017:634–638. https://doi.org/10.1109/ITHERM.2017.7992546
Wong M, Tsopanos S, Sutcliffe CJ, Owen I (2007) Selective laser melting of heat transfer devices. Rapid Prototyp J 13:291–297. https://doi.org/10.1108/13552540710824797
Arie MA, Shooshtari AH, Tiwari R, Dessiatoun SV, Ohadi MM, Pearce JM (2017) Experimental characterization of heat transfer in an additively manufactured polymer heat exchanger. Appl Therm Eng 113:575–584. https://doi.org/10.1016/j.applthermaleng.2016.11.030
Doganay D, Coskun S, Kaynak C, Unalan HE (2016) Electrical, mechanical and thermal properties of aligned silver nanowire/polylactide nanocomposite films. Compos Part B Eng 99:288–296. https://doi.org/10.1016/j.compositesb.2016.06.044
DI Stepashkin C, Senatov FS, Salimon AI, Korsunsky AM, Kaloshkin SD (2018) 3D-printed PEEK-carbon fiber (CF) composites: structure and thermal properties. Compos Sci Technol 164:319–326. https://doi.org/10.1016/j.compscitech.2018.05.032
Espera AH, Valino AD, Palaganas JO, Souza L, Chen Q, Advincula RC (2019) 3D printing of a robust polyamide-12-carbon black composite via selective laser sintering: thermal and electrical conductivity. Macromol Mater Eng 304:1–8. https://doi.org/10.1002/mame.201800718
Waheed S, Cabot JM, Smejkal P, Farajikhah S, Sayyar S, Innis PC, Paull B (2019) Three-dimensional printing of abrasive, hard, and thermally conductive synthetic microdiamond-polymer composite using low-cost fused deposition modeling printer. ACS Appl Mater Interfaces 11:4353–4363. https://doi.org/10.1021/acsami.8b18232
Guiney LM, Mansukhani ND, Jakus AE, Wallace SG, Shah RN, Hersam MC (2018) Three-dimensional printing of cytocompatible, thermally conductive hexagonal boron nitride nanocomposites. Nano Lett 18:3488–3493. https://doi.org/10.1021/acs.nanolett.8b00555
Nikzad M, Masood SH, Sbarski I (2011) Thermo-mechanical properties of a highly filled polymeric composites for fused deposition modeling. Mater Des 32:3448–3456. https://doi.org/10.1016/j.matdes.2011.01.056
Zhao Z, Teng K, Li N, Li X, Xu Z, Chen L, Liu Y (2017) Mechanical, thermal and interfacial performances of carbon fiber reinforced composites flavored by carbon nanotube in matrix/interface. Compos Struct 159:761–772. https://doi.org/10.1016/j.compstruct.2016.10.022
Vinyas M, Athul SJ, Harursampath D, Nguyen Thoi T (2019) Experimental evaluation of the mechanical and thermal properties of 3D printed PLA and its composites. Mater Res Express. https://doi.org/10.1088/2053-1591/ab43ab
Hassouna F, Raquez JM, Addiego F, Dubois P, Toniazzo V, Ruch D (2011) New approach on the development of plasticized polylactide (PLA): grafting of poly(ethylene glycol) (PEG) via reactive extrusion. Eur Polym J 47:2134–2144. https://doi.org/10.1016/j.eurpolymj.2011.08.001
MacDonald E, Salas R, Espalin D, Perez M, Aguilera E, Muse D, Wicker RB (2014) 3D printing for the rapid prototyping of structural electronics. IEEE Access 2:234–242. https://doi.org/10.1109/ACCESS.2014.2311810
Aslanzadeh S, Saghlatoon H, Honari MM, Mirzavand R, Montemagno C, Mousavi P (2018) Investigation on electrical and mechanical properties of 3D printed nylon 6 for RF/microwave electronics applications. Addit Manuf 21:69–75. https://doi.org/10.1016/j.addma.2018.02.016
Jakus AE, Secor EB, Rutz AL, Jordan SW, Hersam MC, Shah RN (2015) Three-dimensional printing of high-content graphene scaffolds for electronic and biomedical applications. ACS Nano 9:4636–4648. https://doi.org/10.1021/acsnano.5b01179
Leigh SJ, Bradley RJ, Purssell CP, Billson DR, Hutchins DA (2012) A simple, low-cost conductive composite material for 3D printing of electronic sensors. PLoS One 7:1–6. https://doi.org/10.1371/journal.pone.0049365
Farahani RD, Dalir H, Le Borgne V, Gautier LA, El Khakani MA, Therriault LMD (2012) Direct-write fabrication of freestanding nanocomposite strain sensors. Nanotechnology. https://doi.org/10.1088/0957-4484/23/8/085502
Tian X, Jin J, Yuan S, Chua CK, Tor SB, Zhou K (2017) Emerging 3D-printed electrochemical energy storage devices: a critical review. Adv Energy Mater 7:1–17. https://doi.org/10.1002/aenm.201700127
Flowers PF, Reyes C, Ye S, Kim MJ, Wiley BJ (2017) 3D printing electronic components and circuits with conductive thermoplastic filament. Addit Manuf 18:156–163. https://doi.org/10.1016/j.addma.2017.10.002
Kim H, Johnson J, Chavez LA, Rosales CAG, Tseng TLB, Lin Y (2018) Enhanced dielectric properties of three phase dielectric MWCNTs/BaTiO3/PVDF nanocomposites for energy storage using fused deposition modeling 3D printing. Ceram Int 44:9037–9044. https://doi.org/10.1016/j.ceramint.2018.02.107
Zang X, Shen C, Chu Y, Li B, Wei M, Zhong J, Lin L (2018) Laser-induced molybdenum carbide–graphene composites for 3D foldable paper electronics. Adv Mater 30:1–8. https://doi.org/10.1002/adma.201800062
Wang Z, Zhang Q, Long S, Luo Y, Yu P, Tan Z, Bai H (2018) Three-dimensional printing of polyaniline/reduced graphene oxide composite for high-performance planar supercapacitor. ACS Appl Mater Interfaces 10:10437–10444. https://doi.org/10.1021/acsami.7b19635
Han T, Kundu S, Nag A, Xu Y (2019) 3D printed sensors for biomedical applications: a review. Sensors (Switzerland). https://doi.org/10.3390/s19071706
Yoo DJ (2011) Computer-aided porous scaffold design for tissue engineering using triply periodic minimal surfaces. Int J Precis Eng Manuf 12:61–71. https://doi.org/10.1007/s12541-011-0008-9
Placone JK, Engler AJ (2018) Recent advances in extrusion-based 3D printing for biomedical applications. Adv Healthc Mater 7:1–11. https://doi.org/10.1002/adhm.201701161
Xu C, Dai G, Hong Y (2019) Recent advances in high-strength and elastic hydrogels for 3D printing in biomedical applications. Acta Biomater 95:50–59. https://doi.org/10.1016/j.actbio.2019.05.032
Alam F, Shukla VR, Varadarajan KM, Kumar S (2020) Microarchitected 3D printed polylactic acid (PLA) nanocomposite scaffolds for biomedical applications. J Mech Behav Biomed Mater 103:103576. https://doi.org/10.1016/j.jmbbm.2019.103576
Mondal S, Nguyen TP, Pham VH (2020) Hydroxyapatite nano bioceramics optimized 3D printed poly lactic acid scaffold for bone tissue engineering application. Ceram Int 46:3443–3455. https://doi.org/10.1016/j.ceramint.2019.10.057
Wang W, Caetano G, Ambler WS, Blaker JJ, Frade MA, Mandal P, Bártolo P (2016) Enhancing the hydrophilicity and cell attachment of 3D printed PCL/graphene scaffolds for bone tissue engineering. Materials (Basel). https://doi.org/10.3390/ma9120992
Bayer IS (2017) Thermomechanical properties of polylactic acid-graphene composites: a state-of-the-art review for biomedical applications. Materials (Basel). https://doi.org/10.3390/ma10070748
Rasoulianboroujeni M, Fahimipour F, Shah P, Khoshroo K, Tahriri M, Eslami H, Tayebi LJMS (2019) Development of 3D-printed PLGA/TiO 2 nanocomposite scaffolds for bone tissue engineering applications. Mater Sci Eng C 96:105–113. https://doi.org/10.1016/j.msec.2018.10.077
Chen X, Gao C, Jiang J, Wu Y, Zhu P, Chen G (2019) 3D printed porous PLA/nHA composite scaffolds with enhanced osteogenesis and osteoconductivity in vivo for bone regeneration. Biomed Mater 14. https://doi.org/10.1088/1748-605X/ab388d
Huang B, Vyas C, Roberts I, Poutrel QA, Chiang WH, Blaker JJ, Bártolo P (2019) Fabrication and characterisation of 3D printed MWCNT composite porous scaffolds for bone regeneration. Mater Sci Eng C 98:266–278. https://doi.org/10.1016/j.msec.2018.12.100
Manzoor F, Golbang A, Jindal S, Dixon D, McIlhagger A, Harkin-Jones E, Mancuso E (2021) 3D printed PEEK/HA composites for bone tissue engineering applications: effect of material formulation on mechanical performance and bioactive potential. J Mech Behav Biomed Mater 121:104601. https://doi.org/10.1016/j.jmbbm.2021.104601
Han X, Yang D, Yang C, Spintzyk S, Scheideler L, Li P, Rupp F (2019) Carbon fiber reinforced PEEK composites based on 3D-printing technology for orthopedic and dental applications. J Clin Med 8:1–17. https://doi.org/10.3390/jcm8020240
Ryan KR, Down MP, Banks CE (2021) Future of additive manufacturing: overview of 4D and 3D printed smart and advanced materials and their applications. Elsevier BV
Valino AD, Dizon JRC, Espera AH, Chen Q, Messman J, Advincula RC (2019) Advances in 3D printing of thermoplastic polymer composites and nanocomposites. Prog Polym Sci 98:101162. https://doi.org/10.1016/j.progpolymsci.2019.101162
Kalsoom U, Nesterenko PN, Paull B (2016) Recent developments in 3D printable composite materials. RSC Adv 6:60355–60371. https://doi.org/10.1039/c6ra11334f
Shemelya C, De La Rosa A, Torrado AR, Yu K, Domanowski J, Bonacuse PJ, Roberson DA (2017) Anisotropy of thermal conductivity in 3D printed polymer matrix composites for space based cube satellites. Addit Manuf 16:186–196. https://doi.org/10.1016/j.addma.2017.05.012
Wang X, Jiang M, Zhou Z, Gou J, Hui D (2017) 3D printing of polymer matrix composites: a review and prospective. Compos Part B Eng 110:442–458. https://doi.org/10.1016/j.compositesb.2016.11.034
Gibbons GJ, Williams R, Purnell P, Farahi E (2010) 3D Printing of cement composites. Adv Appl Ceram 109:287–290. https://doi.org/10.1179/174367509X12472364600878
Zareiyan B, Khoshnevis B (2017) Interlayer adhesion and strength of structures in contour crafting - effects of aggregate size, extrusion rate, and layer thickness. Autom Constr 81:112–121. https://doi.org/10.1016/j.autcon.2017.06.013
Zhang W, Melcher R, Travitzky N, Bordia RK, Greil P (2009) Three-dimensional printing of complex-shaped alumina/ glass composites. Adv Eng Mater 11:1039–1043. https://doi.org/10.1002/adem.200900213
Le Duigou A, Castro M, Bevan R, Martin N (2016) 3D printing of wood fibre biocomposites: from mechanical to actuation functionality. Mater Des 96:106–114. https://doi.org/10.1016/j.matdes.2016.02.018
Paul SC, Tay YWD, Panda B, Tan MJ (2018) Fresh and hardened properties of 3D printable cementitious materials for building and construction. Arch Civ Mech Eng 18:311–319. https://doi.org/10.1016/j.acme.2017.02.008
Minas C, Carnelli D, Tervoort E, Studart AR (2016) 3D printing of emulsions and foams into hierarchical porous ceramics. Adv Mater 28:9993–9999. https://doi.org/10.1002/adma.201603390
Zou R, Xia Y, Liu S, Hu P, Hou W, Hu Q, Shan C (2016) Isotropic and anisotropic elasticity and yielding of 3D printed material. Compos Part B Eng 99:506–513. https://doi.org/10.1016/j.compositesb.2016.06.009
Guessasma S, Belhabib S, Nouri H, Ben Hassana O (2016) Anisotropic damage inferred to 3D printed polymers using fused deposition modelling and subject to severe compression. Eur Polym J 85:324–340. https://doi.org/10.1016/j.eurpolymj.2016.10.030
Jagadeesh P, Thyavihalli Girijappa YG, Puttegowda M, Rangappa SM, Siengchin S (2020) Effect of natural filler materials on fiber reinforced hybrid polymer composites: an overview. J Nat Fibers 00:1–16. https://doi.org/10.1080/15440478.2020.1854145
Niendorf T, Leuders S, Riemer A, Richard HA, Tröster T, Schwarze D (2013) Highly anisotropic steel processed by selective laser melting. Metall Mater Trans B Process Metall Mater Process Sci 44:794–796. https://doi.org/10.1007/s11663-013-9875-z
Mühler T, Gomes CM, Heinrich J, Günster J (2015) Slurry-based additive manufacturing of ceramics. Int J Appl Ceram Technol 12:18–25. https://doi.org/10.1111/ijac.12113
Zocca A, Colombo P, Gomes CM, Günster J (2015) Additive manufacturing of ceramics: issues, potentialities, and opportunities. J Am Ceram Soc 98:1983–2001. https://doi.org/10.1111/jace.13700
He Z, Chen Y, Yang J, Tang C, Lv J, Liu Y, Hui D (2017) Fabrication of polydimethylsiloxane films with special surface wettability by 3D printing. Compos Part B Eng 129:58–65. https://doi.org/10.1016/j.compositesb.2017.07.025
Quan Z, Suhr J, Yu J, Qin X, Cotton C, Mirotznik M, Chou TW (2018) Printing direction dependence of mechanical behavior of additively manufactured 3D preforms and composites. Compos Struct 184:917–923. https://doi.org/10.1016/j.compstruct.2017.10.055
Coniglio N, Sivarupan T, El Mansori M (2018) Investigation of process parameter effect on anisotropic properties of 3D printed sand molds. Int J Adv Manuf Technol 94:2175–2185. https://doi.org/10.1007/s00170-017-0861-5
Yin S, Jacobsen AJ, Wu L, Nutt SR (2013) Inertial stabilization of flexible polymer micro-lattice materials. J Mater Sci 48:6558–6566. https://doi.org/10.1007/s10853-013-7452-0
Maskery I, Aboulkhair NT, Aremu AO, Tuck CJ, Ashcroft IA (2017) Compressive failure modes and energy absorption in additively manufactured double gyroid lattices. Addit Manuf 16:24–29. https://doi.org/10.1016/j.addma.2017.04.003
Jagadeesh P, Puttegowda M, Mavinkere Rangappa S, Siengchin S (2021) Influence of nanofillers on biodegradable composites: a comprehensive review. Polym Compos 42:5691–5711. https://doi.org/10.1002/pc.26291
Harris JA, Winter RE, McShane GJ (2017) Impact response of additively manufactured metallic hybrid lattice materials. Int J Impact Eng 104:177–191. https://doi.org/10.1016/j.ijimpeng.2017.02.007
Goyanes A, Det-Amornrat U, Wang J, Basit AW, Gaisford S (2016) 3D scanning and 3D printing as innovative technologies for fabricating personalized topical drug delivery systems. J Control Release 234:41–48. https://doi.org/10.1016/j.jconrel.2016.05.034
Ursan I, Chiu L, Pierce A (2013) Three-dimensional drug printing: a structured review. J Am Pharm Assoc 53:136–144. https://doi.org/10.1331/JAPhA.2013.12217
Hossainy S, Prabhu S (2008) A mathematical model for predicting drug release from a biodurable drug-eluting stent coating. J Biomed Mater Res Part A 87:487–493. https://doi.org/10.1002/jbm.a.31787
Campbell I, Bourell D, Gibson I (2012) Additive manufacturing: rapid prototyping comes of age. Rapid Prototyp J 18:255–258. https://doi.org/10.1108/13552541211231563
Sharma R, Singh R, Penna R, Fraternali F (2018) Investigations for mechanical properties of Hap, PVC and PP based 3D porous structures obtained through biocompatible FDM filaments. Compos Part B Eng 132:237–243. https://doi.org/10.1016/j.compositesb.2017.08.021
Burn MB, Ta A, Gogola GR (2016) Three-dimensional printing of prosthetic hands for children. J Hand Surg Am 41:e103–e109. https://doi.org/10.1016/j.jhsa.2016.02.008
Mannoor MS, Jiang Z, James T, Kong YL, Malatesta KA, Soboyejo WO, McAlpine MC (2013) 3D printed bionic ears. Nano Lett 13:2634–2639. https://doi.org/10.1021/nl4007744
Edgar J, Tint S (2015) Additive manufacturing technologies: 3D printing, rapid prototyping, and direct digital manufacturing. 2nd Edition. Johnson Matthey Technol Rev 59:193–198. https://doi.org/10.1595/205651315X688406
Turco E, Golaszewski M, Giorgio I, D’Annibale F (2017) Pantographic lattices with non-orthogonal fibres: experiments and their numerical simulations. Compos Part B Eng 118:1–14. https://doi.org/10.1016/j.compositesb.2017.02.039
Naddeo F, Cappetti N, Naddeo A (2017) Novel “load adaptive algorithm based” procedure for 3D printing of cancellous bone-inspired structures. Compos Part B Eng 115:60–69. https://doi.org/10.1016/j.compositesb.2016.10.033
Imbalzano G, Linforth S, Ngo TD, Lee PVS, Tran P (2018) Blast resistance of auxetic and honeycomb sandwich panels: comparisons and parametric designs. Compos Struct 183:242–261. https://doi.org/10.1016/j.compstruct.2017.03.018
Imbalzano G, Tran P, Ngo TD, Lee PVS (2017) Three-dimensional modelling of auxetic sandwich panels for localised impact resistance. J Sandw Struct Mater 19:291–316. https://doi.org/10.1177/1099636215618539
Ren X, Shen J, Tran P, Ngo TD, Xie YM (2018) Design and characterisation of a tuneable 3D buckling-induced auxetic metamaterial. Mater Des 139:336–342. https://doi.org/10.1016/j.matdes.2017.11.025
Parthasarathy J, Starly B, Raman S (2011) A design for the additive manufacture of functionally graded porous structures with tailored mechanical properties for biomedical applications. J Manuf Process 13:160–170. https://doi.org/10.1016/j.jmapro.2011.01.004
Bartlett NW, Tolley MT, Overvelde JTB, Weaver JC, Mosadegh B, Bertoldi K, Wood RJ (2015) Robot powered by combustion. Science 80(349):161–165. https://doi.org/10.1126/science.aab0129
Zarek M, Layani M, Cooperstein I, Sachyani E, Cohn D, Magdassi S (2016) 3D printing of shape memory polymers for flexible electronic devices. Adv Mater 28:4449–4454. https://doi.org/10.1002/adma.201503132
Mohamed OA, Masood SH, Bhowmik JL (2016) Optimization of fused deposition modeling process parameters for dimensional accuracy using I-optimality criterion. Meas J Int Meas Confed 81:174–196. https://doi.org/10.1016/j.measurement.2015.12.011
Parandoush P, Lin D (2017) A review on additive manufacturing of polymer-fiber composites. Compos Struct 182:36–53. https://doi.org/10.1016/j.compstruct.2017.08.088
Download references
This project was funded by King Mongkut’s University of Technology North Bangkok (KMUTNB), Grant No. KMUTNB-PHD-65–02.
Author information
Authors and affiliations.
Natural Composites Research Group Lab, Department of Materials and Production Engineering, The Sirindhorn International Thai-German Graduate School of Engineering (TGGS), King Mongkut’s University of Technology North Bangkok (KMUTNB), Bangkok, Thailand
Praveenkumara Jagadeesh, Sanjay Mavinkere Rangappa & Suchart Siengchin
Department of Mechanical Engineering, Malnad College of Engineering, Hassan, Visvesvaraya Technological University, Belagavi, Karnataka, India
Madhu Puttegowda
Department of Engineering of Technological Equipment, National University of Science and Technology MISIS, Moscow, Russia
Karfidov Alexey & Sergey Gorbatyuk
Chemistry Department, Faculty of Science, King Abdulaziz University, Jeddah, Saudi Arabia
Advanced Manufacturing Lab, Department of Mechanical Engineering, National Institute of Technology Karanataka (NITK), Surathkal, P.O. Srinivasanagar, D.K., Mangalore, 575 025, Karanataka, India
Mrityunjay Doddamani
You can also search for this author in PubMed Google Scholar
Contributions
All authors contributed equally.
Corresponding author
Correspondence to Sanjay Mavinkere Rangappa .
Ethics declarations
Ethics approval.
The authors hereby state that the present work is in compliance with the ethical standards.
Consent to participate
Consent for publication.
All authors have read and agreed to publish the manuscript.

Conflict of interest
The authors declare no competing interests.
Additional information
Publisher's note.
Springer Nature remains neutral with regard to jurisdictional claims in published maps and institutional affiliations.
Rights and permissions
Reprints and permissions
About this article
Jagadeesh, P., Puttegowda, M., Rangappa, S.M. et al. A comprehensive review on 3D printing advancements in polymer composites: technologies, materials, and applications. Int J Adv Manuf Technol 121 , 127–169 (2022). https://doi.org/10.1007/s00170-022-09406-7
Download citation
Received : 16 December 2021
Accepted : 22 May 2022
Published : 31 May 2022
Issue Date : July 2022
DOI : https://doi.org/10.1007/s00170-022-09406-7
Share this article
Anyone you share the following link with will be able to read this content:
Sorry, a shareable link is not currently available for this article.
Provided by the Springer Nature SharedIt content-sharing initiative
- 3D printing
- Technologies
- Polymer composites
- Additive manufacturing
- Multi-functionality
- Applications
- Find a journal
- Publish with us
- Track your research
A Survey of 3D Printing Technologies as Applied to Printed Electronics
Ieee account.
- Change Username/Password
- Update Address
Purchase Details
- Payment Options
- Order History
- View Purchased Documents
Profile Information
- Communications Preferences
- Profession and Education
- Technical Interests
- US & Canada: +1 800 678 4333
- Worldwide: +1 732 981 0060
- Contact & Support
- About IEEE Xplore
- Accessibility
- Terms of Use
- Nondiscrimination Policy
- Privacy & Opting Out of Cookies
A not-for-profit organization, IEEE is the world's largest technical professional organization dedicated to advancing technology for the benefit of humanity. © Copyright 2024 IEEE - All rights reserved. Use of this web site signifies your agreement to the terms and conditions.

An official website of the United States government
The .gov means it’s official. Federal government websites often end in .gov or .mil. Before sharing sensitive information, make sure you’re on a federal government site.
The site is secure. The https:// ensures that you are connecting to the official website and that any information you provide is encrypted and transmitted securely.
- Publications
- Account settings
Preview improvements coming to the PMC website in October 2024. Learn More or Try it out now .
- Advanced Search
- Journal List
- Int J Environ Res Public Health

Implementation of 3D Printing Technology in the Field of Prosthetics: Past, Present, and Future
Albert manero.
1 Limbitless Solutions, University of Central Florida, 4217 E Plaza Drive, Orlando, FL 32816, USA; [email protected] (P.S.); [email protected] (J.S.); gro.snoitulos-sseltibmil@DttaM (M.D.); gro.snoitulos-sseltibmil@euqinimod (D.C.); [email protected] (A.K.)
Peter Smith
John sparkman, matt dombrowski, dominique courbin, anna kester, isaac womack.
2 Division of Trauma, Critical Care & Acute Care Surgery Department of Surgery, Oregon Health and Science University, 3181 SW Sam Jackson Park Rd, Portland, OR 97239, USA; [email protected] (I.W.); ude.usho@aihc (A.C.)
There is an interesting and long history of prostheses designed for those with upper-limb difference, and yet issues still persist that have not yet been solved. Prosthesis needs for children are particularly complex, due in part to their growth rates. Access to a device can have a significant impact on a child’s psychosocial development. Often, devices supporting both cosmetic form and user function are not accessible to children due to high costs, insurance policies, medical availability, and their perceived durability and complexity of control. These challenges have encouraged a grassroots effort globally to offer a viable solution for the millions of people living with limb difference around the world. The innovative application of 3D printing for customizable and user-specific hardware has led to open-source Do It Yourself “DIY” production of assistive devices, having an incredible impact globally for families with little recourse. This paper examines new research and development of prostheses by the maker community and nonprofit organizations, as well as a novel case study exploring the development of technology and the training methods available. These design efforts are discussed further in the context of the medical regulatory framework in the United States and highlight new associated clinical studies designed to measure the quality of life impact of such devices.
1. Introduction
Prosthesis design can be dated back to the ancient Egyptian and Roman empires and has continued to develop across the world throughout the course of history [ 1 , 2 ]. In the late 1800s, John Hanger’s prosthesis, the Hanger Limb, was developed in response to the American Civil War [ 3 ], ushering prosthesis design into the modern era. Medical advancements since the invention of the Hanger Limb have significantly reduced limb loss due to traumatic events [ 4 , 5 ]. In 2005, an estimated 1.6 million people in the United States had a limb difference [ 6 ], and approximately 541,000 individuals had some level of upper-limb loss [ 6 ]. Based on current projections, this value may double by 2050 [ 6 ]. Trauma remains the most significant cause of upper-limb amputation, predominantly for males [ 7 ], though the subsection of dysvascular-driven adult amputations is rapidly growing. Global monitoring for congenital limb loss is reported annually by the International Clearinghouse for Birth Defects Surveillance and Research Annual Report [ 8 ]. It is reported that congenital and pediatric amputations account for a significant population [ 9 , 10 , 11 ] of overall limb difference.
In the United States, more than 32,500 children have experienced a major pediatric amputation [ 10 ]. The Centers for Disease Control and Prevention highlights an estimate that approximately 1500 children are born with upper-limb reductions each year, or approximately 4 of 10,000 live births [ 12 ]. Internationally, limb reductions vary from 7.8/10,000 [ 9 , 13 ] (France) to 13/10,000 [ 14 ] (Finland), 21.1/10,000 [ 14 ] (Netherlands), and 30.4/10,000 [ 14 ] (Scotland). Due to the variety of complexities limiting both access and affinities to devices, usage rates by amputees with a prosthesis are still limited.
Substantial percentages of people with congenital limb loss or acquired limb loss choose not to use a device, despite having access to one [ 15 ]. Usage rates have been reported for upper-limb devices between 37% [ 16 , 17 ] and 56% [ 18 ] among individuals with upper-limb loss. Lower-limb devices are often viewed as more of a necessity than upper-limb devices and have usage rates that vary in literature between 49% [ 19 ] and 95% [ 20 ]. This difference is particularly expressed among children with transverse upper-limb amputations [ 21 ], where usage rates fall between 44% and 66% [ 22 , 23 , 24 ].
Low usage rates of upper-limb prostheses may result from a lack of aesthetic design, weight, availability of insurance and health care, and high costs [ 25 ]. Additionally, device acceptance is complex at the user, provider, parental, and insurance levels. The combination of form and function in the design of prostheses has emerged to provide a higher degree of functionality patterned after the organic 21 degree of freedom human hand [ 2 , 26 ]. Much of the design efforts have been prioritizing achieving a high degree of realism in comparison to the organic analog. Graham Pullin proposed in his book, Design Meets Disability [ 27 ] that prostheses should not be limited to functional design and that duality should exist between aesthetics and functionality.
Limited research has focused on the benefits of improved aesthetics of prosthetic limbs [ 28 ]. Research has found that those with limb difference may have lower self-esteem and higher concern of others’ negative overall perception of their body image [ 29 ]. Psychosocial development effects and quality of life considerations for this demographic are still being understood. Research has found that those with limb difference can have lower self-esteem and an overall negative perception of their body image. Donovan et al. [ 28 ] highlighted the use of a prosthesis improved social engagement and confidence in those with limb difference. This study did not focus on the visual treatment or appearance of the limbs. Murray [ 30 , 31 ] approached their studies from the prosthesis user’s perspective and found that prostheses use improved the psychoemotional health of those who wore them. Additional research investigating both the functional benefits of prostheses and the role of aesthetic design on the user’s psychosocial development could lead to improved design considerations.
2. Contemporary Issues
3D printing is becoming an integral part of upper-limb prosthesis, resulting in response to several tangible issues, including reduced access to conventional prostheses in a timely manner and, in some cases, restricted access. This review focuses on the history of 3D printed prostheses, the populations they support, and the concerns that have driven the work. This is followed by a discussion on the path forward to link the current outcomes into the medical system.
2.1. Prosthetic Limb Abandonment
Expectations and daily goals for patients using their prosthetic device have been shown to differ depending on the style of the device the patient receives [ 32 ]. An investigation found for device users that the relative importance of many factors, including comfort, vary based on the perception of the device [ 32 ]. It can be surmised that a device with increased hand articulation will change the user’s expectation of needed comfort, decreasing its relative weighted importance to the user. The promise of increased control establishes an expectation and level of importance for robust performance. It has also been seen that the factors that contribute to rejections change with the type of amputation method, gender, and age [ 25 ]. Congenital limb loss patients are more likely to reject and forgo using a device as an adult, while females with acquired limb loss were more likely to reject devices than their male counterparts [ 25 ]. Prosthesis abandonment is a major issue in all populations and can be caused by many reasons. In the adult population, sensory feedback, appearance, function, control, comfort, and durability were all cited as key areas in need of study concerning prosthesis design and acceptance [ 25 ].
Biddiss et al. [ 33 ] reviewed over 200 research articles and found that pediatric rejection rates ranged from 38% for passive devices, 45% for body-powered devices, and 32% for electric devices. For adults, rejection rates range from 39% for passive devices, 26% for body-powered devices, and 23% for electric devices. Myoelectric devices were not prevalently seen in long term follow-up [ 34 ]. The factors driving rejection rates for devices necessitate a conversation of how new approaches can be taken to improve user affinities and outcomes.
2.2. Appearance
Those with limb difference can be ostracized due to their perceived impairments. The benefits of having a prosthetic limb can help eliminate some of that stigma. The additional inclusion of art and design in prosthesis development can further empower those involved. Goffman [ 35 ] theorized that stigma or rejection by others lead those with disabilities to try to compensate by adopting practices that would hide the disability. This response may be seen in those with limb difference adopting behaviors like holding their different limb behind them for pictures, diminishing the ability for viewers of the picture to notice.
Prostheses for children have often comprised a body-powered hook or a skin tone colored passive device. Even at the current time of publication, passive or body-powered devices with a hook, which may have a silicone glove for aesthetics, are still a common course of care [ 25 , 32 ]. Current trends in prostheses are to push normalization and reduce the level of stigma a user may encounter. Frank [ 36 ] has shown that early prosthesis users have found empowerment through various methods. These positive interactions are not limited to the user’s social engagement but also allow the individual to develop their personal acceptance of their limb difference. This development is a complex process [ 29 ]. With modern materials, the ability to simulate a natural limb look is becoming more feasible. Huang et al. [ 37 ] proposed a LivingSkin™ silicone elastomer gloving material and novel motors to a more realistic look without increasing weight. These devices aim to project a natural appearance; as seen in other fields, art can give those with disabilities ways to express themselves while also increasing their self-esteem [ 38 ].
Our research team has theorized that the blend of aesthetic design for functional prostheses, including those that diverge from traditional human form, may be able to support the development of positive social identities and interactions. This will be a point of investigation for the investigators as the research progresses.
2.3. Function and Control
Three methods for control of modern bionics include [ 39 ]: (i) Body-powered through cable extension or contraction, (ii) button press [ 40 , 41 ], and (iii) electromyography (EMG). All three methods provide unique experiences that users may prefer for use in daily life. Body-powered devices require the complete movement of a different section of the body to move a mechanical section of the hand, claw, or fingers. This additional functionality goes beyond the cosmetic and looks to address specific tasks the individual will encounter in daily life. The selection of a prosthesis is often based off a patient’s particular needs, experience, and functional requirements [ 42 ]. Often, in the case of children, their participation in the selection process can be limited. Long duration studies comparing patient outcomes of children fit with devices to those without have not been well reported [ 43 ].
Body-powered devices are more predominantly prescribed in the United States and have, in many cases, been viewed as a more robust option than myoelectric devices [ 25 , 32 , 34 ]. Body-powered devices provide users with a physical sensation feedback, while myoelectric devices may only provide visual feedback [ 44 ]. Because of this and further challenges related to robustness, training, and technical limitations, such as overall system weight [ 25 , 32 ], many professionals and users are reported to prefer body-powered devices. When using skin movement or button-press techniques, these control schemes require the use of an essential part of the patient’s body to control this function, which may result in a high degree of false positives, giving the user a bad experience.
Electromyography uses the measurement observation of the resulting electrical potential of a muscle during contraction [ 45 ]. This method has stood out due to its many benefits, including small form factor, reliability, and stability. An EMG sensor that has an amplification and rectification circuit to condition the signal and an integrated band pass filter to remove unwanted artifacts and noise can capture the intentionality of the user through a muscle contraction. This filtered signal is able to correlate the intensity of a patient’s contracted muscle, which can be used in actuating the electromechanical hand’s function states. Many prosthetic systems use a group of sensors placed on a set of local muscle groups to capture a set of signals for engagement, or calibrate the resulting signal set derived from various organic (amputated) limb motions [ 46 , 47 ].
3. A New Approach to Prosthetic Limb Design
Being able to digitally share 3D design models through the internet has led to a growing maker community globally. A carpentry accident in 2011 led to a global collaboration in an effort to regain some of the carpenters’ lost dexterity due to the loss of several fingers [ 48 ]. The carpenter, Richard Van As, enlisted the help of a mechanical special effects artist Ivan Owen. This collaboration led to the world’s first 3D-printed upper-extremity prosthesis device in 2012, and the designs were uploaded as an open-source format for the global maker community to reproduce and evaluate. This body-powered device utilized the wrist-flexion of the residual limb to activate uniform contraction of the phalanges and is featured in Figure 1 .

The Robohand assistive device, first made available for 3D printing globally via Thingiverse. Image from the Food and Drug Administration https://www.flickr.com/photos/fdaphotos/9564033498 .
3.1. The Rise of 3D Printed Prosthetic Arms
The availability of the designs led by both the Robohand and the designer Ivan Owen had a lasting effect globally on the potential application for a variety of accessibility technologies. This inspired researchers and maker enthusiasts to contact the designers wanting to have a similar impact. Upon seeing the effectiveness and impact of collaborative design and production, several maker communities and nonprofit organizations were developed to support local access in their communities, including: e-NABLE ( http://enablingthefuture.org/ ), Enable Community Foundation ( http://next2.e-nable.me/ ), Robohand ( http://robobeast.co.za/rich-van-as/ ), and Limbitless Solutions ( https://limbitless-solutions.org/ ). These groups have included both local at home designers as well as research groups based at various universities, including: Rochester Institute of Technology (RIT), Creighton University, University of Central Florida (UCF), and the University of Washington at Bothell. Additive manufacturing techniques have utilized everything from home-built kit 3D printers to industry-grade machines. While much of the work has been done for body-powered devices, some groups have pushed the research on electromyographically actuated devices to accommodate for higher degrees of limb loss via biosensing and electromechanical motors [ 49 ].
Custom sizing, designed specific to the end-user through either volumetric scaling or more precise parametric tailoring, allowed for rapid production and iteration. While many printers now allow for a variety of colors of filament materials, the same base 3D model could be constructed with a user-specified color scheme. This has provided additional affinities and involvement for participatory design. In 2014, a conference for “Prosthetists Meet 3D Printers” was held at Johns Hopkins Hospital, bringing together the maker community and medical professionals, including surgeons, prosthetists, and therapists, to discuss the use of 3D printing for improving access and quality of care [ 50 ]. This conference and the e-NABLE web platform were coordinated by a team, including Jen Owen and Jon Schull. The conference brought together a significant amount of limb-different individuals, designers, and medical professionals.
Collaborative design efforts, many utilizing cloud-based real-time design software such as Autodesk Fusion 360, allowed for group support and advances in the functionality, robustness, and a user-driven feedback opportunity. The Enable network developed substantial advances, which were made available through the Thingiverse.com website’s open-source, with attribution, repository. These design schematics and an image of an example printed and assembled part were made available ( https://www.thingiverse.com/thing:476403 ) and are presented in Figure 2 . These devices have reached new heights of accessibility for children all over the globe, made possible due to the availability of open-source customizable designs and new 3D printers used in schools, libraries, and even residences [ 51 , 52 ].

The Raptor reloaded hand by Enable available for download via Thingiverse. ( a ) Exploded view of design and user assembly methods. ( b ) Completed assembly of device. https://www.thingiverse.com/thing:476403 .
This type of global support has allowed for an accelerated prototyping phase utilizing such collaborative design mindsets. A repository or “family tree” of how the designs and global chapters have progressed is visually available on the https://e-NABLE.org website, including a full visualization at https://kumu.io/jonschull/devices .
As the maker movement has continued to propagate, there has been integration with the university research environment. Some research groups have sought to standardize production methodologies and establish best practices through data-driven analytics. One example is the work of Jorge M. Zuniga, established during his time at Creighton University (at time of this paper’s publication, at the University of Nebraska). Their work [ 53 , 54 , 55 ] has pushed the design efforts and field regarding the implementation of additive manufacturing to accelerate bio-medical research and its translation to the medical environment. Their design of the Cyborg Beast hand, a wrist powered design, built on the prior work and improved integration and assembly challenges, has seen significant implementation for children with limb differences and is pictured in Figure 3 .

The Cyborg Beast by Creighton University’s Jorge M. Zuniga and available on Thingiverse https://www.thingiverse.com/thing:261462 . ( a ) Personalized assembled device. ( b ) A group of assembled hands featuring different cosmetic treatments.
One of the founders and curators of the e-NABLE movement, Jon Schull, and his research team developed significant contributions to the field at RIT, including new body-powered forearms and hands actuated by elbow or wrist movement. This has led to substantial developments for introducing new educational techniques incorporating project-based learning [ 56 ] utilizing 3D printers and global design networks [ 57 ].
3.2. Appearance-Cooperative Expression
Design work using 3D printers has allowed for a higher degree of individual customization of devices. As the collaborative minded network of developers has grown, the role of user-driven design points has been of significant emphasis. In an effort to improve affinities to bionic designs, participation by the end-user has been prioritized. This effort, applied by our research team to prosthesis design, is described as “cooperative expression” and is built on the methodology of participatory design and its strategies, such as cooperative inquiry.
Participatory design represents a field of research, distinguished by a variety of methods, investigating the role of direct user participation with designers [ 58 ]. While this was originally discussed for computer-based systems in the workplace, it can also be applied for learning from children and their perspectives while in the development of low-tech design prototypes [ 59 ]. In the design process, specific inquiries in the brainstorming methods have become known as cooperative inquiry [ 59 , 60 ]. Druin et al. [ 59 ] categorized three dimensions related to children as design partners to be considered, including: (i) The child’s relationship to the participating adults, (ii) their relationship to the technology, and (iii) the goals for the inquiry. Cooperative inquiry, when applied as a method of developing technology, is flexible in construct. Foss et al. [ 61 , 62 , 63 ] applied the technique to study the role of children with special learning needs and adults as partners in the design process of software while empowering children to customize their experience [ 62 , 63 ]. Their study using a cooperative inquiry approach found reports of emotional engagement in children when the method was applied. Ultimately, this higher engagement is speculated to develop more ownership of the project for the children [ 62 ].
A new modified participatory design approach, entitled cooperative expression, is now being applied to visual aesthetic treatment in an effort to improve affinities to the bionic limbs. Our design team’s efforts have taken this participatory and cooperative approach to support the customization of the aesthetics for 3D-printed bionic limbs. Recipients of the bionics have the ability to artistically customize their interchangeable sleeves using an interactive website. Various 3D designs can be compared, selected, and further personalized with customizing color and effect regions. Artists support the initial creation of the aesthetic scaffolding, such as the design of color pallets and the discretization of the zones for customization. This scaffolding is designed to provide an initial framework to minimize selection fatigue and maximize the participants’ ability to explore artistic designs potentially outside their reference frame.
This unique customization design process and methodology highlighted in Figure 4 looks to integrate the end-user from start to finish in the design process. The structural and mechatronic components of the arm have been standardized for the digital designers to create a digital 3D representation of the artistic shell. An interactive web portal allows the user to customize colors and effects and regions of the sleeve, allowing expressive visualization of the final design. In some cases, modifications are made to the artistic design visualized on the user portal to support the human–machine interface. Production of the aesthetic sleeves uses an interdisciplinary process including: 3D printing, surface preparation and priming, automotive finishing techniques, and painting. During the painting process, artists capture the effects and colors selected through the user portal and deepen the visual effect. The full system is then validated and prepared for fitting to the participant. This system allows participants to be actively involved with their arm before production or fitting, and an example interaction is presented in Figure 5 . This early interaction is anticipated to establish an emotional connection to the limb before the participant is fitted.

Overview of design process and methodology from design generation, user participation, and interdisciplinary manufacturing.

( Left ) Example interactive web page for children to customize color and effect regions during the design process, and how user participation can be translated to ( Right ) the final design with artistic input from art team and production teams. Sleeve design made in partnership with Riot Games.
Part of the design process offers the ability for different categories or “empowerment classes” of interchangeable aesthetic sleeves. These classes are broken down into four individual groups, Warrior, Shadow, Ethereal, and Serenity . These classes are designed to represent different personalities linked to emotional affinities. Artists create these inspired 3D models to connect with these personalities, and in some cases, external artists representing characters have added designs to the catalog. Examples of the “empowerment classes” are presented in Figure 6 . This variety coupled with the interchangeable options allows the child to have more freedom over their expression; this should improve development of affinity to the device, lower social stigma factors, and support a longer-term engagement, thereby improving user performance. An evaluation of the role of the device on psychosocial development and stigma factors will be conducted in future research.

3D-printed electromyographic actuated limb device with interchangeable artistic covers from Limbitless Solutions at the University of Central Florida. ( a ) Warrior class, ( b ) Ethereal class, ( c ) Serenity class, and ( d ) Shadow class.
3.3. Function–Electromyography
Antfolk et al. [ 44 ] found that a 16-sensor EMG was capable of predicting participants’ desired control with 86% accuracy after a two-day training session. This system was used with a 25-year-old male transradial amputee. The result was produced by the system learning how the user’s EMG input should be interpreted, based on movements performed similarly to how they would have been prior to the amputation [ 44 ]. Much of the increased complexity arises from the use of multiple EMG inputs mapping to multiple computer-controlled outputs. Each additional monitored region requires intentional actuation of a corresponding muscle group and, in some cases, simultaneous actuation [ 64 ]. When used by children, this complexity may be overwhelming and has led to a reported impact on rejection of devices [ 33 ].
This team’s unique 3D-printed prosthesis leverages a single EMG measurement, which may support simplicity in daily calibration and application. On-board signal processing can correlate the intensity of the measured muscle contraction or the number of contractions in a specific time period. This can result in actuating different types of hand-state gestures, including individual finger actuation motor position. The limitations associated with current EMG devices are an opportunity to examine improvements to both the design and training methodology.
3.4. Control-Gamification and Training
Single-surface EMG providing multigestural control allows the user, using contraction intensity and patterns, to control their prosthesis. Due to the complexity of the controls, a custom video game-based training system was developed to train the user in a risk-free environment. The video game system collects the filtered EMG input from the user and routes it to the computer system; where it is either interpreted as a multifunction controller or analog input. Training systems with mechanics that are similar to the active arm control, similar to punching or slapping, using an EMG controller have increased usability scores [ 65 ].
This research team’s newest game for the prosthetic arm training, called Magical Savior of Friends (MSOF), places the character in a ‘Mario style’ side-scrolling game with a magical character that can initiate superpower attacks and defenses based on the amplitude of their contraction. After going through a thresholding calibration sequence, the player is able to vary their measured contraction magnitude, which correlates to how hard the player activates their muscle contraction. For example, calibration can be set for low, mid and max thresholds. Calibration for setting the low threshold is applied to move the reading above the noise threshold. In application, these gestures initiate three completely different superpower attacks to occur.
While the game is designed to be fun and approachable on the surface, it provides meaningful training through simulation to learn complex multigesture hand states. Preliminary results are promising, as players become significantly more accurate with the tuned contraction after playing the game for one hour [ 66 ]. By gamifying the training, this method provides many opportunities for failure and feedback in a safe low-pressure environment. Multiple studies have found that feedback is crucial in improving control of a myoelectric prosthesis [ 33 , 44 ]. Disguised as a game, simulation is effective in allowing practice and training the prosthesis user. An extension of this work is underway to provide prosthesis users with new opportunities to play games they otherwise might not have the dexterity or proper interface for.
3.5. Comfort and Durability
3D printing, as with most manufacturing processes, has its advantages and limitations. The promise of personalized medicine and ability to rapidly produce models has been shown to be effective in the clinical setting [ 67 ]. While not significantly impacting professionals that use 3D printing to help to plan procedures via the production of representative models, concerns for the safety of 3D-printed parts have been expressed [ 68 ]. Professionals using material properties to optimize design must be aware of the manufacturing process’ impact. In testing of additive manufacturing ABS plastic, samples have been reported to have variable mechanical properties based on print orientation and are estimated to have between 10% to 73% of the strength of samples produced by injection molding [ 69 , 70 ]. While the method through which layers are printed has an impact on the deviation from material standards, optimization can be used to improve reliability and predictability of performance [ 69 , 71 ]. In an effort to have consistent and reliable 3D-printed components for use in the medical environment, consistent standards and best practices should be implemented. While FDA guidelines make many recommendations toward work-flow and documentation for part tracking in the event of a part failure [ 71 ], better understanding the underlying principles behind part vulnerability can help to minimize those risks preemptively. When designed with realistic loading expectations and considerations for manufacturing, additive manufacturing can provide stable and resilient parts that can reduce overall system weight and manufacturing costs [ 72 ].
4. Discussion of Regulatory Framework
While a tremendous body of work is available on attempts to advance the novel manufacturing techniques, reviews of various studies have identified areas for continued work [ 73 , 74 ]. An independent review [ 73 ] of 314 current studies evaluated for levels of evidence and validity highlighted a trend of the reports to be more in line with case studies as opposed to randomized control trials. Several areas of evidence and clarity, including sufficient study power, statistical tests, reliable outcome measures, and clarity in recruitment, were all identified as needing expounding. The review [ 73 ] called for a significant appraisal of both efficacy and effectiveness to provide healthcare professionals with more information to make critical decisions on readiness for broader patient care.
In order to advance the state-of-the-art and to quantify the impact and effectiveness of our research team’s new 3D-printed electromyographically actuated multigesture arms, a novel clinical trial has been proposed in collaboration between Oregon Health & Science University and the University of Central Florida. This study is considered nonsignificant-risk. Twenty patients between the ages of 6 and 17 will participate in a one-year clinical trial with four total assessments. Assessment has been designed in two parts: Influence on quality of life (Children’s Hand-Use Experience Questionnaire (CHEQ) and PedsQL) and myoelectric control (Assessment of capacity for Myoelectric Control (ACMC)).
CHEQ uses a four-category rating scale to assess the functionality and limitations of a child, developed for ages 6 through 18 years old, and is available on the internet ( www.cheq.se ) for easy access [ 75 ]. It uses a variety of questions with a nested structure, such as: “The first question reads: ‘Is this something you usually do independently?’ and has the response options:
- ‘yes’
- ‘no’
- ‘I get help/avoid doing it’
- or ‘not applicable’.
If the answer is ‘no’ or ‘not applicable’, the item is scored as missing, and the respondent moves to the next item. If the answer is ‘yes’, the second opening question appears: ‘Do you use one hand or both hands together?’, with the response options:
- ‘one hand’
- ‘both hands’
- ‘with the involved hand supporting but not holding’
- ‘both hands, with the involved hand holding the object’.
This type of assessment [ 75 ] provides a reliable baseline to understand how the child’s daily life is influenced by their limb difference.
PedsQL is a well-validated survey that asks twenty-three questions of both parents and children about various aspects of health-related quality of life over the past month. Published results are available for the general population. The PedsQL scoring algorithm translates the available responses to questions (“never”, “almost never”, “sometimes”, “often”, or “almost always”) into scores of 0%, 25%, 50%, 75%, and a maximum of 100% for each of the four generic core scales (Physical Health, Emotional Functioning, Social Functioning, and School Functioning).
Assessment of capacity for myoelectric control (ACMC) [ 76 , 77 ] is a Rasch rating scale that is used to detect expected change in a person’s ability using objective variables. There are 30 items that evaluate a prosthetic arm’s ability to do specific functions that involve gripping, holding, releasing, and coordinating between limbs. This is done by asking the subject to perform certain tasks and scoring their motions, including traditional chores such as making simple meals and setting a table. Additional questions look at hobby and leisure activities, such as assembling a simple project such as LEGO bricks [ 76 , 77 ]. The occupational therapy team supporting the evaluation will support evaluation of performance, with feedback from the study participants documented. The findings from this study will be reported following the assessment and evaluation, and the data used to continue to improve both the design methodology and study methodology.
5. Conclusions
The outlook for using 3D printing manufacturing techniques and collaborative design is bright, with rapidly progressing iteration and designs that can better develop affinities for users. At this time, limited work has been reported involving sufficient power and clinical assessment [ 73 , 74 ]. By designing and conducting novel clinical assessment of these electromyographic 3D printed bionic limbs with well defined outcome metrics, this may lead to being able to add to the field and better capture the readiness for broader distribution. Continuing efforts to validate and assess both design and performance will improve translation of the technology and design methods. The process for designing specifically for the end=user with significant reduction in costs may radically change the accessibility of functional prosthesis for pediatric patients.
Acknowledgments
The authors would like to thank the many members of the Limbitless Solutions team who have supported the project throughout its development. Our appreciation to the corporations and external artists from both Riot Games and 343 Industries for supporting additional designs in the sleeve catalog.
Author Contributions
This paper involved author contributions including conceptualization, A.M., P.S. and A.C.; writing–original draft preparation, A.M. and P.S. with support from J.S., M.D., D.C., A.K., I.W. and A.C.; and writing–review and editing, all authors.
This research received no external funding.
Conflicts of Interest
The authors declare no personal conflict of interest, but want to disclose that the Limbitless Solutions organization has received support from the 3D printer manufacturer Stratasys.
Thank you for visiting nature.com. You are using a browser version with limited support for CSS. To obtain the best experience, we recommend you use a more up to date browser (or turn off compatibility mode in Internet Explorer). In the meantime, to ensure continued support, we are displaying the site without styles and JavaScript.
- View all journals
- My Account Login
- Explore content
- About the journal
- Publish with us
- Sign up for alerts
- Review Article
- Open access
- Published: 03 June 2024
A comprehensive review of sustainable materials and toolpath optimization in 3D concrete printing
- Zicheng Zhuang 1 na1 ,
- Fengming Xu 1 na1 ,
- Junhong Ye 1 na1 ,
- Liming Jiang 3 &
- Yiwei Weng 1
npj Materials Sustainability volume 2 , Article number: 12 ( 2024 ) Cite this article
226 Accesses
Metrics details
- Climate sciences
- Environmental sciences
The construction sector has experienced remarkable advancements in recent years, driven by the demand for sustainable and efficient building practices. Among these advancements, 3D concrete printing has emerged as a highly promising technology that holds the potential to revolutionize the construction industry. This review paper aims to provide a comprehensive analysis of the latest developments in three vital areas related to 3D concrete printing: sustainable materials, structural optimization, and toolpath design. A systematic literature review approach is employed based on established practices in additive manufacturing for construction to explore the intersections between these areas. The review reveals that material recycling plays a crucial role in achieving sustainable construction practices. Extensive research has been conducted on structural optimization methodologies to enhance the performance and efficiency of 3D printed concrete structures. In the printing process, toolpath design plays a significant role in ensuring the precise and efficient deposition of concrete. This paper discusses various toolpath generation strategies that take factors such as geometric complexity, printing constraints, and material flow control into account. In summary, the insights presented in this paper may serve as guidelines for researchers, engineers, and industry professionals towards sustainable and efficient construction practices using 3D concrete printing technology.
Similar content being viewed by others
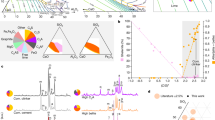
Electric recycling of Portland cement at scale
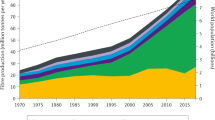
The environmental price of fast fashion
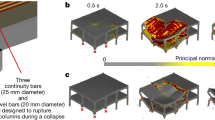
Arresting failure propagation in buildings through collapse isolation
Introduction.
Climate change has emerged as a global challenge due to the substantial carbon emissions and energy consumption. In 2022, the global carbon emissions and energy consumption reached 36.8 gigatons and 14,585 million tonnes of oil equivalent 1 , 2 , respectively. The construction sector is a major contributor to global carbon emission and energy consumption, accounting for 40% and 36% in 2022 3 , respectively. With the urban population estimated to increase to 68% by 2050, the environmental impacts of the construction sector will continuously increase 4 , underscoring the urgent need for developing sustainable construction technologies.
3D concrete printing (3DCP), also known as additive manufacturing (AM) in the construction sector 5 , offers a promising solution for achieving sustainable construction. 3DCP constructs structures by depositing printable concrete materials layer-atop-layer based on a pre-designed building model. The unique construction process possesses the advantages of enhanced sustainability and design flexibility. For example, a prefabricated bathroom unit (PBU) constructed by 3DCP achieved a reduction of 85.9% and 87.1% in carbon emissions and energy consumption, respectively, compared to that of a mold-cast counterpart 6 .
3DCP has gained much attention from both academia and engineering. Figure 1a shows the rapid growth in the publications and citations related to the keywords of “3DCP” based on data obtained from Web of Science. The number of publications reached 444 and 420 in 2022 and 2023, respectively. In these publications, several review works have been conducted in the fields of 3DCP and its potential applications 7 , 8 , 9 , 10 . Wangler et al. 8 present a technical review of 3DCP from fresh materials to hardened materials and further practical applications. Lu et al. 9 provide a comprehensive review of the material behaviors of 3DCP. However, the review articles primarily focus on the technical or material advancements of 3DCP, with less attention given to its sustainability aspects. Figure 1b illustrates the growth of publications related to the keywords of “3DCP and Sustainability”. Despite the growing interest in 3DCP, only 46 publications in 2023, approximately 10% of total 3DCP-related publications, focused on sustainability (Fig. 1a ). Among these publications, Dey et al. 11 provide a comprehensive review of the utilization of industrial wastes in printable materials to improve the sustainability of 3DCP. However, there is a lack of in-depth understanding of how to improve sustainability in 3DCP across its various construction processes.
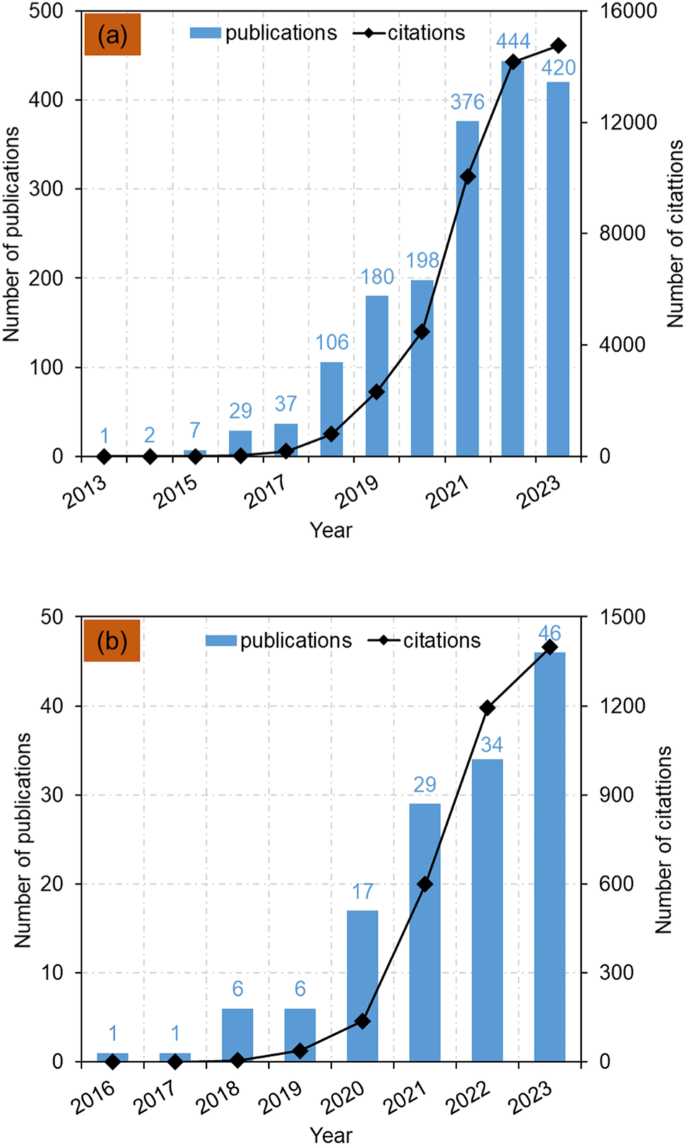
a Keywords of “3DCP”; b keywords of “3DCP and Sustainability”.
The typical construction processes of 3DCP include the development of printable materials, structural optimization, toolpath design, and printing 12 , as shown in Fig. 2 . Each of these processes offers opportunities for enhancing sustainability. At the material level, sustainability can be improved by developing printable materials incorporated with waste materials. The waste materials are used as the substitutions of aggregate and binder contents, thereby reducing the carbon emission associated with the material extraction. At the structural level, the design of hollow structures via topology optimization (TO) 13 , 14 reduces the material usage and thus enhances sustainability. TO involves the optimization of material distribution to achieve the desired performance. In addition, the design flexibility of 3DCP is compatible with the structural TO. Finally, to implement the optimized structure into 3DCP, toolpath design methods 15 , 16 are adopted to determine the efficient path for sustainable concrete printing. The integration of sustainable printable materials, TO, and toolpath design techniques with 3DCP represents a promising synergy for future research and sustainability development in the construction sector. However, comprehensive reviews covering these three aspects are currently lacking in the existing literature.
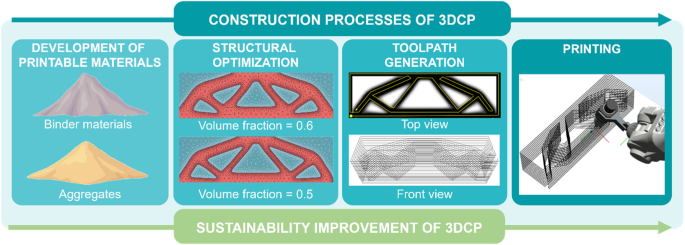
The typical processes include the development of printable materials, structural optimization, toolpath generation, and printing.
This paper aims to fill the abovementioned research gap by providing a comprehensive review of sustainable materials, structural topology optimization, and toolpath planning for the enhancement of sustainability in 3DCP. Based on the findings of these reviewed articles, the perspectives and methods to enhance sustainability with respect to the abovementioned three aspects of 3DCP are highlighted. Finally, Section 5 conclusions are summarized and future research directions are identified.
Sustainable materials in 3D concrete printing
Integrating sustainable materials into 3DCP is a potential strategy for enhancing the sustainability of 3DCP 17 since the construction sector increasingly focuses on the recycling of natural resources, reduction in material waste and carbon emissions. The commonly developed 3D printable cementitious materials consist of binder materials (primarily cement), natural fine aggregates, additives, admixtures, and water 18 . However, two main challenges impede the development of sustainable 3D printable cementitious materials. Firstly, the high usage of ordinary Portland cement (OPC, 700–800 kg/m 3 ) 18 impacts sustainability due to the associated carbon footprint 8 . Secondly, during the printing process, most developed material mixtures only use fine aggregates for 3DCP due to the limitation of the pumping process and nozzle opening 19 , 20 .
Employing sustainable binder and aggregate alternatives is a potential solution to address these challenges 6 , 21 . This section reviews relevant advancements in adopting recycled aggregates and supplementary cementitious materials (SCMs) into 3D printable materials. Figure 3 shows the number of publications associated with the keywords “3D printed concrete”, “Recycled glass”, “Recycled sand”, “Recycled concrete aggregate”, “Recycled plastics”, “Recycled rubber”, “3D printed concrete”, “Silica fume”, “Rice husk”, “Fly ash”, “Limestone”, “Calcined clay”, “Granulated blast-furnace slag” and “Sustainable” from the Web of Science database. As shown in Fig. 3 , a growing academic interest is observed related to recycled aggregates and SCMs. The following sections discuss the performance characteristics and implications of these sustainable materials in 3DCP applications.
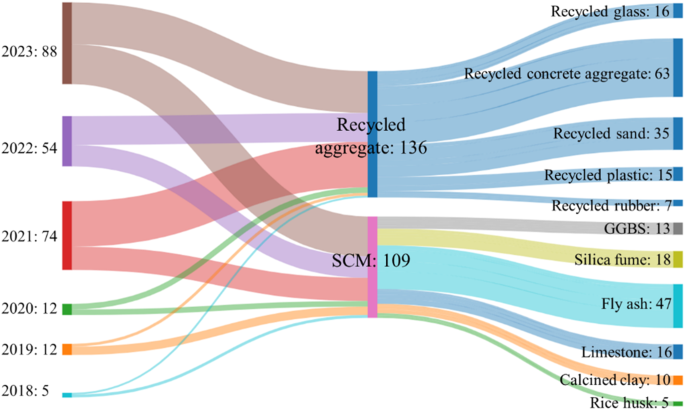
The literature study includes research on two main types of sustainable materials, recycled aggregates and SCM, from 2018 to 2023.
The impact of recycled aggregates, such as recycled glass 22 , concrete 23 , plastics 24 , and rubber 25 , alongside SCMs, such as silica fume 26 , rice husk ash 27 , fly ash 28 , limestone 29 , calcined clay 30 , and granulated blast-furnace slag (GGBS) 31 , on the fresh and hardened properties of 3D printable materials are analyzed. The analysis underscores the importance of these materials in advancing 3DCP sustainability but also reveals the future potential research direction to mitigate environmental impacts and foster sustainable development in 3D printable cementitious materials 19 , 32 , 33 .
3D printable material performance with recycled aggregates
The primary recycled materials in 3D printed concrete for sustainability enhancement include sand 34 , glass 22 , concrete 35 , plastics 24 , and rubber 25 . According to the data from the Hong Kong Environmental Protection Department in 2022 36 , daily waste generation in Hong Kong includes 222.6 tons of glass and 2336.9 tons of plastics. In addition, concrete and sand, derived mainly from construction and demolition debris and construction waste, account for a considerable portion of the waste, with daily production of construction waste reaching 49,865 tons 36 . In the blueprint for Hong Kong 2035 37 , the government proposes a new target concerning “Waste Reduction, Resources Circulation, Zero Landfill”, which presents a significant challenge for the recycling of waste materials in sustainable construction.
In 3DCP, it is essential to achieve a balance of fresh properties and hardened properties for printable materials. Fresh properties such as printability and pumpability, hardened properties such as strength and durability, and sustainability are critical factors for material tailoring 19 , 38 . Recycled aggregates are sustainable alternatives to natural aggregates, helping to conserve natural resources and reduce land waste from landfills 24 , 39 , 40 , 41 . This section discusses the various recycled aggregates in 3DCP (see Table 1 for details) to illustrate their impact on material fresh and hardened performance as well as sustainability.
Impacts of recycled aggregates on fresh properties
Summarizing the findings from Table 1 , the usage of recycled aggregates impacts the fresh properties of cementitious materials. The fresh properties are critical factors, which determine the printability of materials during the printing process. The printability can be characterized by workability, pumpability, extrudability, and buildability 34 . In the 3D printing process, the most essential steps are conveying mixed materials to the nozzle via a delivery system and depositing materials to build the solid object in a layer-by-layer manner 42 . In the conveying step, the materials are required to have good workability and pumpability, which indicates how easily the material can be conveyed. In addition, extrudability indicates the ability of a material to be extruded with minimal energy consumption during the delivery 43 . In the deposition step, the materials are required to have good buildability, which indicates how well the materials can be stacked stably.
With respect to workability, research has indicated that the presence of recycled sand, characterized by its high water absorption rate and irregular shape, tends to reduce the workability of concrete 44 . Similarly, incorporating recycled rubber particles with poor shape and rough surfaces has diminished the workability of 3D printed concrete, resulting in the slow relative motion of rubber particles within the concrete mixture, causing reduced processability 25 . In terms of pumpability, studies conducted by Ting et al. 45 have shown that adding recycled glass to concrete reduces its pumpability. This phenomenon can be attributed to recycled glass particles’ angular and sharp-edged nature, which obstruct flow and decrease pumpability.
Analyzing the extrudability in recycled aggregate concrete, it has been observed that the high water absorption of recycled sand necessitates the addition of extra water and superplasticizers to enhance the extrudability of 3D printed concrete 34 . In addition, the water-absorbing nature of surface cracks in recycled rubber can result in reduced extrudability. However, subjecting recycled rubber to heat treatment can partially close these surface cracks, reducing water absorption and significantly improving extrudability 46 .
Finally, with respect to buildability, increasing the substitution rate of recycled concrete aggregates has been found to improve the buildability of 3D printed concrete. Liu et al.’s 35 research suggests that the buildability increases with the rising substitution rate of recycled concrete aggregates due to the reduction in concrete density. Conversely, studies involving recycled plastics have revealed that while plastic’s hydrophobic nature enhances material flow, it also delays the hydration reaction of calcium silicate, slowing the thixotropic behavior of concrete and ultimately reducing its buildability 24 , 41 .
In summary, recycled aggregates’ influence on cementation materials’ fresh properties is multifaceted and crucial for 3D printing applications. Workability can be compromised by recycled sand and rubber, while pumpability may be hindered when using recycled glass due to its angular characteristics. Extrudability can be improved with additional water and heat treatment for specific recycled materials. In addition, buildability is positively correlated with higher substitution rates of recycled concrete aggregates, while challenges arise from the delayed hydration reaction of calcium silicate when recycled plastics are involved. These insights underscore the need for careful material selection and processing adjustments to optimize the performance of 3D printable materials.
Impacts of recycled aggregates on mechanical properties and sustainability
The mechanical performance of printed structures is paramount for ensuring their structural integrity and safety. Table 1 summarizes the mechanical properties of various types of recycled aggregates, revealing their impact on the mechanical properties of 3D printed concrete. Specifically, incorporating recycled materials such as recycled sand, coarse aggregates, glass, and plastics as sustainable alternatives in concrete leads to decreased compressive strength with increasing substitution rates 22 , 24 , 34 , 35 . This reduction in compressive strength can be attributed to the increased porosity within the concrete resulting from the addition of recycled materials, with higher porosity leading to reduced compressive strength 24 , 39 .
Beyond the problem of increased porosity, the bond between recycled aggregates and the cement matrix plays a significant role in the mechanical performance of 3D printed concrete. The smoother surface and sharper edges of recycled glass particles compared to that of natural sand particles may result in weaker bonding between the particles and the cement matrix at the interface transition zone, decreasing mechanical strength 45 . The inherent properties of recycled aggregates also impact the strength of 3D printed concrete. Recycled concrete aggregates containing old mortar and aggregates with adhering old mortar, which have lower mechanical properties, can serve as weak areas of a structure, decreasing mechanical performance 35 . On the contrary, adding cement-coated modified recycled rubber in 3D printed concrete enhances its compressive strength. This enhancement is primarily attributed to the transformation of the rubber from a hydrophobic material to a hydrophilic material after modification, promoting its interaction with the fresh mortar during mixing and resulting in a more compact interface transition zone within the structure 33 .
These findings emphasize the necessity of incorporating recycled aggregates in 3D printed concrete in appropriate amounts after considering the structural integrity and safety to achieve the desired overall properties of 3D printed concrete. As a type of sustainable material, utilizing recycled aggregates in 3DCP can reduce material costs and mitigate environmental impacts 47 . Han et al. indicate that as the proportion of recycled aggregates increases from 0% to 100%, the CO 2 emissions of 3D printed concrete decrease from 5637.647 kg to 5499.505 kg 48 . Cost analyses demonstrate a downward trend in the total cost of 3D printed concrete with the increasing proportion of recycled aggregates. For instance, the costs of 3D printed concrete with recycling proportions of 0%, 50%, and 100% are 12,913.54 CNY, 12,555.77 CNY, and 12,194.97 CNY, respectively 48 . This underscores that increasing the proportion of recycled aggregates can effectively reduce greenhouse gas emissions during concrete production and enhance building materials’ sustainability in the practical application.
3D printable material performance with supplementary cementitious materials
This section explores the impact of supplementary cementitious materials (SCMs) on the performance of 3D printable cementitious materials. In the area of 3DCP, a significant aspect is its heavy reliance on OPC compared to traditional concrete 18 . Specifically, 3D printable cementitious materials contain more than 20% of OPC, expressed by mass weight due to the requirements of printability 19 . Including SCMs in material mixtures is an alternative solution to address his problem. Various types of SCMs have been adopted for the mixture design of 3D printable concrete in the existing literature, such as fly ash, ground granulated blast furnace slag, and calcined clay from various industrial processes 49 . Fly ash, a residue from coal combustion in power plants 50 , and calcined clay, derived from high-temperature treatment of clay materials 29 , are among these industrially sourced SCM. In addition, GGBS originates from the milling process of waste slag from steel production 51 , while silica fume comes from silicon ferroalloy smelting 52 , and rice husk ash is a by-product of rice milling 27 . Incorporating these SCMs reduces the environmental burden associated with concrete production and addresses the high carbon dioxide emissions from cement production 29 , 53 .
In the selection of SCMs for 3D printable concrete, optimizing characteristics such as fresh properties, mechanical properties, durability, and sustainability is crucial 29 , 30 , 54 . These attributes directly impact the efficiency of the printing process and the performance of the final structure. Table 2 summarizes the material characteristics of individual SCM used in 3DCP and their impacts on the performance of 3D printable concrete by a systematic literature review.
Impacts of SCMs on fresh properties
Based on Table 2 , the utilization of SCMs affects the printability of 3D printed concrete. These parameters serve as crucial indicators of the stability and performance of materials during processes such as pumping, extrusion, and bearing continuous printing layer loads. In terms of workability, adding silica fume reduces the workability of 3D printed concrete. This is primarily attributed to the high surface area of silica fume, which easily aggregates with cement particles to form flocculent structures, partially hindering the free flow of water, and therefore, affecting the workability 26 , 53 .
In terms of pumpability and extrudability, the appropriate addition of fly ash and GGBS can enhance the pumpability and extrudability of cementitious materials. This is primarily attributed to the spherical and smooth surface characteristics of fly ash 55 and GGBS 56 , as shown in Table 2 , and therefore, contribute to improving the extrudability of concrete. However, excessive fly ash and GGBS may diminish extrudability due to increased water absorption. As the dosage increases, the water absorption rate rises, resulting in increased viscosity, thereby impeding the extrusion process during 3D printing 57 . The replacement of cement with silica fume 26 , rice husk ash 27 , limestone, and calcined clay 29 can enhance buildability. For example, torque viscosity rises while flow resistance and thixotropy are decreased with the rise of fly ash-to-cement ratio, negatively impacting the buildability 55 . Conversely, the influence of the silica fume-to-cement ratio shows an opposite trend on rheological properties as compared to that of the fly ash-to-cement ratio. Adding silica fume increases the filler content in concrete, strengthening the interaction between particles and thereby improving the 3D printing performance of the material 50 . Rice husk ash exhibits strong water absorption capability, reducing voids between concrete particles and promoting flocculation and hydration product formation, thereby enhancing the buildability of 3D printed concrete 27 . The addition of limestone and calcined clay can enhance the buildability due to the reduced water film thickness 30 .
In summary, incorporating SCMs significantly impacts the workability, pumpability, extrudability, and buildability of 3D printed concrete. While silica fume reduces workability due to its high surface area 26 , fly ash and GGBS can enhance pumpability and extrudability when added appropriately. However, excessive amounts may hinder extrudability due to increased water absorption 57 . Substituting cement with fly ash, silica fume, rice husk ash, limestone, and calcined clay enhances buildability 26 , 27 , 58 .
Impacts of SCMs on mechanical properties and sustainability
The mechanical performance of 3D printed concrete is crucial for construction practices. Incorporating SCMs can reduce the environmental impact and directly influence the mechanical properties of 3D printed concrete. Studies have shown that materials such as silica fume 26 , limestone, and calcined clay 29 can positively impact the mechanical properties of concrete. Silica fume acts as an inert filler in 3D printed concrete, filling voids, improving pore structure, and therefore, enhancing mechanical performance 50 . Liu et al. 26 attributed the improvement in the mechanical properties of silica fume to the fact that silica fume increases the density of the concrete, which increases the pore densities and reduces the number of connecting and oversized pores.
Moreover, the quantity of SCMs added also affects the mechanical properties of 3D printed concrete. Increasing the content of limestone and calcined clay can increase the amount of fine particles in concrete, promoting microstructure development 54 . However, small additions of fly ash and GGBS can enhance mechanical properties but excessive amounts may compromise concrete strength. This is mainly due to that the high amount of replacement of cement with fly ash or GGBS reduces the initial cement hydration at the early stage 57 . As a result, the mechanical performance of 3D printable concrete decreases. Therefore, when designing formulations for 3D printed concrete, it is essential to consider the type, quantity, and interactions of SCMs to achieve optimal mechanical performance and ensure the sustainability and durability of structures.
In 3DCP, the CO 2 emission in the material production stage is 583.1 kg CO 2 -eq/m 3 , 75% of which is contributed by the production of cement and other binder materials 18 . Therefore, using SCMs as the substitution of binder materials showed possible advantages in enhancing the environmental sustainability of 3D printable concrete 26 , 28 , 54 . Most of the reviewed studies focus on the fresh and hardened properties of 3D printable concrete with SCMs, with limited attention to the quantitative carbon emission assessment of the materials. Long et al. 59 reported that the 3D printable Limestone & Calcined clay cement composites (LC3) reduced carbon emission by 45% and energy consumption by 40%. Conversely, Yao et al. 60 reported that the carbon emission of printable materials was when geopolymer was used as the binder material. The increased carbon emission of geopolymer was due to the use of silicate (alkaline activator). Liu et al. 61 reported that the printable materials with fly ash showed less carbon emission compared to that of the printable geopolymer concrete. Different conclusions were drawn from the existing articles in terms of the carbon emission of 3D printable materials with SCM. Therefore, to comprehensively assess the sustainability effectiveness of SCMs in 3DCP, additional research is necessary in future works by conducting the quantitative carbon emission assessment.
Conventional structural topology optimization methods
Traditional design principles and considerations are being re-evaluated to leverage the unique capabilities provided by 3D printing 62 . This section aims to review the specific structural optimization methods and considerations tailored for 3DCP technology, with a particular focus on the potential to create functional, efficient, and sustainable designs using topology optimization approaches.
Structural topology optimization is the process of arranging the distribution of materials within a specified design domain to maximize specific mechanical or physical properties, while adhering to prescribed constraints. This concept arose in 1904 when Michell proposed a theoretical analysis to obtain the lightest truss 63 . The advent of finite element analysis (FEA) and the development of the widely used homogenization method 64 , 65 in the late 1980s significantly progressed this concept. Since then, the field has seen substantial advancements, thanks to methods such as Solid Isotropic Microstructure with Penalization (SIMP) 66 , Evolutionary Structural Optimization (ESO) 67 , Bi-directional Evolutionary Structural Optimization (BESO) 68 , 69 , and level set method 70 , 71 . These developments have allowed for more sophisticated and efficient designs and further expanded the possibilities of structural topology optimization. As shown in Table 3 , the various topology optimization approaches have continuously evolved to improve their effectiveness and efficiency, which are introduced individually in this section.
After the introduction of the homogenization-based topology optimization method by Bendsoe and Kikuchi 64 and later developments by Bendsoe 72 , the SIMP method was proposed 73 , 74 . Sigmund 75 provided a clear explanation of the numerical implementation of the SIMP method in 2001 using a concise 99-line Matlab code. The SIMP method assumes constant material properties for the solid material within the design domain. The design variables in the optimization process are the relative densities of each element, which range between zero and one. The material properties are modeled as the relative material density raised to a power multiplied by the properties of the solid material. During the early 1990s, Xie and Steven initially put forth the Evolutionary Structural Optimization (ESO) method to attain optimal topologies for continuum structures 67 , 76 , 77 . Subsequently, Querin et al. 68 and Yang et al. 78 advanced the ESO method to develop the Bi-directional Evolutionary Structural Optimization (BESO) method. The level set-based topology optimization method utilizes a higher-dimensional embedded function to implicitly represent solid-void interfaces 79 , 80 . In the traditional level set method, the Hamilton-Jacobi equation (PDE) is solved using the velocity normal to the interface 71 , 81 , 82 . The zero-level contour of the embedded function in the conventional level set method defines the material boundary, serving as the partition between the solid and void domains.
Advanced structural topology optimization methods
In recent years, a variety of innovative optimization algorithms have emerged to tackle the practical challenges associated with flexible design domains, smooth material boundaries, and complex fabrication constraints. One such method is the Reaction diffusion-based level set (RDLS) approach, which was initially introduced in 2014 83 . The RDLS method enables the specification of geometrical complexities within the optimal configuration, thereby facilitating the identification of the desired structure shape through the evolution of the level set function. Another notable advancement is the Floating projection topology optimization (FPTO) method, which was unveiled in 2021 84 . FPTO ensures that design variables take discrete values, resulting in more robust and practical optimization outcomes. Lastly, the Node moving-based topology optimization (NMTO) method, introduced in 2023 85 utilizes a narrowband offset from the structural profile to establish a signed-distance function, which determines the direction of node movement. NMTO aims to optimize the structural topology and enhance its overall performance by manipulating node positions. These cutting-edge methods show great promise for advancing the capabilities of 3DCP and optimizing the production of high-performance structures.
Nowadays, structural topology optimization has become increasingly popular in various fields, including additive manufacturing 69 , 86 , architectural design 87 , 88 , biochemical 89 , 90 , and aerospace engineering 91 , 92 . Among them, the high design flexibility of 3DCP makes it compatible with topology optimization to decrease material usage and improve sustainability. With the integration of these approaches and 3DCP, it becomes possible to create intricate designs that are both structurally sound and resource-efficient.
To find an appropriate method for 3DCP, the benefits and limitations of each topology optimization method should be fully understood, which are introduced and summarized in this subsection. The key scientific differences between the various topology optimization methods include mathematical formulation, optimization algorithms, material models, sensitivity analysis, and post-processing techniques.
The advantages and disadvantages of these topology optimization methods can be concluded to judge whether they can be integrated with 3DCP to fabricate efficient and environmentally friendly structures. For instance, the homogenization method allows for accurate computation of material properties using a systematic approach to obtain optimal topology. However, it may not be suitable for structures with complex material distributions and may struggle with handling geometric complexities. The SIMP method is advantageous as it provides a simple and effective way to model material properties and incorporate manufacturing constraints. Nevertheless, it produces designs with intermediate densities and may suffer from numerical instabilities. Next, the ESO method offers improved utilization of material resources by gradually removing ineffective material but may require a large number of iterations and struggle with complex geometries. Similarly, the BESO method efficiently optimizes structures by employing fundamental strategies but may produce designs with checkerboard patterns and require careful parameter tuning. On the other hand, the conventional level set method utilizes higher-dimensional embedded functions to implicitly represent solid-void interfaces accurately, which can handle topological changes during the optimization process. Nonetheless, it requires careful handling of interface tracking to avoid spurious geometries and may suffer from numerical diffusion and grid-related issues.
On the other hand, the RDLS method allows for specifying geometrical complexity but requires significant computational resources. Besides, this method is sensitive to parameter settings. The FPTO method incorporates floating projection constraints and heuristically simulates 0/1 constraints of design variables, leading to discrete and practical solutions, that provide robust optimization results by considering upper and lower bounds. However, the method’s heuristic nature may not guarantee global optimality, and it may require careful tuning of parameters to balance feasibility and optimality. The NMTO method establishes a signed-distance function to determine node-moving directions, allowing for efficient topology optimization, complex structure design, and flexibility in node manipulation. The disadvantage of the NMTO method is that it may struggle with handling complex boundary conditions and geometric constraints. These are just some general advantages and disadvantages of the topology optimization methods mentioned.
In summary, the suitability of each method regarding 3DCP depends on specific applications and requirements. Different topology optimization methods employ various mathematical formulations to represent and solve the optimization problem. Each formulation has its advantages and limitations in terms of modeling flexibility, convergence behavior, and computational efficiency. Besides, topology optimization methods may differ in the sensitivity analysis approach employed to evaluate the influence of design changes on the objective function and constraints. After obtaining an optimized design, different methods employ various post-processing techniques to interpret and convert the obtained results into manufacturable forms. These techniques can include filtering, mesh smoothing, or shape reconstruction algorithms. The selection of post-processing techniques impacts the final quality, manufacturability, and practicality of the optimized design.
Structural topology optimization in 3D concrete printing
Structural topology optimization has been widely applied in the field of 3DCP, due to the benefits to create efficient and optimized structures. By combining these two techniques, engineers can maximize the use of material, reduce weight, and enhance load-bearing capabilities, resulting in more sustainable and cost-effective structures.
The emergence of 3DCP technology has revolutionized the field of structural design by providing unprecedented freedom in creating intricate geometries and customized structures 93 . This capability opens up new opportunities for designers to push the boundaries of traditional design principles 94 , 95 . By harnessing the inherent freedom of design, 3DCP can create structures that are aesthetically appealing and optimized for performance and functionality 87 . For instance, the optimization of material distribution in 3DCP is a vital research direction to minimize material waste and optimize structural efficiency 14 , 96 . Since the last decade, structural topology optimization has been increasingly applied in 3DCP 97 , 98 . Figure 4 shows the research article number in the last decade integrating different topology optimization approaches and 3DCP using the keywords “3D printed concrete”, “Homogenization method”, “SIMP method”, “ESO method”, “BESO method”, “Level set method”, and “Phase field method” based on data obtained from the Web of Science database. This section focuses on the approaches that have been explored to achieve structural topology optimization in 3DCP. These include using additive manufacturing techniques to build complex geometries and incorporating reinforcement elements during the printing process 14 , 86 , 99 . Existing works 96 , 97 have demonstrated the ability to optimize the internal structure of concrete components, resulting in improved mechanical properties and enhanced performance.
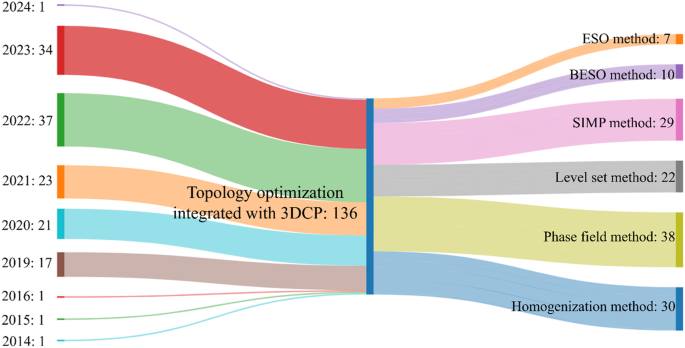
The literature study includes research on the application of six typical optimization methods from 2014 to 2024.
The integration of topology optimization and 3DCP has the potential to enhance the performance and resource efficiency of buildings. With the increasing emphasis on sustainable and eco-friendly practices, optimized structural design has emerged as a critical strategy to reduce material usage while maintaining structural strength 99 , 100 . For instance, the varying physical properties present in functionally graded materials can be customized to meet specific requirements, all while making efficient use of material resources 101 . Building on the multi-material BESO method, a novel approach to 3DCP structural design was introduced 102 . In this approach, 3DPC components primarily experience compression without the need for extra reinforcement. Instead, they synergistically collaborate with tensioned steel cables to create an effective composite structural system. The previous study 96 examined the production process of a topology-optimized 3D printed concrete bridge structure, highlighting its significant deviation from the manufacturing procedures of conventional concrete structures. Yang et al. 103 presented an integrated design method for 3DCP by incorporating extrusion-based manufacturing characteristics into the topology optimization algorithm. Lightweight structures tend to have better seismic performance, increased durability, and reduced energy consumption compared to their heavier counterparts 61 . In addition, lighter structures require less foundation support, resulting in cost savings during construction 104 . Since construction activities are responsible for a significant amount of carbon emissions, reducing the amount of material used can significantly decrease the carbon footprint of a building.
Several examples of a combination of topology optimization and waste materials have been achieved using additive manufacturing 105 , 106 . These technologies provide benefits including minimized waste materials, accelerated construction timelines, and the capacity to create distinctive designs with intricate details. In addition, they classify large-scale 3DCP technologies, emphasizing the importance of optimizing printing ink to enhance economic and environmental results by utilizing waste materials in 3DCP applications. The combination of topology optimization and waste materials offers numerous benefits. Firstly, it promotes sustainable design practices by utilizing recycled or waste materials, contributing to the circular economy and reducing waste. Secondly, it helps reduce costs as waste materials are often less expensive or even available for free compared to conventional materials. In addition, incorporating waste materials into the design improves resource efficiency by minimizing the need for extracting and processing new materials. Moreover, the unique properties of waste materials can enhance the performance of the optimized design, such as strength, durability, or lightweight. This combination also encourages innovation and creativity by exploring unconventional design solutions.
In summary, the integration of topology optimization and 3DCP can enhance the performance and resource efficiency of buildings. The impact of structural lightweighting on seismic performance, durability, and energy consumption makes it a compulsory consideration in achieving resource efficiency. In terms of future research directions, further advancements in structural topology optimization for 3DCP are anticipated. This includes developing advanced algorithms that can handle anisotropic, large-scale optimization problems and integrating multi-material printing capabilities. In addition, research efforts could focus on exploring the potential of bio-inspired design principles and incorporating functional requirements such as interlocking, thermal insulation, and acoustic performance into the optimization process.
Toolpath design and optimization in 3D concrete printing
Toolpath design is a critical aspect of 3DCP as it directly impacts the quality, efficiency, and structural integrity of the printed components. Firstly, toolpath design takes into account material-related problems, such as the flowability and workability of the concrete mixture. By carefully planning the toolpath, engineers can ensure that the material is properly deposited, minimizing problems, such as clogging or inconsistent layering. Toolpath design also addresses process-related concerns, such as the prevention of sagging or deformation during printing. Optimizing the toolpath by the integration of factors such as load-bearing capabilities, stress distribution, and reinforcement placement, can enhance the structural integrity of the printed components.
Toolpath planning determines the success of the 3DCP process. Toolpath design involves mapping out the trajectory and deposition strategy of the printing toolhead to ensure accurate material placement and optimal structural integrity 101 , 107 , 108 . By carefully coordinating the movement of the toolhead, designers can achieve precise layering, intricate geometries, improved sustainability, and desired material properties in the printed structure. Xia et al. 109 proposed an integrated design method to improve the mechanical performance and manufacturability of material extrusion structures according to the technical characteristics of material extrusion. The technical aspects of toolpath planning encompass various considerations, such as path optimization 110 , 111 , 112 , layer sequencing 113 , 114 , manufacturing constraints 14 , 115 , 116 , and support structure generation 86 , 117 , 118 .
Figure 5 illustrates the number of publications during the past decade related to the keywords “3D printed concrete”, “Extrusion-based toolpath design”, “Geometric toolpath design”, “Toolpath visualization”, “Manufacturing constraints”, “Topology optimization-based toolpath design”, “Sliced toolpath design”, and “Toolpath design efficiency/performance” based on data obtained from the Web of Science database. Path optimization algorithms aim to minimize print time, reduce material waste, and enhance printing efficiency by optimizing the toolhead’s movement trajectory. Layer sequencing determines the order in which layers are printed to ensure stability and prevent collapse during the printing process. Material flow control involves adjusting the printing parameters, such as nozzle speed and extrusion rate, to achieve consistent material deposition and avoid defects. Lastly, support structure generation ensures the stability of overhanging or complex geometries during printing.
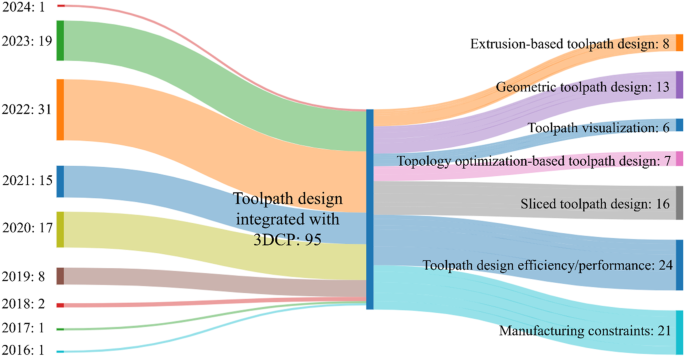
The literature study includes research on different toolpath design approaches from 2016 to 2024.
In recent years, there have been key research developments 14 , 15 , 96 , 119 in toolpath design and optimization. One of the key areas of focus has been on optimizing toolpaths for material efficiency and print time reduction. Researchers have explored various toolpath design methods with the instruction of topology optimization to achieve efficient and environmentally friendly structures. In addition, advancements in path optimization algorithms 110 , 111 , 112 , layer sequencing 113 , 114 , and support structure generation 86 , 117 , 118 have helped to enhance the printing efficiency and accuracy of 3DCP. Two novel printing techniques, “knitting” and “tilting” filaments, were proposed to address the anisotropy inherent in 3D printed ECC, emulating the natural crossed-lamellar structure of conch shells 120 . Three-dimensional spatial paths were devised to distribute tensile and flexural resistance in multiple directions and establish an interwoven interface system to enhance the strength of the structure.
The integration of toolpath design, 3D concrete printing, and topology optimization
Toolpath planning includes the strategic arrangement of the printing toolhead’s movement paths and deposition patterns to achieve the desired structural form 121 , 122 , 123 , 124 . This section aims to highlight the significance of toolpath planning in 3DCP and topology optimization. Existing methods for toolpath design in 3DCP involve a combination of computational algorithms, simulation techniques, and empirical knowledge. These methods consider various constraints and challenges, including printer limitations 14 , geometric complexity 16 , surface finish requirements 125 , overhang (self-support) problem 86 , interlocking 126 , and stability 127 during the printing process. They aim to generate toolpaths that maximize printing efficiency while ensuring the structural integrity and quality of the final product.
The toolpath design methods displayed in Fig. 5 can be integrated with 3DCP to fabricate efficient and high-performance structures depending on the fabrication requirements. Extrusion-based toolpath design in 3D concrete printing refers to the process of planning and creating the specific paths along which the extrusion nozzle will move to deposit layers of concrete material in a three-dimensional printed structure. Extrusion-based toolpath design 128 , 129 offers several advantages. It allows for the generation of toolpaths tailored to the specific material deposition process, resulting in efficient and optimized printing trajectories. By considering the extrusion process, this method can minimize print time, reduce material waste, and enhance printing efficiency. However, it may be limited in its ability to handle complex geometries and struggle with intricate support structure generation. Geometric toolpath design 16 , 130 focuses on creating toolpaths based on the geometric characteristics of the part being printed. This approach can lead to precise toolpaths that align with the part’s geometry, potentially reducing material waste. However, it may be less effective in optimizing toolpaths for overall printing efficiency and may struggle with handling complex layer sequencing. Toolpath visualization 131 , 132 provides a visual representation of the toolpaths, aiding in the identification of potential issues such as collisions, inefficient trajectories, or inadequate support structures. While it can help in identifying and addressing these issues, it may not actively optimize the toolpaths for print time, material waste, or printing efficiency. This method allows for precise control over layer sequencing and material flow control, ensuring stable and accurate printing. However, it may require additional computational resources and not fully optimize toolpaths for overall printing efficiency.
Toolpath design can be integrated with topology optimization to generate better performance 103 , 133 . Topology optimization-based toolpath design integrates the principles of topology optimization into the generation of toolpaths. By considering material deposition constraints and printing process dynamics, this method aims to create toolpaths that are not only geometrically optimized but also aligned with manufacturing constraints and support structure requirements. This approach can lead to highly efficient toolpaths that minimize print time, material waste, and enhance overall printing efficiency.
In summary, each of these toolpath design methods offers unique advantages and considerations. The selection of the most suitable method depends on the specific printing requirements, material characteristics, geometric complexity, and manufacturing constraints of the part being printed.
Benefits and challenges for future applications
The impact of toolpath optimization on the quality and efficiency of 3DCP has garnered significant attention. This section aims to analyze how optimized toolpaths positively influence printing quality and efficiency, emphasizing the reduction of waste and energy consumption. Advanced algorithms and computational models 119 , 127 , 134 are being developed to strategically plan the movement paths and deposition patterns of the printing toolhead, enabling precise material placement and optimized structural performance. A well-planned toolpath can result in structurally sound and aesthetically pleasing printed structures, while inadequate planning can lead to issues like material sagging, poor bonding between layers, or excessive material use 15 , 135 , 136 . Therefore, understanding and optimizing the toolpath planning process is vital for successful and reliable 3DCP 137 , 138 . Furthermore, toolpath planning also provides opportunities for customization and innovation in construction 16 , 125 . With the ability to precisely control the deposition pattern and material properties, designers can explore novel architectural forms, integrate functional features, and optimize performance characteristics.
Through systematic toolpath planning, it becomes possible to mitigate issues such as over-extrusion, uneven material distribution, and inaccuracies in layer deposition, ultimately leading to superior printing quality 112 , 139 . Moreover, the relationship between toolpath planning and material efficiency is paramount in the context of sustainable manufacturing practices. Optimized toolpaths contribute to the reduction of material waste and energy consumption by streamlining the printing process. Efficient toolpaths enable precise material deposition, minimize unnecessary movements, and optimize the use of support structures, thereby reducing material consumption and enhancing overall sustainability in 3DCP 132 , 140 , 141 .
The technical considerations involved in toolpath optimization for 3DCP encompass path optimization algorithms, print speed adjustments, and support structure generation. Path optimization algorithms aim to minimize print time and reduce material waste by optimizing the toolhead’s movement trajectory, while print speed adjustments ensure consistent material flow and deposition 132 . In addition, support structure generation and layer sequencing contribute to the stability and efficiency of the printing process 86 . Real-world case studies provide valuable insights into the benefits and challenges associated with toolpath optimization in construction projects 142 , 143 .
In terms of future research directions, there is a requirement to address additional constraints for the practical usage of 3DCP. For instance, the development of artificial intelligence empowered toolpath design methods for structures with complex geometric features. The integration of real-time monitoring and feedback systems into the toolpath design process can help improve accuracy and adaptability during printing. In addition, considering sustainability aspects, such as the use of recycled materials or minimizing waste, presents another avenue for future research in toolpath design for 3DCP.
Conclusions
This study presents a comprehensive overview of three vital aspects integrated with 3D concrete printing (3DCP) that contribute to enhancing sustainability in the construction sector. The first area of focus is sustainable material, which involves optimizing the constituents of printable materials through the recycling of waste materials into aggregates and supplementary cementitious materials. This approach reduces the environmental impact of the materials but also enhances the economic viability of 3DCP. The second vital area discussed is structural optimization, which plays a crucial role in maximizing structural performance and efficiency by rearranging material distribution. This optimization leads to improved structural integrity, reduced material usage, and minimized construction time and cost. Lastly, advances in toolpath planning have significantly improved the quality and efficiency of 3DCP. By strategically planning the movement paths and deposition patterns of the printing toolhead, toolpath optimization enhances printing accuracy, minimizes defects, and improves overall structural integrity. Furthermore, the review article also explores the influence of printing parameters on the quality and integrity of printed structures, providing valuable insights for future research and development in the field. By investigating the synergies between these three elements, this research aims to provide valuable insights for advancing sustainable and efficient building practices through the implementation of 3DCP technology.
The future of 3DCP in the construction sector is promising, while more systematic works are required to facilitate the practical application and sustainability of 3DCP:
Integration of Advanced Technologies: Future research should focus on integrating advanced technologies such as artificial intelligence and robotic control into toolpath optimization. These technologies can be adopted in the material design, system integration, and real-time optimization of printing processes.
Development of New Algorithms: There is a need for the development of new algorithms for toolpath optimization that can address specific challenges in 3DCP, such as handling complex geometries, optimizing material flow, and managing overhangs. These algorithms should also aim to optimize multiple objectives simultaneously.
Exploration of Novel Applications: Future research should explore novel applications of toolpath optimization in construction, such as printing complex architectural forms, integrating functional features, and creating customized structures. The potential of toolpath optimization in challenging environments, such as underwater or in space, should also be investigated.
Systematic literature review
This review article employs a systematic literature review approach based on established practices in additive manufacturing for construction to explore the intersections between 3DCP, material sustainability, structural topology optimization, and toolpath design. The Web of Science Core Collection, including indices such as SCI, SSCI, SCI-Expanded, and ESCI, is utilized to gather diverse publications until December 2023, encompassing journal articles, conference proceedings, books, and reports. A three-stage review method is meticulously designed to ensure objectivity and reproducibility.
Initially, relevant keywords, including “3D concrete printing,” “sustainable material,” “structural topology optimization,” and “toolpath design,” are defined to ensure a focused review. The literature reviews for sustainable material, TO, and toolpath design sections are conducted independently by different researchers. In the first stage, 1033 papers related to 3DCP are identified, with further breakdowns of 400 papers for sustainable material, 472 for structural topology optimization, and 161 for toolpath design. In the second stage, manual screening is conducted based on predefined criteria, including methodology robustness, published year, bibliographic information, and sustainability considerations. Comparative analysis results in the identification of 476 papers, comprising 245 for sustainable material, 136 for structural topology optimization, and 95 for toolpath design, as displayed in Figs. 3 , 4 , and 5 . In the third stage, the literature was further narrowed down to 160 references for inclusion in this review according to the specific criteria, including published journals, impact in the field, and number of citations. This three-step screening procedure guarantees that the literature review remains focused and relevant.
An analytical synthesis is then performed to summarize the primary studies of additive manufacturing in construction. The 160 studies obtained by the screening procedure are integrated systematically and classified into three sections according to their context, study design, and outcomes. The references cited in the sections on sustainable material, structural topology optimization, and toolpath design are 61, 76, and 23, respectively. In conclusion, the systematic literature review methodology minimizes reliance on subjective judgments, mitigates personal biases and errors, and upholds the integrity of scholarly research 144 .
Data availability
No datasets were generated or analyzed during the current study.
I.E. Agency. CO 2 Emissions in 2022 . https://www.iea.org/reports/co2-emissions-in-2022 (2023).
Enerdata. World Energy & Climate Statistics—Yearbook 2022 . World Energy Consumption Statistics| Enerdata France. https://yearbook.enerdata.net/total-energy/world-consumption-statistics.html (2022).
C.I. Council. Sustainable Construction . https://www.sc.cic.hk/index.php/en/ (2023).
Khan, S. A., Koç, M. & Al-Ghamdi, S. G. Sustainability assessment, potentials and challenges of 3D printed concrete structures: a systematic review for built environmental applications. J. Clean. Prod. 303 , 127027 (2021).
Article Google Scholar
Tay, Y. W. D. et al. 3D printing trends in building and construction industry: a review. Virtual Phys. Prototyp. 12 , 261–276 (2017).
Weng, Y. et al. Comparative economic, environmental and productivity assessment of a concrete bathroom unit fabricated through 3D printing and a precast approach. J. Clean. Prod. 261 , 121245 (2020).
Ngo, T. D. et al. Additive manufacturing (3D printing): a review of materials, methods, applications and challenges. Compos. Part B: Eng. 143 , 172–196 (2018).
Article CAS Google Scholar
Wangler, T. et al. Digital concrete: a review. Cem. Concr. Res. 123 , 105780 (2019).
Lu, B. et al. A systematical review of 3D printable cementitious materials. Constr. Build. Mater. 207 , 477–490 (2019).
Xiao, J. et al. Large-scale 3D printing concrete technology: current status and future opportunities. Cem. Concr. Compos. 122 , 104115 (2021).
Dey, D. et al. Use of industrial waste materials for 3D printing of sustainable concrete: a review. J. Clean. Prod. 340 , 130749 (2022).
Teng, F. et al. BIM-enabled collaborative-robots 3D concrete printing to construct MiC with reinforcement. HKIE Trans. 30 , 106–115 (2023).
Vantyghem, G., De Corte, W., Shakour, E. & Amir, O. 3D printing of a post-tensioned concrete girder designed by topology optimization. Autom. Constr. 112 , 103084 (2020).
Bi, M. et al. Topology optimization for 3D concrete printing with various manufacturing constraints. Addit. Manuf. 57 , 102982 (2022).
Google Scholar
Weng, Y. et al. Extracting BIM information for lattice toolpath planning in digital concrete printing with developed dynamo script: a case study. J. Comput. Civ. Eng. 35 , 05021001 (2021).
Breseghello, L. & Naboni, R. Toolpath-based design for 3D concrete printing of carbon-efficient architectural structures. Addit. Manuf. 56 , 102872 (2022).
CAS Google Scholar
Adaloudis, M. & Roca, J. B. Sustainability tradeoffs in the adoption of 3D Concrete Printing in the construction industry. J. Clean. Prod. 307 , 127201 (2021).
Tinoco, M. P. et al. Life cycle assessment (LCA) and environmental sustainability of cementitious materials for 3D concrete printing: a systematic literature review. J. Build. Eng. 52 , 104456 (2022).
Chen, Y. et al. A review of printing strategies, sustainable cementitious materials and characterization methods in the context of extrusion-based 3D concrete printing. J. Build. Eng. 45 , 103599 (2022).
Lu, B., Li, M., Wong, T. N. & Qian, S. Effect of printing parameters on material distribution in spray-based 3D concrete printing (S-3DCP). Autom. Constr. 124 , 103570 (2021).
Ford, S. & Despeisse, M. Additive manufacturing and sustainability: an exploratory study of the advantages and challenges. J. Clean. Prod. 137 , 1573–1587 (2016).
Liu, J. et al. 3D-printed concrete with recycled glass: effect of glass gradation on flexural strength and microstructure. Constr. Build. Mater. 314 , 125561 (2022).
Wu, Y. et al. Study on the rheology and buildability of 3D printed concrete with recycled coarse aggregates. J. Build. Eng. 42 , 103030 (2021).
Oosthuizen, J. D., Babafemi, A. J. & Walls, R. S. 3D-printed recycled plastic eco-aggregate (Resin8) concrete. Constr. Build. Mater. 408 , 133712 (2023).
Valente, M., Sambucci, M., Chougan, M. & Ghaffar, S. H. Composite alkali-activated materials with waste tire rubber designed for additive manufacturing: an eco-sustainable and energy saving approach. J. Mater. Res. Technol. 24 , 3098–3117 (2023).
Liu, C. et al. Influence of hydroxypropyl methylcellulose and silica fume on stability, rheological properties, and printability of 3D printing foam concrete. Cem. Concr. Compos. 122 , 104158 (2021).
Tinoco, M. Pimentel et al. The use of rice husk particles to adjust the rheological properties of 3D printable cementitious composites through water sorption. Constr. Build. Mater. 365 , 130046 (2023).
Ye, J. et al. Development of 3D printable engineered cementitious composites with incineration bottom ash (IBA) for sustainable and digital construction. J. Clean. Prod. 422 , 138639 (2023).
Ibrahim, K. A., van Zijl, G. P. A. G. & Babafemi, A. J. Influence of limestone calcined clay cement on properties of 3D printed concrete for sustainable construction. J. Build. Eng. 69 , 106186 (2023).
Chen, Y. et al. 3D printing of calcined clay-limestone-based cementitious materials. Cem. Concr. Res. 149 , 106553 (2021).
Qian, H. et al. Synergistic effect of EVA copolymer and sodium desulfurization ash on the printing performance of high volume blast furnace slag mixtures. Addit. Manuf. 46 , 102183 (2021).
Bhattacherjee, S. et al. Sustainable materials for 3D concrete printing. Cem. Concr. Compos. 122 , 104156 (2021).
Liu, J., Setunge, S. & Tran, P. 3D concrete printing with cement-coated recycled crumb rubber: compressive and microstructural properties. Constr. Build. Mater. 347 , 128507 (2022).
Ding, T., Xiao, J., Zou, S. & Wang, Y. Hardened properties of layered 3D printed concrete with recycled sand. Cem. Concr. Compos. 113 , 103724 (2020).
Liu, H. et al. Hardened properties of 3D printed concrete with recycled coarse aggregate. Cem. Concr. Res. 159 , 106868 (2022).
H.K.E.P. Department. Hong Kong Solid Waste Monitoring Report Waste Statistics 2022 (2022).
H.K.E.P. Department. Waste Blueprint for Hong Kong 2035 (2021).
Ahmed, G. H., Askandar, N. H. & Jumaa, G. B. A review of largescale 3DCP: material characteristics, mix design, printing process, and reinforcement strategies. Structures 43 , 508–532 (2022).
Christen, H., van Zijl, G. & de Villiers, W. The incorporation of recycled brick aggregate in 3D printed concrete. Clean. Mater. 4 , 100090 (2022).
Sambucci, M., Biblioteca, I. & Valente, M. Life Cycle Assessment (LCA) of 3D concrete printing and casting processes for cementitious materials incorporating ground waste tire rubber. Recycling 8 , 15 (2023).
Skibicki, S. et al. The effect of using recycled PET aggregates on mechanical and durability properties of 3D printed mortar. Constr. Build. Mater. 335 , 127443 (2022).
Weng, Y., Li, M., Tan, M. J. & Qian, S. Design 3D printing cementitious materials via Fuller Thompson theory and Marson-Percy model. Constr. Build. Mater. 163 , 600–610 (2018).
Nerella, V. N. et al. Inline quantification of extrudability of cementitious materials for digital construction. Cem. Concr. Compos. 95 , 260–270 (2019).
Wu, Y. et al. 3D printed concrete with recycled sand: pore structures and triaxial compression properties. Cem. Concr. Compos. 139 , 105048 (2023).
Ting, G. H. A., Tay, Y. W. D., Qian, Y. & Tan, M. J. Utilization of recycled glass for 3D concrete printing: rheological and mechanical properties. J. Mater. Cycles Waste Manag. 21 , 994–1003 (2019).
Zou, M. et al. Evaluation and control of printability and rheological properties of 3D-printed rubberized concrete. J. Build. Eng. 80 , 107988 (2023).
Ding, T., Xiao, J. & Tam, V. W. Y. A closed-loop life cycle assessment of recycled aggregate concrete utilization in China. Waste Manag. 56 , 367–375 (2016).
Article PubMed Google Scholar
Han, Y., Yang, Z., Ding, T. & Xiao, J. Environmental and economic assessment on 3D printed buildings with recycled concrete. J. Clean. Prod. 278 , 123884 (2021).
Shen, W. et al. Quantifying CO 2 emissions from China’s cement industry. Renew. Sustain. Energy Rev. 50 , 1004–1012 (2015).
Weng, Y. et al. Feasibility study on sustainable magnesium potassium phosphate cement paste for 3D printing. Constr. Build. Mater. 221 , 595–603 (2019).
Gardner, L. J. et al. Characterisation of magnesium potassium phosphate cements blended with fly ash and ground granulated blast furnace slag. Cem. Concr. Res. 74 , 78–87 (2015).
Tangstad, M. in Handbook of Ferroalloys (ed. Gasik, M.) 179–220 (Butterworth-Heinemann, 2013).
Lucen, H. et al. The synergistic effect of greenhouse gas CO 2 and silica fume on the properties of 3D printed mortar. Compos. Part B: Eng. 271 , 111188 (2024).
Chen, Y. et al. Limestone and calcined clay-based sustainable cementitious materials for 3D concrete printing: a fundamental study of extrudability and early-age strength development. Appl. Sci. 9 , 1809 (2019).
Weng, Y. et al. Empirical models to predict rheological properties of fiber reinforced cementitious composites for 3D printing. Constr. Build. Mater. 189 , 676–685 (2018).
Zhao, Y. et al. Development of low-carbon materials from GGBS and clay brick powder for 3D concrete printing. Constr. Build. Mater. 383 , 131232 (2023).
Xu, Z. et al. Effect of FA and GGBFS on compressive strength, rheology, and printing properties of cement-based 3D printing material. Constr. Build. Mater. 339 , 127685 (2022).
Rahul, A. V., Santhanam, M., Meena, H. & Ghani, Z. 3D printable concrete: Mixture design and test methods. Cem. Concr. Compos. 97 , 13–23 (2019).
Long, W.-J. et al. Printability and particle packing of 3D-printable limestone calcined clay cement composites. Constr. Build. Mater. 282 , 122647 (2021).
Yao, Y., Hu, M., Di Maio, F. & Cucurachi, S. Life cycle assessment of 3D printing geo‐polymer concrete: an ex‐ante study. J. Ind. Ecol. 24 , 116–127 (2020).
Liu, S. et al. A comparative study on environmental performance of 3D printing and conventional casting of concrete products with industrial wastes. Chemosphere 298 , 134310 (2022).
Article CAS PubMed Google Scholar
Gao, W. et al. The status, challenges, and future of additive manufacturing in engineering. Comput. Aided Des. 69 , 65–89 (2015).
Michell, A. G. M. The limits of economy of material in frame structures. Philos. Mag. 8 , 589–597 (1904).
Bendsoe, M. P. & Kikuchi, N. Generating optimal topologies in structural design using a homogenization method. Comput. Methods Appl. Mech. Eng. 71 , 197–224 (1988).
Lurie, K. A., Cherkaev, A. V. & Fedorov, A. V. Regularization of optimal design problems for bars and plates, part 1. J. Optim. Theory Appl. 37 , 499–522 (1982).
Suzuki, K. & Kikuchi, N. A homogenization method for shape and topology optimization. Comput. Methods Appl. Mech. Eng. 93 , 291–318 (1991).
Xie, Y. M. & Steven, G. P. A simple evolutionary procedure for structural optimization. Comput. Struct. 49 , 885–896 (1993).
Querin, O. M., Steven, G. P. & Xie, Y. M. Evolutionary structural optimisation (ESO) using a bidirectional algorithm. Eng. Comput. 15 , 1031–1048 (1998).
Zhuang, Z., Xie, Y. M., Li, Q. & Zhou, S. Body-fitted bi-directional evolutionary structural optimization using nonlinear diffusion regularization. Comput. Methods Appl. Mech. Eng. 396 , 115114 (2022).
Sethian, J. A. & Wiegmann, A. Structural boundary design via level set and immersed interface methods. J. Comput. Phys. 163 , 489–528 (2000).
Allaire, G., Jouve, F. & Toader, A.-M. A level set method for shape optimization. C. R. Math. 334 , 1125–1130 (2002).
Bendsoe, M. P. Optimal shape design as a material distribution problem. Struct. Optim. 1 , 193–202 (1989).
Sigmund, O. Materials with prescribed constitutive parameters: an inverse homogenization problem. Int. J. Solids Struct. 31 , 2313–2329 (1994).
Sigmund, O. On the design of compliant mechanisms using topology optimization. Mech. Struct. Mach. 25 , 493–524 (1997).
Sigmund, O. A 99 line topology optimization code written in Matlab. Struct. Multidiscip. Optim. 21 , 120–127 (2001).
Xie, Y. M. & Steven, G. P. Evolutionary Structural Optimization (Springer-Verlag, 1997).
Xie, Y. M. & Steven, G. P. Evolutionary structural optimization for dynamic problems. Comput. Struct. 58 , 1067–1073 (1996).
Yang, X. Y., Xie, Y. M., Steven, G. & Querin, O. Bidirectional evolutionary method for stiffness optimization. AIAA J. 37 , 1483–1488 (1999).
Osher, S. & Sethian, J. A. Fronts propagating with curvature-dependent speed: Algorithms based on Hamilton-Jacobi formulations. J. Comput. Phys. 79 , 12–49 (1988).
Vese, L. A. & Chan, T. F. A multiphase level set framework for image segmentation using the mumford and Shah model. Int. J. Comput. Vis. 50 , 271–293 (2002).
Wang, M. Y., Wang, X. & Guo, D. A level set method for structural topology optimization. Comput. Methods Appl. Mech. Eng. 192 , 227–246 (2003).
Wang, M. Y. & Wang, X. “Color” level sets: a multi-phase method for structural topology optimization with multiple materials. Comput. Methods Appl. Mech. Eng. 193 , 469–496 (2004).
Otomori, M., Yamada, T., Izui, K. & Nishiwaki, S. Matlab code for a level set-based topology optimization method using a reaction diffusion equation. Struct. Multidiscip. Optim. 51 , 1159–1172 (2014).
Huang, X. On smooth or 0/1 designs of the fixed-mesh element-based topology optimization. Adv. Eng. Softw. 151 , 102942 (2021).
Zhuang, Z. et al. A node moving-based structural topology optimization method in the body-fitted mesh. Comput. Methods Appl. Mech. Eng. 419 , 116663 (2024).
Bi, M., Tran, P. & Xie, Y. M. Topology optimization of 3D continuum structures under geometric self-supporting constraint. Addit. Manuf. 36 , 101422 (2020).
Xie, Y. M. Generalized topology optimization for architectural design. Architect. Intell. 1 , 1–11 (2022).
Liu, Y. et al. Reducing the number of different faces in free-form surface approximations through clustering and optimization. Comput. Aided Des. 166 , 103633 (2024).
Zhao, Z.-L., Zhou, S., Feng, X.-Q. & Xie, Y. M. Morphological optimization of scorpion telson. J. Mech. Phys. Solids 135 , 103773 (2020).
Cai, K., Chen, B. S. & Zhang, H. W. Topology optimization of continuum structures based on a new bionics method. Int. J. Computat. Methods Eng. Sci. Mech. 8 , 233–242 (2007).
Zhu, J., Zhang, W. & Xia, L. Topology optimization in aircraft and aerospace structures design. Arch. Comput. Methods Eng. 23 , 595–622 (2016).
Leader, M. K., Chin, T. W. & Kennedy, G. High resolution topology optimization of aerospace structures with stress and frequency constraints, In Proc. 2018 Multidisciplinary Analysis and Optimization Conference, American Institute of Aeronautics and Astronautics (2018).
Menna, C. et al. Opportunities and challenges for structural engineering of digitally fabricated concrete. Cem. Concr. Res. 133 , 106079 (2020).
Chen, Y., Zhou, C. & Lao, J. A layerless additive manufacturing process based on CNC accumulation. Rapid Prototyp. J. 17 , 218–227 (2011).
Pan, Y., Zhou, C., Chen, Y. & Partanen, J. Multi-tool and multi-axis CNC Accumulation for fabricating conformal features on curved surfaces. J. Manuf. Sci. Eng. 136 , 031007 (2014).
Ooms, T. et al. Third RILEM International Conference on Concrete and Digital Fabrication 37–42 (Springer International Publishing, 2022).
Asprone, D., Auricchio, F., Menna, C. & Mercuri, V. 3D printing of reinforced concrete elements: Technology and design approach. Constr. Build. Mater. 165 , 218–231 (2018).
Gebhard, L. et al. Structural behaviour of 3D printed concrete beams with various reinforcement strategies. Eng. Struct. 240 , 112380 (2021).
Martens, P., Mathot, M., Bos, F. & Coenders, J. High Tech Concrete: Where Technology and Engineering Meet 301–309 (Springer International Publishing, 2018).
Ahmed, G. H. A review of “3D concrete printing”: materials and process characterization, economic considerations and environmental sustainability. J. Build. Eng. 66 , 105863 (2023).
Tay, Y. W. D., Lim, J. H., Li, M. & Tan, M. J. Creating functionally graded concrete materials with varying 3D printing parameters. Virtual Phys. Prototyp. 17 , 662–681 (2022).
Li, Y. et al. FloatArch: a cable-supported, unreinforced, and re-assemblable 3D-printed concrete structure designed using multi-material topology optimization. Addit. Manuf. 81 , 104012 (2024).
Yang, W., Wang, L., Ma, G. & Feng, P. An integrated method of topological optimization and path design for 3D concrete printing. Eng. Struct. 291 , 116435 (2023).
Mechtcherine, V. et al. Extrusion-based additive manufacturing with cement-based materials—production steps, processes, and their underlying physics: A review. Cem. Concr. Res. 132 , 106037 (2020).
Tu, H. et al. Recent advancements and future trends in 3D concrete printing using waste materials. Dev. Built Environ. 16 , 100187 (2023).
Heywood, K. & Nicholas, P. Sustainability and 3D concrete printing: identifying a need for a more holistic approach to assessing environmental impacts. Architect. Intell. 2 , 12 (2023).
Wang, L., Jiang, H., Li, Z. & Ma, G. Mechanical behaviors of 3D printed lightweight concrete structure with hollow section. Arch. Civ. Mech. Eng. 20 , 16 (2020).
Geng, Z., Pan, H., Zuo, W. & She, W. Functionally graded lightweight cement-based composites with outstanding mechanical performances via additive manufacturing. Addit. Manuf. 56 , 102911 (2022).
Xia, L. et al. Integrated lightweight design method via structural optimization and path planning for material extrusion. Addit. Manuf. 62 , 103387 (2023).
Jin, Y.-a et al. Optimization of tool-path generation for material extrusion-based additive manufacturing technology. Addit. Manuf. 1-4 , 32–47 (2014).
Jin, Y. et al. An optimization approach for path planning of high-quality and uniform additive manufacturing. Int. J. Adv. Manuf. Technol. 92 , 651–662 (2017).
Ding, D., Pan, Z., Cuiuri, D. & Li, H. A practical path planning methodology for wire and arc additive manufacturing of thin-walled structures. Robot. Comput. Integr. Manuf. 34 , 8–19 (2015).
Sales, E., Kwok, T.-H. & Chen, Y. Function-aware slicing using principal stress line for toolpath planning in additive manufacturing. J. Manuf. Process. 64 , 1420–1433 (2021).
Chakraborty, D., Reddy, B. & Choudhury, A. Extruder path generation for Curved Layer Fused Deposition Modeling. Comput. Aided Des. 40 , 235–243 (2008).
Jiang, J. & Ma, Y. Path planning strategies to optimize accuracy, quality, build time and material use in additive manufacturing: a review. Micromachines 11 , 633 (2020).
Article PubMed PubMed Central Google Scholar
Giberti, H., Sbaglia, L. & Urgo, M. A path planning algorithm for industrial processes under velocity constraints with an application to additive manufacturing. J. Manuf. Syst. 43 , 160–167 (2017).
Jin, Y. et al. A non-retraction path planning approach for extrusion-based additive manufacturing. Robot. Comput. Integr. Manuf. 48 , 132–144 (2017).
Wang, T. et al. Load-dependent path planning method for 3D printing of continuous fiber reinforced plastics. Compos. Part A: Appl. Sci. Manuf. 140 , 106181 (2021).
Chen, X., Fang, G., Liao, W.-H. & Wang, C. C. L. Field-based toolpath generation for 3D printing continuous fibre reinforced thermoplastic composites. Addit. Manuf. 49 , 102470 (2022).
Zhou, W., McGee, W., Gökçe, H. S. & Li, V. C. A bio-inspired solution to alleviate anisotropy of 3D printed engineered cementitious composites (3DP-ECC): Knitting/tilting filaments. Autom. Constr. 155 , 105051 (2023).
Anton, A. et al. A 3D concrete printing prefabrication platform for bespoke columns. Autom. Constr. 122 , 103467 (2021).
Dörrie, R. et al. Automated force-flow-oriented reinforcement integration for Shotcrete 3D Printing. Autom. Constr. 155 , 105075 (2023).
Breseghello, L., Hajikarimian, H., Jørgensen, H. B. & Naboni, R. 3DLightBeam+. Design, simulation, and testing of carbon-efficient reinforced 3D concrete printed beams. Eng. Struct. 292 , 116511 (2023).
Moini, M. et al. Additive manufacturing and performance of architectured cement-based materials. Adv. Mater. 30 , 1802123 (2018).
Lin, Z. et al. Tool path generation for multi-axis freeform surface finishing with the LKH TSP solver. Comput. Aided Des. 69 , 51–61 (2015).
Zareiyan, B. & Khoshnevis, B. Effects of interlocking on interlayer adhesion and strength of structures in 3D printing of concrete. Autom. Constr. 83 , 212–221 (2017).
Bi, M. et al. Continuous contour-zigzag hybrid toolpath for large format additive manufacturing. Addit. Manuf. 55 , 102822 (2022).
Vispute, M., Kumar, N., Taufik, M. & Jain, P. K. Improving surface finish of extrusion based additive manufactured parts using novel triangle based toolpath approach. Int. J. Interact. Des. Manuf. 18 , 433–452 (2024).
Jensen, M. L. et al. Toolpath strategies for 5DOF and 6DOF extrusion-based additive manufacturing, Appl. Sci. 9 , 4168 (2019).
Li, C. L. A geometric approach to boundary-conformed toolpath generation. Comput. Aided Des. 39 , 941–952 (2007).
Breseghello, L. & Naboni, R. Adaptive toolpath: enhanced design and process control for robotic 3DCP. In International Conference on Computer-Aided Architectural Design Futures 301–316 (2022).
Liu, W., Chen, L., Mai, G. & Song, L. Toolpath planning for additive manufacturing using sliced model decomposition and metaheuristic algorithms. Adv. Eng. Softw. 149 , 102906 (2020).
Yang, W. et al. An integrated topology optimization method including manufacturing constraints for 3D printed fiber-reinforced concrete structures. Mater. Lett. 355 , 135442 (2024).
Lin, S. et al. A maze-like path generation scheme for fused deposition modeling. Int. J. Adv. Manuf. Technol. 104 , 1509–1519 (2019).
Jin, G. Q., Li, W. D., Gao, L. & Popplewell, K. A hybrid and adaptive tool-path generation approach of rapid prototyping and manufacturing for biomedical models. Comput. Ind. 64 , 336–349 (2013).
Moini, R. Perspectives in architected infrastructure materials. RILEM Tech. Lett. 8 , 125–140 (2024).
Xia, L., Lin, S. & Ma, G. Stress-based tool-path planning methodology for fused filament fabrication. Addit. Manuf. 32 , 101020 (2020).
Xia, L. et al. Globally continuous hybrid path for extrusion-based additive manufacturing. Autom. Constr. 137 , 104175 (2022).
Li, N. et al. Path-designed 3D printing for topological optimized continuous carbon fibre reinforced composite structures. Compos. Part B: Eng. 182 , 107612 (2020).
Liu, J., Ma, Y., Qureshi, A. J. & Ahmad, D. R. Light-weight shape and topology optimization with hybrid deposition path planning for FDM parts. Int. J. Adv. Manuf. Technol. 97 , 1123–1135 (2018).
Ye, J. et al. Feasibility of using ultra-high ductile concrete to print self-reinforced hollow structures. in Proc. Third RILEM International Conference on Concrete and Digital Fabrication 133–138 (2022).
Jin, G. Q., Li, W. D., Tsai, C. F. & Wang, L. Adaptive tool-path generation of rapid prototyping for complex product models. J. Manuf. Syst. 30 , 154–164 (2011).
Jin, Y.-A., He, Y., Xue, G.-H. & Fu, J.-Z. A parallel-based path generation method for fused deposition modeling. Int. J. Adv. Manuf. Technol. 77 , 927–937 (2015).
Roberts, H. & Petticrew, M. Systematic Reviews in the Social Sciences: A Practical Guide (Wiley, 2006).
Glowinski, R. Trends and Applications of Pure Mathematics to Mechanics 96–145 (Springer Berlin Heidelberg, 1984).
Kikuchi, N., Chung, K. Y., Torigaki, T. & Taylor, J. E. Computer Methods in Applied Mechanics and Engineering 139–169 (1986).
Andreassen, E. et al. Efficient topology optimization in MATLAB using 88 lines of code. Struct. Multidiscip. Optim. 43 , 1–16 (2011).
Ferrari, F. & Sigmund, O. A new generation 99 line Matlab code for compliance topology optimization and its extension to 3D. Struct. Multidiscip. Optim. 62 , 2211–2228 (2020).
Rozvany, G. I. N., Zhou, M. & Birker, T. Generalized shape optimization without homogenization. Struct. Optim. 4 , 250–252 (1992).
Huang, X. & Xie, Y. M. Evolutionary Topology Optimization of Continuum Structures: Methods and Applications (Wiley, 2010).
Zuo, Z. H. & Xie, Y. M. A simple and compact Python code for complex 3D topology optimization. Adv. Eng. Softw. 85 , 1–11 (2015).
Huang, X., Xie, Y. M. & Burry, M. C. A new algorithm for bi-directional evolutionary structural optimization. JSME Int. J. Ser. C. Mech. Syst., Mach. Elem. Manuf. 49 , 1091–1099 (2006).
Challis, V. J. A discrete level-set topology optimization code written in Matlab. Struct. Multidiscip. Optim. 41 , 453–464 (2010).
Allaire, G., Jouve, F. & Toader, A.-M. Structural optimization using sensitivity analysis and a level-set method. J. Comput. Phys. 194 , 363–393 (2004).
Zhuang, Z., Xie, Y. M., Li, Q. & Zhou, S. A 172-line Matlab code for structural topology optimization in the body-fitted mesh. Struct. Multidiscip. Optim. 66 , 11 (2022).
Yamada, T., Izui, K., Nishiwaki, S. & Takezawa, A. A topology optimization method based on the level set method incorporating a fictitious interface energy. Comput. Methods Appl. Mech. Eng. 199 , 2876–2891 (2010).
Li, H. et al. Full-scale 3D structural topology optimization using adaptive mesh refinement based on the level-set method. Finite Elem. Anal. Des. 194 , 103561 (2021).
Zhuang, Z., Xie, Y. M. & Zhou, S. A reaction diffusion-based level set method using body-fitted mesh for structural topology optimization. Comput. Methods Appl. Mech. Eng. 381 , 113829 (2021).
Huang, X. A Matlab code of topology optimization by imposing the implicit floating projection constraint. (2022).
Huang, X. & Li, W. Three-field floating projection topology optimization of continuum structures. Comput. Methods Appl. Mech. Eng. 399 , 115444 (2022).
Zhang, X. et al. A nodal-based optimization method for the design of continuous fiber-reinforced structures. Compos. Struct. 323 , 117455 (2023).
Download references
Acknowledgements
This project was funded by National Science and Foundation of China (52308284), Department of Science and Technology of Guangdong Province (306071352047), and Hong Kong Polytechnic University (P0038598, P0038966, P0044299, P0045796). The funder played no role in study design, data collection, analysis and interpretation of data, or the writing of this manuscript.
Author information
These authors contributed equally: Zicheng Zhuang, Fengming Xu, Junhong Ye.
Authors and Affiliations
Department of Building and Real Estate, The Hong Kong Polytechnic University, Hong Kong, China
Zicheng Zhuang, Fengming Xu, Junhong Ye & Yiwei Weng
Department of Civil Engineering and Transportation, South China University of Technology, Hong Kong, China
Department of Building Environment and Energy Engineering, The Hong Kong Polytechnic University, Hong Kong, China
Liming Jiang
You can also search for this author in PubMed Google Scholar
Contributions
Z.Z., M.X., and J.Y. conducted the review and wrote the manuscript. H.N., L.J., and Y.W. made suggestions and revised the manuscript. Y.W. provided the resources and supervision. All authors read and approved the final manuscript.
Corresponding author
Correspondence to Yiwei Weng .
Ethics declarations
Competing interests.
The authors declare no competing interests.
Additional information
Publisher’s note Springer Nature remains neutral with regard to jurisdictional claims in published maps and institutional affiliations.
Rights and permissions
Open Access This article is licensed under a Creative Commons Attribution 4.0 International License, which permits use, sharing, adaptation, distribution and reproduction in any medium or format, as long as you give appropriate credit to the original author(s) and the source, provide a link to the Creative Commons licence, and indicate if changes were made. The images or other third party material in this article are included in the article’s Creative Commons licence, unless indicated otherwise in a credit line to the material. If material is not included in the article’s Creative Commons licence and your intended use is not permitted by statutory regulation or exceeds the permitted use, you will need to obtain permission directly from the copyright holder. To view a copy of this licence, visit http://creativecommons.org/licenses/by/4.0/ .
Reprints and permissions
About this article
Cite this article.
Zhuang, Z., Xu, F., Ye, J. et al. A comprehensive review of sustainable materials and toolpath optimization in 3D concrete printing. npj Mater. Sustain. 2 , 12 (2024). https://doi.org/10.1038/s44296-024-00017-9
Download citation
Received : 30 December 2023
Accepted : 02 March 2024
Published : 03 June 2024
DOI : https://doi.org/10.1038/s44296-024-00017-9
Share this article
Anyone you share the following link with will be able to read this content:
Sorry, a shareable link is not currently available for this article.
Provided by the Springer Nature SharedIt content-sharing initiative
Quick links
- Explore articles by subject
- Guide to authors
- Editorial policies
Sign up for the Nature Briefing: Anthropocene newsletter — what matters in anthropocene research, free to your inbox weekly.


IMAGES
VIDEO
COMMENTS
Abstract. 3D printing, unlike other manufacturing processes, being an additive process has emerged as a viable technology for the production of engineering components. The aspects associated with 3D printing such as less material wastage, ease of manufacturing, less human involvement, very less post processing and energy efficiency makes the ...
A Literature Review on 3D Printing Technologies in Teaching and Education. ... it is thought that it will be more effective to focus on the instructional aspect of 3D printing technology.
Advances in 3D printing technology can significantly change and improve the manufacturing world with effects on energy use, waste reduction, customization, product availability, medicine, art, construction and science. ... It is followed by far by experimental research, with 22.6%. Literature review, action research, modelling and simulation ...
Abstract This paper provides a critical review of the related literature on 3D printing in construction. The paper discusses and evaluates the different 3D printing techniques in construction. The paper also discusses and categorizes the benefits, challenges, and risks of 3D printing in construction. The use of 3D printing technology offers several advantages over traditional methods. However ...
Traditional manufacturing methods have become obsolete nowadays with the evolution of 3D printing. 3D printing has provided a totally new paradigm to modern-day manufacturing, and has had huge economic, environmental, scientific, and political implications. The paper describes the copious methodologies and applied 3D printing in several fields such as medicine, fashion industry, automobiles ...
This research aims to conduct a comprehensive systematic literature review (SLR) on the impact of state-of-the-art 3DP technology on architectural design and construction. ... The Role of 3D Printing Technology in Landscape Architecture Teaching and Learning Practices. Educ. Sci. Theory Pract. 2021, 21, 13-26. [Google Scholar] Bedarf, P ...
3D printing is an emerging educational technology that is said to prepare learners for a more technologically designed world. In this review, 3D printing studies are analyzed to identify the dominant theoretical approaches and learning outcomes associated with 3D printing in education. Five theories are identified, including situated learning (Lave & Wenger, 1991), experiential learning (Kolb ...
Although 3D printing (3DP) technology has become an increasingly popular educational tool in recent years, very little is known about the learning benefits of this technology. This systematic literature review synthesized empirical research on. Although 3D printing (3DP) technology has become an increasingly popular educational tool in recent ...
3D printing in education: a literature review Version 1.0 - 17 September 2016 Simon Ford 1,2* and Tim Minshall 1
Findings. This paper critically evaluates the current 3D printing technologies, including the development and optimizations made to the printing methods, as well as the printed objects. Meanwhile, previous developments in this area and contributions to the modern trend in manufacturing technology are summarized briefly.
This review covers a wide range of topics related to the use of 3D printing in manufacturing. As 3D printing technology enters the production sector, it has numerous advantages for the people, businesses, and the government alike. As a result, more research into effective strategies for increasing the uptake of 3D printing technology is required.
Polymer 3D printing is an emerging technology with recent research translating towards increased use in industry, particularly in medical fields. Polymer printing is advantageous because it enables printing low-cost functional parts with diverse properties and capabilities. Here, we provide a review of recent research advances for polymer 3D ...
The 3D printing technology has the advantages of faster production of dentures, and there are fewer stages in the work process, which can reduce the possibility of errors ... Lo L. J. 3D printing in orthognathic surgery − a literature review. Journal of the Formosan Medical Association. 2018; 117 (7):547-558. doi: 10.1016/j.jfma.2018.01.008.
1. Introduction. From aerospace and automotive to consumer goods, 3D printing (3DP) is embraced as process innovation (Steenhuis et al., 2020) across multiple industries to accelerate new product development, optimize supply chains and enable creative freedom.Still, the technology's rate of adoption is low when compared to initial forecasts (Gartner, 2019; Wohlers Associates, 2019).
3D printing in education: a literature review. Version 1.0 - 17 September 2016. Simon Ford 1,2* and Tim Minshall 1. 1 Institute for Manufacturing, University of Cambridge, 17 Charles Babbage ...
3D printing is a constantly expanding technology that represents one of the most exciting and disruptive production possibilities available today. This technology has gained global recognition and garnered considerable attention in recent years. However, technological breakthroughs, particularly in the field of material science, continue to be the focus of research, particularly in terms of ...
3D printing technologies (3DP) leverage the benefits of additive manufacturing across many areas including electronics, food, medicine and optics. These technologies allow varying materials to be precision deposited, forming structures ranging from simple to complex composites such as organs and satellites. One important application for 3DP is printed electronics which is expected to exceed ...
on the instructional aspect of 3D printing technology. Keywords: 3D printing technology, Education, K-12, Graduate students, Literature Review. 1. INTRODUCTION Technology is now advancing quickly. There have been advancements in numerous sectors owing to Industry 4.0. One of the most significant places where these improvements
The 3D printing technology can reduce the waste generated during construction, thus making the process more sustainable. Traditional construction methods often result in a significant amount of waste, as materials are cut to size and assembled on-site [9], [10], [11].In contrast, 3D printing technology can reduce waste by producing building components with greater precision, using only the ...
1. Introduction. Prosthesis design can be dated back to the ancient Egyptian and Roman empires and has continued to develop across the world throughout the course of history [1,2].In the late 1800s, John Hanger's prosthesis, the Hanger Limb, was developed in response to the American Civil War [], ushering prosthesis design into the modern era.. Medical advancements since the invention of the ...
The primary recycled materials in 3D printed concrete for sustainability enhancement include sand 34, glass 22, concrete 35, plastics 24, and rubber 25.According to the data from the Hong Kong ...
1. Introduction. 3D printing construction technology was an amazing breakthrough in the past decade and potentially can revolutionize the construction industry, resolve residential crises, serve the green house, and brings in sustainability benefits, such as increased resource efficiency, improved construction productivity, compensates for shortages of skilled labors, and building of complex ...