
An official website of the United States government
The .gov means it’s official. Federal government websites often end in .gov or .mil. Before sharing sensitive information, make sure you’re on a federal government site.
The site is secure. The https:// ensures that you are connecting to the official website and that any information you provide is encrypted and transmitted securely.
- Publications
- Account settings
Preview improvements coming to the PMC website in October 2024. Learn More or Try it out now .
- Advanced Search
- Journal List
- Int J Environ Res Public Health


The Public Distribution System and Food Security in India
The Public Distribution System (PDS) of India plays a crucial role in reducing food insecurity by acting as a safety net by distributing essentials at a subsidised rate. While the PDS forms a cornerstone of government food and nutrition policy, India continues to be home to a large population of hungry and malnourished people. This review seeks to explore the functioning and efficiency of the PDS in achieving food and nutritional security in India. A comprehensive and systematic search using the key terms “food insecurity” OR “food security” AND “Public Distribution System” OR “PDS” OR “TPDS” AND “India” identified 23 articles which met the inclusion criteria. This review draws attention to the lack of published literature in areas of PDS and food security in India. The findings of the review emphasise the role of PDS in tackling hunger and malnutrition while highlighting its limited role in improving food security and childhood mortality due to operational inefficiencies. The PDS has the potential to act as a solution to food insecurity in India if the operational inefficiencies and environmental footprints are addressed by adequate policy reforms.
1. Introduction
Food insecurity is a situation of limited access to safe and healthy food [ 1 ], while food security refers to a situation when ‘all people, at all times, have physical, social, and economic access to sufficient, safe and nutritious food that meets their dietary needs and food preferences for an active and healthy life’ [ 2 ]. Food insecurity can be understood as a continuum that progresses from uncertainty and anxiety about access to sufficient and appropriate food at the household level, to the extreme condition of hunger among children because they do not have enough to eat [ 3 ]. The experience of food insecurity has been found to be more severe in low-income communities, and for those who already experience poor health [ 4 , 5 ]. While factors contributing to poverty are important when considering food insecurity, it is not the only determinant. Other influences include policy, the distribution of food across populations, countries, and regions, unstable political conditions, and climate change induced adverse environmental conditions including severe droughts, lack of water, and soil degradation and erosion [ 1 , 6 , 7 , 8 , 9 ].
2. Food Security in India
While overall global rates of food insecurity have decreased, there remains a large population of people experiencing food insecurity living in sub-Saharan African and South Asia. Countries most affected are typically characterised by high rates of disease and mortality associated with nutritional deficiencies coupled with high rates of poverty [ 10 , 11 , 12 ]. Despite rapid economic growth over the last two decades, many Indians have not benefited from the economic improvement, and continue to experience food insecurity and hunger, a high burden of malnutrition and undernourishment, and increasing obesity [ 13 ]; in 2016, over 190 million people were reported undernourished—the highest in any single country [ 14 ].
The reasons behind food insecurity and hunger in India are complex. Some research indicates that high rates of migration from rural to urban areas may play a role [ 15 ], as evidenced by the concentration of economic gains in urban areas, and the significant changes to the contribution of the agriculture sector to GDP [ 16 ]. The adverse effects of climate change are also an emerging contributor to food insecurity, with uneven weather patterns and increasing droughts to blame for uneven growth and production of food stock [ 5 ]. The most recent Global Hunger Index (GHI) ranks India at the high end of the ‘serious’ category, as India continues to perform poorly in addressing hunger and malnutrition; currently one in three Indian children is stunted representing one third of the world’s stunted population, and one in five is wasted [ 17 ]. Many in India also experiences hidden hunger. Hidden hunger refers to a situation of chronic micronutrient deficiency, where a person might have access to sufficient calories, but lacks adequate micronutrients [ 18 ]. Hidden hunger can have lasting effects on health and wellbeing, and is especially problematic for children [ 19 ].
The government of India have adopted a range of policies over the last 60 years in an attempt to strengthen food security [ 5 ]. One of the key responses to food insecurity and hunger in India is the distribution of food grains through the government controlled Public Distribution System (PDS) [ 20 ]. Established after World War Two with an aim of increasing domestic agricultural production and improving food security, the PDS has evolved to the largest universal distribution system in the world for the supply of subsidised food grains [ 20 , 21 ]. Through a partnership between central and state governments, the food-safety net program aims to supplement essential household supplies including wheat, rice, sugar, and kerosene. However, like other solutions to food scarcity, this program is not intended to provide all required household foods, but rather provide goods to supplement purchased or farmed goods [ 20 , 22 ]. To facilitate the distribution, the Food Corporation of India (FCI) acts a central nodal agency responsible for the procurement of food grains from farmers at a price that is often higher than market price [ 20 , 23 ]. The individual state governments then procure the food grains at a subsidised price known as the ‘central issue price’ from the FCI [ 20 , 24 ], these goods are then distributed to consumers via fair price or ration shops [ 20 ].
Amendments made to the PDS in June 1992 sought to improve coverage, especially to those living in disadvantaged, remote, or difficult to access areas [ 20 ]. The PDS was re-structured again in June 1997, to better target lower socio-economic areas [ 20 ]. This Targeted Public Distribution System (TPDS) aimed to provide over seven million tonnes of foods to 60 million households identified as below the poverty line [ 20 ]. This was followed by the introduction (in December 2000 and expansion in 2003–2006) of the Antyodaya Anna Yojana (a Hindi term meaning “grain scheme for the downtrodden” [ 25 ]) scheme to provide highly subsidised foods to India’s poor. The scheme was an attempt to streamline the PDS to more efficiently target the poorest of the poor. This expansion of the PDS also included provision of food and goods to senior citizens and pensioners over 60 years, as well as widows and people affected by disease or infirmity [ 20 ].
These measures to strengthen the PDS received statutory backing through the introduction of the National Food Security Act (NFSA) [ 20 , 26 , 27 ]. Through the adoption of a life cycle approach, the importance of food security was emphasised by the NFSA. Through its implementation, the PDS achieved 75% coverage of the rural population, and around half of the urban population, monetary and nutritional support was mandated to pregnant and lactating women, and through the Integrated Child Development Services and Mid-Day Meal Schemes, children aged 6 months to 14 years were also covered [ 20 , 26 , 28 , 29 , 30 ]. The NFSA marked an important milestone in that it awarded India’s food safety network a legal standing in accordance with the fundamental right to good health and nutritious food [ 20 , 26 , 31 ]. The NSFA also went some way to address the challenges faced by the TPDS in the form of corruption and diversion by enforcing more accountability on stakeholders while improving the transparency of its operation [ 21 , 28 ].
While a number of studies have identified the role of the PDS and NFSA in improving the food security of India, at least through the provision of calories [ 32 , 33 ], there is some concern relating to issues of miss-targeting, under-coverage, corruption and diversion affecting the implementation and operation of the food safety network in India [ 13 , 26 , 34 ]. Understanding these concerns is challenging, as while a number of non-government organisations and government bodies provide reports on the quantity of food distributed [ 35 ], and the number of people reached through the PDS [ 36 , 37 ], there is little analysis or evidence surrounding the broader issues related to food insecurity and the PDS. Furthermore, these reports rarely provide the method for data collection and/or analysis, making further interpretation difficult. This current review seeks to bring together the published literature on the PDS, in order to investigate the role it plays in addressing food insecurity in India. This review seeks to understand this large and expensive food distribution system and its role in one of the most populist, but inequitable countries. This is the first review of its kind of this program, with the objective of providing a clear overview of current knowledge in this area. By investigating the available peer-reviewed literature, this review seeks to understand the role of the PDS in any attempt to achieve food security in India.
Specifically, there are two main objectives to this review:
- To investigate the role of the PDS in delivering an efficient food-safety network in India;
- To investigate the barriers and enablers of the PDS.
3. Method and Approach
A systematic literature search was conducted in November 2018 to identify articles that investigated the role of the PDS in responding to food insecurity in India. Databases included; Academic Search Complete, CINAHL Complete, EconLit, Global Health, GreenFILE, Health Policy Reference Center, Legal Source, Scopus, and Medline. Search terms included “food insecurity” OR “food security” AND “Public Distribution System” OR “PDS” OR “TPDS” AND “India”. Limits restricted the search to those articles with full text published in English. In order to gain a comprehensive understanding of the role of the PDS in achieving food security over time, no temporal limitations were placed on articles.
Articles were included in this review if they (1) included food security or food insecurity as an exposure of interest; (2) investigated the role of the PDS in addressing food security; and (3) were peer-reviewed. Editorials or commentaries [ 34 , 38 , 39 , 40 , 41 ] were excluded. Both authors reviewed all articles to identify relevancy. Articles were first screened by title and abstract based on the inclusion criteria. The full text of selected articles was obtained for assessment for final inclusion.
The database search utilising the key words identified 457 articles, of which 35 were duplicates. The titles and abstracts of the remaining 422 articles were reviewed to determine eligibility. Of these 334 articles were rejected based on the title or abstract, the full text of the remaining 88 articles was reviewed, leaving 23 that met the inclusion criteria ( Figure 1 ).

Flow chart showing article selection.
4.1. Study Characteristics
Reflecting recent interest in issues of the role of the PDS in addressing food insecurity, most articles ( n = 19) were published between 2010 and 2018. While some ( n = 11) articles reported on data collected by the National Sample Survey (NSS), India’s nation-wide household survey conducted on various socio-economic issues, only three articles reported on data that was collected at a national level [ 42 , 43 , 44 ]. The remaining studies focused on single states or a group of states, the most included states were Chhattisgarh, Andhra Pradesh, and Uttar Pradesh (see Table 1 ).
Summary of included studies.
Four studies employed mixed methods [ 45 , 46 , 47 , 48 ]. These studies employed a variety of methods including household interviews, and interviews with individual household members, surveys and interviews with PDS beneficiaries, and interviews with shop owners. Three studies were qualitative; Chopra, et al. [ 49 ] conducted interviews with 98 key informants including rice millers, production workers and shop owners; Dar [ 50 ] conducted interviews with 266 households in Kashmir to investigate a range of issues related to food access and entitlement; while Panigrahi and Pathak [ 51 ] conducted 50 interviews with above poverty line (APL) households and 50 interviews with below poverty line (BPL) households in Odisha to better understand their experiences with the PDS. All the remaining studies employed quantitative methods. Studies that focused on households ranged in participant household numbers from 50 [ 51 ] to more than half a million [ 44 ], while studies focused on individuals ranged in participant numbers from 98 [ 49 ] to 7124 [ 45 ].
4.2. Effectiveness of the PDS
Eight articles specifically examined the effectiveness of the PDS [ 45 , 46 , 47 , 49 , 53 , 54 , 56 , 58 ]. Each of these studies suggested that the PDS was not working effectively, with large amounts of food not reaching the intended recipients, and significant wastage resulting in high costs for limited benefits. For example, Dhanaraj and Gade [ 53 ] estimated that in Tamil Nadu, for every 5.43 kgs of PDS rice distributed by the government, only 1 kg reached those in need; the distribution was less efficient in the case of sugar, where only 1 kg for each 8.21 kgs distributed was consumed by those in need. Kumar [ 58 ], in a large investigation spanning 12 states, found that up to 100% of wheat was diverted in some cases, with diversion and provision of rice and wheat being different across all states. Khera [ 54 ], suggested that households cannot access their full entitlement to goods, and as a result are forced to purchase much of their food from the free market. Conversely, a positive trend was identified in the state of Bihar, where in 1993, 90% of food grains were diverted away from those in need; by 2001 this figure was down to just 12.5% of diverted food grain [ 56 ]. Similar findings were reported by Nair [ 61 ] in Kerala. In both states, this was attributed to better transparency and infrastructure.
4.3. Barriers and Enablers to the Efficient Working of the PDS
A number of barriers and enablers influencing the efficient working of the PDS were investigated across the studies included in this review. One key barrier to a more efficient system was the presence of illegal (or ghost) cards, with the finding that some households hold multiple cards [ 51 , 58 ]. The illegal cards were identified in several states, with Kumar [ 58 ] suggesting that there were approximately 230 million excess cards across the country in 2006.
Despite a number of significant, system-wide changes over recent years, high levels of corruption and leakage continue to plague the PDS [ 48 , 53 , 54 ]. Part of this leakage occurs at the level of the fair price shops, where Gupta and Singh [ 48 ] reported that some store owners exchanged the high quality goods provided from the government for distribution through the PDS with lesser quality goods from the general stores. Both Khera [ 54 ] and Dhanaraj and Gade [ 53 ] reported very high rates of corruption within the system, in some states this was up to 100% leakage or ‘diversion’ from the supply chain. Transparency, better governance, technology and the introduction of computerisation, along with use of global positioning system and distribution via doorstep delivery have been suggested as potential ways to address these issues [ 45 , 56 ].
Targeting errors, specifically the problems associated with targeting BPL and APL households were identified as areas where efficiencies could be made [ 45 , 52 , 58 ]. These studies suggest that while there was some effort to target the BPL households, the targeting has had a marginal effect on poor households [ 52 ]. There is also a suggestion from Kumar [ 58 ] that non-poor households have been included in the PDS to the detriment of the system. This is consistent with the work of Nair [ 61 ], who suggest that better targeting and the removal of APL households, that is, a removal of universal nature of the system, would have significant positive impacts on the operation and effects of the PDS.
4.4. Food Security in India—A Concept Map
In attempting to dissect the various areas that affect the effectiveness of the PDS and incorporate the involvement of multiple interrelated factors in addressing food security in India, a concept map was created ( Figure 2 ). In the conceptual representation of the factors that determine the national food security, blue arrows signify those factors that can be influenced by policy changes. The red arrows and negative polarity indicate elements that act as barriers while the green arrows and positive polarity specify variables that act as enablers.

A concept of the various determinants that affect effectiveness of the PDS and NFSA in addressing the food security in India. NFSA: National Food Security Act; PDS: Public Distribution System.
As shown in Figure 2 , there are multiple cross-links between the domains influencing food insecurity; the complex nature of the problem means that there is no one single overarching solution. One of the main responses to food insecurity in India, the PDS, is riddled with problems of targeting, diversion, and corruption [ 45 , 54 , 56 ]. As shown here, increased corruption decreases the accessibility which in turn affects the overall functioning of the PDS [ 54 , 56 ]. Despite the existence of multiple barriers, the PDS can be fortified if the enablers identified here are concentrated on. Efficient and well monitored administration was observed as a conclusive answer to not just problems with targeting but is also an effective solution for diversion [ 21 , 45 , 54 , 56 ]. Subsidised rates for commodities and an efficient transport system can also be drivers of success [ 21 , 45 ], as can an efficient food chain system that fosters greater inclusivity while reducing transaction costs [ 21 , 45 ]. Finally, increased wages in the agricultural sector were also recognised as a facilitating factor for the functioning of the PDS and agriculture in India [ 45 , 64 ].
The NFSA also plays a key role in responding to food insecurity. Having been a positive reinforcement for the PDS, it has the capacity to boost agriculture and reduce hunger and malnutrition by enhancing economic growth [ 65 ]. However, the NFSA fails to support environmental sustainability which is vital for the economy and long-term sustenance of the agricultural sector [ 64 , 65 ]. As shown in Figure 2 , the NFSA is a double-edged sword which requires careful monitoring and modifications in order to achieve its full potential [ 65 ].
While each determinant present in the concept map is vital for safeguarding food security in India, the key driver that influences most of the factors is policy change. Policy amendments have the capacity to establish food security within the country by regulating the barriers and enablers that affect the operational efficiency of the PDS [ 21 , 45 , 54 , 56 ]. Positive steps towards the eradication of hunger, malnutrition, childhood mortality, and environmental sustainability can be attained with effective policies [ 44 , 66 ]. The economy of a country, including the pricing strategies, is also influenced by policy reforms and thus affects subsidised rates which are vital for the effective penetration of the PDS [ 21 ]. The concept map thus helps to understand that policy changes coupled with reforms in PDS and NFSA are crucial for India to achieve food security for its inhabitants.
5. Discussion
This review examined 23 studies to investigate the role of the PDS in addressing food insecurity in India. The key finding of this review is that, while the PDS has been strengthened over recent years, particularly through efforts to target those most in need, more work remains, particularly around transparency and accountability [ 67 , 68 , 69 , 70 ].
5.1. Failure of PDS
The PDS is the largest food distribution network in the world [ 20 , 71 , 72 ], and its effective and efficient functioning is an essential component of any response to food insecurity. However, inefficiencies, miss-targeting, and corruption mean that there remain a large number of food insecure people in India. This review has found two key reasons for the failure of PDS in addressing food security: (1) problems with targeting; and (2) problems of diversion and corruption.
5.1.1. Targeting
In 1997, the PDS was restructured, shifting from a universal system, where all Indians, in principle, were eligible to receive a food subsidy, to a system that targeted those most in need. This targeting had two main purposes: (1) to bring down the ever increasing costs of the system; and (2) to provide more food to those in need [ 73 ]. Under this change BPL households continued to receive subsidised foods, while subsidies for APL households were phased out [ 54 ]. BPL households were identified via household income, compared against an absolute income line. However, households with any assets (such as televisions, fans, two or four wheeled vehicles, or land) were classified as APL under this change, and despite owning such goods, many of these APL households were food insecure, and with the removal of rations were unable to purchase sufficient goods [ 21 , 45 , 74 ]. The problem of targeting is compounded by the lack of good quality regular data; no regular official estimates of the actual income of households are conducted, with many households BPL not classified as such, and some BPL households not holding ration cards [ 73 ]. These problems with targeting limit the usefulness of the PDS in acting as an effective food safety network [ 58 , 75 ], and are further exacerbated by the existence of illegal cards [ 76 ].
5.1.2. Diversion and Corruption
Leakage and diversion continue to limit the efficiency of the PDS [ 77 ]. Whole India data have shown mixed results over the past two decades, with figures from 2007–2008 showing a 44 percent diversion rate for grain, down from 55 percent three years earlier. While these figures reflect a decrease in losses from the system, there remains a significant amount of food displaced in the system [ 78 ]. These diversions of commodities, intended for the PDS, into the general market result in shortages for those who rely on subsidised rations [ 73 ]. Targeting has not resolved problems with leakage and diversion, some suggest that it has in fact made it worse [ 79 ]. Dual pricing introduced through the TPDS is seen by some as an incentive for stakeholders to divert commodities into the open market where they can command a higher price [ 80 ]. These diversions and leakages coupled with the inefficiency in monitoring partly due to the decentralised operation increase the likelihood of ingrained, and ongoing corruption [ 80 ].
5.2. Mechanisms to Strengthen the PDS
While there are many challenges with the working of the PDS, it has the potential to play an important role in addressing food security in India. This review has identified two mechanisms to reinforce efficiency into the functioning of the PDS: (1) the National Food Security Act, 2013; and (2) tracking and electronic governance.
5.2.1. The National Food Security Act
The introduction of the NFSA in 2013 was a constructive step towards strengthening the PDS. Representing a shift from a traditional welfare approach, to an approach underpinned by the acceptance of the human right to food and adequate nutrition, the NFSA formalises the aim of the PDS to provide subsidised food grains to over 800 million people, or approximately two thirds of India’s population. The broad nature of the NSFA allowed for a number of existing food security measures to be entered into law. The NSFA is underpinned by a life cycle approach, that is, it considers the nutritional requirements of the population across all age groups [ 81 ], and it includes both universal aspects, available to all Indians, such as the Midday Meal Scheme and the Integrated Child Development Services Scheme, while retaining PDS targeting. Under the NFSA, 75 percent of the rural and 50 percent of the urban population are entitled to 5 kg food grains per month at Rs 3, Rs 2, and Rs 1 for a kg of rice, wheat, and millet, respectively (100 Rupees (Rs) is equal to 1.39 USD).
In addition to the formalisation of a number of pre-existing entitlements, the NFSA aimed to reinforce the role of the states in the coordination of the PDS, as well as improving transparency and accountability [ 26 ]. With empowerment of women and the vulnerable sections of society among the key objectives of the NFSA, monitoring measures to address issues of corruption, diversion and leakages through better partnerships between the central and state governments are also highlighted [ 26 , 28 ].
While the NFSA has every potential to be a “game changer” to strengthen the agricultural industry and the economy of the country, the ability of the NFSA to have a sustained effect on nutrition is questionable [ 10 , 65 ]. Problems with identifying those households in need have not been resolved by the NSFA, and there remains problems with illegal cards [ 82 ]. Finally, the expanding need of food grain associated with the NFSA may be detrimental to the environmental sustainability as it demands increased fertiliser, water, and land use, which if unchecked, may lead to land, air, and water pollution [ 65 ].
5.2.2. Tracking and Electronic Governance
The role of information and communications technology has the potential to be a critical element of success if endorsed and implemented. With the dissemination of digitalisation into the public sector, computerisation can improve the operation of PDS reducing some leakage [ 83 ]. It can aid in the identification of beneficiaries and reduce inclusion and exclusion errors associated with targeting while increasing transparency and accountability [ 56 ]. Other technology currently trialed in some areas is the application of global positioning system in tracking the food supply chain. This approach works by ensuring that goods are scanned in and out at all points of the supply chain, and has so far shown a reduction in corruption, leakages, and diversion [ 57 , 76 ], and has also shown that goods provided to consumers are higher after the implementation of the system [ 84 ].
5.2.3. Food Insecurity in India in a Global Context
India is not alone in seeking a range of measures to combat food insecurity. China, like India has a growing economy and a large population. Despite economic growth in China over recent years, like India, China is home to one of the largest populations of hungry people [ 85 ]. While India relies on the PDS to mitigate food insecurity, China has focused significant attention on programs that seek to redistribute wealth, and non-food based social security. China is also experiencing a shift in diet patterns, a shift that is having an impact on agricultural production and on the use of land [ 86 ]. Likewise, the food insecurity situation in Brazil is undergoing a transformation in the agricultural sector. Like the populations in China and India, the people of Brazil have an increasingly global diet, forcing a change in agricultural patterns. This transition, however, is being supported by the government, alongside cash transfers and school meal programs as an avenue to address increasing food insecurity, with initial indications suggesting some success [ 87 , 88 ]. Given the very large populations in need in these countries, there is unlikely to be a single solution that will work within or across countries. What is important going forward, is that complete and comprehensive data are collected to effectively evaluate these programs.
5.3. Limitations
Several limitations within the literature studied needs to be acknowledged. The limited information on recruitment, data collection, and analysis across the 23 articles included in the review makes comparison difficult and makes any attempt at meta-analysis impossible. The use of secondary data by most of the studies can also affect the quality of the results as it could be outdated or inaccurate among many other pitfalls leading to measurement errors or bias [ 21 , 44 , 54 , 56 , 64 , 65 , 66 , 89 ].
While the authors have attempted to ensure a comprehensive search strategy and methodology for undertaking the literature review, additional articles may have been missed. The complexity of the research topic including the multi-sectoral and multi-dimensional nature of food security has also posed limitations on the literature review. There is also a possibility of relevant grey literature, or literature not available to the public having been missed. Publications in languages other than English may also have been missed.
6. Conclusions
The findings of the review suggest a failure at the policy level. The PDS is a cornerstone of government policy responding to nutrition and food security. However, it is riddled with inefficiencies that decrease its capacity to effectively distribute food to those in need. One positive response has been the implementation of the NFSA in 2013, which has strengthened the PDS by providing statutory backing. As evidenced by the review, policy reforms targeted at improving the operational efficiencies and sustainability aspect of the PDS and NFSA are vital for its success. The PDS may not be able to eliminate the issue of malnutrition and childhood morbidity or mortality in India, but it can reduce the levels of hunger in India if implemented effectively. Integrating the PDS with other interventions including those that will increase transparency and accountability may increase its potential to realise every citizen’s right to nutritious food while propagating good health.
The review is the first of its kind to examine the effectiveness of PDS in addressing food insecurity in India. The study also observed the lack of published research around PDS, NFSA and food security in India. This raises the possibility of missing out on existing interventions that have the potential to improve the food distribution network in India. Overall, the review brings out the need for more dedicated research in the field of food security in India which is vital for identifying best practice solutions that will improve the efficacy and operation of the PDS.
Author Contributions
Conceptualization, N.A.G. and F.H.M.; Data curation, N.A.G. and F.H.M.; Formal analysis, N.A.G. and F.H.M.; Investigation, N.A.G. and F.H.M.; Methodology, N.A.G.and F.H.M.; Project administration, N.A.G.and F.H.M; Supervision, F.H.M.; Writing–original draft, N.A.G. and F.HM.; Writing–review, editing, N.A.G. and F.H.M.
Conflicts of Interest
The authors declare no conflict of interest.
Measuring Food Insecurity in India: A Systematic Review of the Current Evidence
Affiliations.
- 1 School of Health and Social Development, Faculty of Health, Deakin University, Victoria, Australia. [email protected].
- 2 Institute for Health Transformation, Faculty of Health, Deakin University, Victoria, Australia. [email protected].
- 3 School of Health and Social Development, Faculty of Health, Deakin University, Victoria, Australia.
- 4 Institute for Physical Activity and Nutrition (IPAN), School of Exercise and Nutrition Sciences, Deakin University, Geelong, Australia.
- PMID: 37022635
- PMCID: PMC10264273
- DOI: 10.1007/s13668-023-00470-3
Purpose of review: India is home to an estimated 200 million malnourished people, suggesting widespread food insecurity. However, variations in the methods used for determining food insecurity status mean there is uncertainty in the data and severity of food insecurity across the country. This systematic review investigated the peer-reviewed literature examining food insecurity in India to identify both the breadth of research being conducted as well as the instruments used and the populations under study.
Recent findings: Nine databases were searched in March 2020. After excluding articles that did not meet the inclusion criteria, 53 articles were reviewed. The most common tool for measuring food insecurity was the Household Food Insecurity Access Scale (HFIAS), followed by the Household Food Security Survey Module (HFSSM), and the Food Insecurity Experience Scale (FIES). Reported food insecurity ranged from 8.7 to 99% depending on the measurement tool and population under investigation. This study found variations in methods for the assessment of food insecurity in India and the reliance on cross-sectional studies. Based on the findings of this review and the size and diversity of the Indian population, there is an opportunity for the development and implementation of an Indian-specific food security measure to allow researchers to collect better data on food insecurity. Considering India's widespread malnutrition and high prevalence of food insecurity, the development of such a tool will go part of way in addressing nutrition-related public health in India.
Keywords: Food insecurity; Health; India; Measurement; Nutrition; Review.
© 2023. The Author(s).
Publication types
- Systematic Review
- Research Support, Non-U.S. Gov't
- Cross-Sectional Studies
- Food Insecurity
- Food Supply*
- India / epidemiology
- Malnutrition* / epidemiology
- Nutritional Status
Click through the PLOS taxonomy to find articles in your field.
For more information about PLOS Subject Areas, click here .
Loading metrics
Open Access
Peer-reviewed
Research Article
Sustainable food security in India—Domestic production and macronutrient availability
Roles Conceptualization, Data curation, Formal analysis, Investigation, Methodology, Visualization, Writing – original draft, Writing – review & editing
* E-mail: [email protected]
Affiliation School of Geosciences, University of Edinburgh, Edinburgh, United Kingdom

Contributed equally to this work with: David Reay, Peter Higgins
Roles Conceptualization, Supervision, Writing – review & editing
Affiliation Moray House School of Education, University of Edinburgh, Edinburgh, United Kingdom
- Hannah Ritchie,
- David Reay,
- Peter Higgins
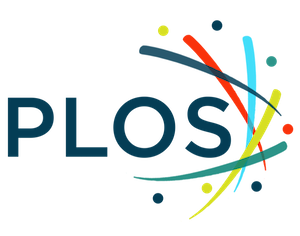
- Published: March 23, 2018
- https://doi.org/10.1371/journal.pone.0193766
- Reader Comments
India has been perceived as a development enigma: Recent rates of economic growth have not been matched by similar rates in health and nutritional improvements. To meet the second Sustainable Development Goal (SDG2) of achieving zero hunger by 2030, India faces a substantial challenge in meeting basic nutritional needs in addition to addressing population, environmental and dietary pressures. Here we have mapped—for the first time—the Indian food system from crop production to household-level availability across three key macronutrients categories of ‘calories’, ‘digestible protein’ and ‘fat’. To better understand the potential of reduced food chain losses and improved crop yields to close future food deficits, scenario analysis was conducted to 2030 and 2050. Under India’s current self-sufficiency model, our analysis indicates severe shortfalls in availability of all macronutrients across a large proportion (>60%) of the Indian population. The extent of projected shortfalls continues to grow such that, even in ambitious waste reduction and yield scenarios, enhanced domestic production alone will be inadequate in closing the nutrition supply gap. We suggest that to meet SDG2 India will need to take a combined approach of optimising domestic production and increasing its participation in global trade.
Citation: Ritchie H, Reay D, Higgins P (2018) Sustainable food security in India—Domestic production and macronutrient availability. PLoS ONE 13(3): e0193766. https://doi.org/10.1371/journal.pone.0193766
Editor: David A. Lightfoot, College of Agricultural Sciences, UNITED STATES
Received: September 13, 2017; Accepted: February 17, 2018; Published: March 23, 2018
Copyright: © 2018 Ritchie et al. This is an open access article distributed under the terms of the Creative Commons Attribution License , which permits unrestricted use, distribution, and reproduction in any medium, provided the original author and source are credited.
Data Availability: All relevant data are within the paper and its Supporting Information files, or can be accessed at the UN FAO databases through the following URL: http://www.fao.org/faostat/en/#home .
Funding: The authors received funding from the Natural Environment Research Council (NERC) as part of its E3 DTP programme.
Competing interests: The authors have declared that no competing interests exist.
Introduction
In 2015, the United Nations (UN) committed to achieving zero hunger by 2030 as the second of the Sustainable Development Goals (SDGs). An important element of this goal is to end all forms of malnutrition, including agreed targets on childhood stunting and wasting. This represents an important progression beyond the Millennium Development Goals (MDGs), where food security was defined and measured solely on the basis of basic energy requirements (caloric intake), and prevalence of underweight children [ 1 ]. This new commitment has significant implications for the focus of research and policy decisions; it requires a broadening of scope beyond the traditional analysis of energy intake, and inclusion of all nutrients necessary for adequate nourishment.
India offers a potentially unique example in the development of models and mechanisms by which nutritional needs can be addressed sustainably. In 2016, India ranked 97 out of 118 on the Global Hunger Index (GHI)—this rates nations’ nutritional status based on indicators of undernourishment, child wasting, stunting and mortality [ 2 ]. Despite ranking above some of the world’s poorest nations, India’s reduction in malnourishment has been slow relative to its recent strong economic growth and puts it behind poorer neighbouring countries [ 3 ]; India has fallen from 80 th to 97 th since 2000.
India’s nutritional problems are extensive. In 2016, 38.7% of children under five were defined as ‘stunted’ (of below average height) [ 2 ], a strong indicator of chronic malnourishment in children and pregnant women, and a largely irreversible condition leading to reduced physical and mental development [ 4 ]. Malnourishment within the adult population is also severe, with approximately 15% of the total population defined as malnourished. The issue of malnutrition in India is complex, and determined by a combination of dietary intake and diversity, disease burden (intensified by poor sanitation and hygiene standards), and female empowerment and education [ 5 ]. Improvements in dietary intake alone will therefore by insufficient to eliminate malnutrition, however it forms an integral component alongside progress in other social and health indicators—particularly sanitation. Quantification of India’s micronutrient and amino acid profiles, and recommendations for addressing these deficiencies have been completed as a follow-up paper (Ritchie et al. in submission) to provide a more holistic overview of its nutritional position.
India’s nutritional and health challenges are likely to be compounded in the coming decades through population growth and resource pressures. Its current population of 1.26 billion is projected to increase to 1.6 billion by 2050, overtaking China as the world’s most populous nation [ 6 ]. India has also been highlighted as one of the most risk-prone nations for climate change impacts, water scarcity, and declining soil fertility through land degradation [ 7 ].
A number of studies have focused specifically on Indian food intake and malnutrition issues from survey assessments at the household level [ 8 ]. The emphasis within India’s agricultural policy and assessment of its success has traditionally been on energy (caloric) intake [ 9 ]. Since the Green Revolution in the 1970s, agricultural policies have been oriented towards a rapid increase in the production of high-yielding cereal crops with a focus to meet the basic calorific needs of a growing population. India has attempted to reach self-sufficiency predominantly through political and investment orientation towards wheat and rice varieties [ 10 ]. While production of staple crops has increased significantly, India’s agricultural policy focus on cereal production raises a key challenge in simultaneously meeting nutritional needs in caloric, high-quality protein and fat intakes. Few studies have addressed the system-wide balance between supply and demand of the three key macronutrients—calories, protein and fat; nor have they assessed the importance of protein quality through digestibility and amino acid scoring. This assessment is particularly significant for India as a result of its extensive and complex malnutrition issues. Whether India is capable of meeting these macronutrient needs in the future through domestic production improvements alone is of prime importance for study, as a result of its growing population and policy orientation towards self-sufficiency.
Improving the availability and access to food at the consumer level requires an understanding of how food is created and lost through its various pathways across the full agricultural supply chain. Here, for the first time, we have attempted to capture this high-level outlook from crop harvesting to residual food availability across the three macronutrient categories.
Mapping the current Indian food system
The Indian food system was mapped from crop production through to per capita food supply using FAO Food Balance Sheets (FBS) from its FAOstats databases [ 11 ]. FBS provide quantitative data (by mass) on production of food items and primary commodities, and their utilisations throughout the food supply chain. Such data are available at national, regional and global levels. Food Balance Sheet data for 2011 have been used, these being from the latest full data-set available. Some aspects of FBS data are available for the years 2012 and 2013, however such data are not complete across all commodities and value chain stages at the time of writing.
Food Balance Sheets provide mass quantities across the following stages of the supply chain: crop production, exports, imports, stock variation, re-sown produce, animal feed, other non-food uses, and food supplied (as kg per capita per year). Data on all key food items and commodities across all food groups (cereals; roots and tubers; oilseeds and pulses; fruit and vegetables; fish and seafood; and meat and dairy) are included within these balances.
While there are uncertainties in FAO data (see Supplementary Information for further discussion on FAO data limitations), FBS provide the only complete dataset available for full commodity chain analysis. Therefore, while not perfect, they provide an invaluable high-level outlook of relative contribution of each stage in the food production and distribution system. As shown in this study (see Results section below), a top-down model using FAO FBS has a discrepancy of <10% with national nutrition survey results at the household level.
FBS do not provide food loss and waste figures by stage in the supply chain. To maintain consistency with FAO literature, food loss figures have therefore been calculated based on South Asian regional percentages within FAO publications [ 12 ]. These percentage figures break food losses down across seven commodity groups and five supply chain stages (agricultural production, postharvest handling and storage, processing and packaging, distribution and consumption). The applied percentage values by commodity type and supply chain stage are provided in S1 Table .
In order to calculate the total nutritional value at each supply chain stage, commodity mass quantities were multiplied by FAO macronutrient nutritional factors [ 11 ]. In this analysis, energy content (kilocalories), protein, and fat supply were analysed. Protein quality is a key concern for India in particular as a result of its largely grain-based diet, with grains tending to have poorer digestibility and amino acid (AA) profiles than animal-based products and plant-based legume alternatives [ 13 ]. To best quantify limitations in protein quality in the Indian diet, protein intakes have therefore been corrected for digestibility using FAO digestibility values [ 14 ].
For consistency, and to provide a better understanding of the food system down to the individual supply level, all metrics have been normalised to average per person per day (pppd) availability using UN population figures and prospects data [ 6 ]. Whilst this provides an average per capita availability value, it does not account for variability in actual macronutrient supply within the population. To help adjust for this, we have also estimated the assumed distribution of supply of each macronutrient using the FAO’s preferred log-normal distribution and India-specific coefficient variation (CV) factor of 0.26 [ 15 ]. Whilst we recognise that food requirements vary between demographics based on age, gender and activity levels, the normalisation of food units to average per capita supply levels is essential in providing relatable measures of food losses within the system, and its measure relative to demographically-weighted average nutritional requirements (as described below) is appropriate in providing an estimation of the risk of malnourishment.
Estimated macronutrient supply has then been compared to recommended intake values. The FAO defines the “Average Daily Energy Requirement” (ADER)—for India’s demographic specifically—as 2269kcal pppd; ADER is defined as the average caloric intake necessary to maintain a healthy weight based on the demographics, occupation, and activity levels of any given population [ 16 ]. Protein requirements can vary between similar individuals; recommended daily amounts (RDA) are therefore typically given as two standard deviations (SD) above the average requirement to provide a safety margin, which some individuals would be at risk of falling below. The World Health Organization (WHO) define a ‘safe’ (recommended) intake in adults of 0.83 grams per kilogram per day (g/kg/d) of body mass for proteins with a digestibility score of 1.0 [ 17 ]. The average vegetarian Indian diet contains lower intakes of animal-based complete proteins; the Indian Institute of Nutrition therefore recommends a higher intake of 1 g/kg/d of total protein for Indians to ensure requirements of high-quality protein are met [ 18 ]. This is equivalent to 55 and 60 grams of protein per day in average adult females and males, respectively based on mean body weight [ 19 ]. Since our analysis attempts to correct for protein digestibility, WHO’s lower safe intake of 0.83g/kg/d would reduce to an equivalent of 50 grams of high-quality protein per day for an average 60 kilogram individual. Consequently in this study we have adopted this RDA value of 50 gpppd.
Dietary fat intake plays a key dietary role in the absorption of essential micronutrients. Several vital vitamins, including vitamin A, D, E and K are fat-soluble—insufficient intake can therefore result in poor micronutrient absorption and utilisation [ 20 ]. Inadequate fat intake can therefore exacerbate the widespread ‘hidden hunger’ (micronutrient deficiency) challenge in India [ 21 ] through poor nutrient absorption. However, daily requirements for fatty acids are less straightforward to determine, relative to energy or protein—there is no widely-agreed figure for total fat requirements for adequate nutrition [ 22 ]. The resolution of food balance sheet data does not allow us to adequately quantity the availability to the level of specific fatty acids. As a result, although we have mapped pathways of total fat availability through the food system in a similar manner to energy and protein, we have not here attempted to quantity the prevalence of potential insufficiency at the household level.
Mapping potential near-term and long-term scenarios
Our initial analysis identified two mechanisms potentially crucial in increasing food availability at the household level: reduction of harvesting, postharvest and distribution losses; and improvements in crop yields. Medium-term (through to 2030) and long-term (2050) scenarios have therefore been mapped based on use of these mechanisms. It should be noted that these scenarios are focused on domestic supply-side measures to enhance food availability as opposed to demand drivers related to consumer preferences. A summary of assumptions used in each scenario in this analysis is provided in S2 Table .
A 2030 baseline scenario (assuming yields stagnate and population growth continues in line with UN projections) and three alternative scenarios to 2030 were analysed:
Scenario 1 (halving food supply chain losses): it was assumed that a significant shift in post-harvest management practices, appropriate refrigeration, and efficient distribution allowed for a halving of food loss percentages at the production, postharvest, processing and distribution stages of the supply chain. This would make its relative losses more in line with those of more developed nations [ 12 ]. In this scenario consumption (household) waste was assumed to remain constant.
Scenario 2 (achieving 50% of attainable yield (AY) across all key crops): the halving of food chain losses in scenario 1 was assumed. In addition, it was assumed that all key crops managed to achieve 50% AY through better agricultural management, irrigation and fertiliser practices. ‘Attainable yield’ is defined as the yield achieved with best management practices including pest, nutrient (i.e. nutrients are not limiting) and water management.
Scenario 3 (achieving 75% AY across all key crops): assumptions as in scenario 2 except an attainment of 75%, rather than 50% AY, has been assumed through crop yield improvements.
Long-term (through to 2050) scenarios were as follows:
Scenario 1 (halving food supply chain losses): the same assumption of halving food loss percentages at the production, postharvest, processing and distribution stages of the supply chain was applied in this scenario. This will require a significant shift in post-harvest management practices, appropriate refrigeration, and efficient distribution, hence 50% reduction represents a magnitude which is more likely to be achieved in this long-term scenario than in the near-term.
Scenario 2 (achieving 75% AY across all key crops): the same assumption of a closure of the yield gap to 75% AY across all crop types, as in the near-term scenario 3, was applied.
Scenario 3 (achieving 90% AY across all key crops): it was assumed that all crop types managed to achieve closure of the yield gap to 90% AY.
To correct for 2030 and 2050 population estimates, all metrics were re-normalised to ‘per person per day’ (pppd) based on a projected Indian population estimate from UN prospects medium fertility scenarios [ 6 ].
To best demonstrate the food production potential of current agricultural support mechanisms, such as governmental policy and subsidy (which largely determine crop choices), the relative allocation of crop production was assumed constant. It was also assumed that production increases were achieved through agricultural intensification alone; this assumption was based on FAOstats data which has shown no increase in agricultural land area over the past decade, indicating a stagnation in agricultural extensification ( http://faostat.fao.org/beta/en/#home ).
Crop yield increases were derived based on closure of current farm yields (FY) to reported attainable yields (AY). FY is defined as the average on-farm yield achieved by farmers within a given region, and AY is defined as the economically attainable (optimal) yield which could be achieved if best practices in water and pest management, fertiliser application and technologies are utilised in non-nutrient limiting conditions). Estimates of crop yield improvements were based on given percentage realisations of maximum attainable yields (AY) attained from published Indian crop-specific figures [ 23 ]. These data are available across all key crop types. Baseline and AY values are provided in S3 Table .
Significant improvements in yield would predominantly be achieved through improved nutrient and water management. In the present study, scenarios were mapped based on achievement of 50% and 75% AY in the near-term. Fifty percent AY should be technically feasible by 2030: many crops have already reached these values, and those which have yet to do so, typically fall short by 3–5% (see S3 Table for baseline, and AY values). Attainment of 75% AY would be highly ambitious in the near-term, representing an increase of >20% in yield. However, 75% AY and higher may be feasible in the long-term if significant investment in agricultural management and best practice were to be realised in this sector.
Our scenarios to 2050 are therefore modelled on the basis of closure of the yield gap to 75% and 90% AY. To assess whether these estimates were realistic, necessary growth rates were cross-checked based on historical yield growth rates in India. Discussion on this comparison and the suitability of attainable yield valuables utilised in this study are available in the Supplementary Discussion.
Climate change impacts on crop yields remain highly uncertain; the importance of temperature thresholds in overall crop tolerance makes yield impacts highly dependent on GHG emission scenarios. This makes it challenging to accurately quantify 2050 climate impacts. As such, we applied average percentage changes in yields of Indian staple crops based on literature review [ 24 ] of field-based observations and climate model results. The studies utilised presented results for a doubling of atmospheric CO 2 from pre-industrial levels. This approximates to a business-as-usual (BAU) scenario for 2050 [ 25 ]. The yield-climate factors applied in this analysis are provided in S4 Table .
It is projected that, through economic growth and shifts in dietary preferences, meat and dairy demand in India will continue to increase through to 2050. It has been assumed that per capita demand in 2050 is in line with FAO projections; this represents an increase in meat from 3.1kg per person per year (2007) to 18.3kg in 2050, and an increase in milk and dairy from 67kg to 110kg per person per year [ 26 ]. We here assume that this increase in livestock production has been met through increased production of crop-based animal feed rather than pasture. The change in macronutrient demand for animal feed was calculated based on energy and protein conversion efficiency factors for dominant livestock types (beef cattle, dairy cattle, ruminants and poultry) [ 27 ].
Our analysis assumes that the per person allocation of crops for resowing and non-food uses, and the relative allocation of land for respective crop selection, is the same as in the initial baseline (2011) analysis.
Current food system pathways
The pathways of macronutrients from crop production to residual food availability are shown for calories, digestible protein and fat in Fig 1A–1C . Across all macronutrients, the relative magnitude of exports, imports and stock variation is small, and approximately balance as inputs and outputs to the food system. This result is in line with India’s orientation towards meeting food demand through self-sufficiency agricultural policies [ 28 , 29 ]. This study’s scenarios are therefore designed to assess whether this same emphasis on self-sufficiency in food supply through to 2050 could be achieved through waste reduction and crop yield improvements alone.
- PPT PowerPoint slide
- PNG larger image
- TIFF original image
Food pathways in (a) calories; (b) digestible protein; and (c) fat from crop production to residual food availability, normalised to average per capita levels assuming equal distribution. Red bars (negative numbers) indicate food system losses; blue bars indicate system inputs; green bars indicate meat and dairy production; and grey bars indicate macronutrient availability at intermediate stages of the chain.
https://doi.org/10.1371/journal.pone.0193766.g001
In 2011, India produced 3159kcal, 72g of digestible protein, and 86g of fat per person per day (pppd) ( Fig 1A–1C ). Across the system, this resulted in average food availability of 2039kcal, 48g digestible protein, and 49g fat pppd; this represents a loss across the food supply system of 35%, 33%, and 43% in calories, digestible protein, and fat respectively.
Our top-down supply model has been cross-checked against India’s National Sample Survey (NSS) data—this reports nutritional intakes bi-annually measured through national household surveys. In its 68 th Round (2011–12) report, the NSS reported average daily intakes of 2206kcal and 2233kcal in urban and rural areas, respectively; 60g of protein in both demographics; and 58g (urban) and 46g (rural) of fat [ 30 ]. Our top-down analysis therefore suggests slightly lower caloric availability than NSS intake figures (but with a discrepancy of <10%); and strong correlation regarding fat intake. Since NSS data reports total protein and take no account of quality or digestibility, our results of digestible protein are not directly comparable. However, with digestibility scores removed, our analysis suggests a total average protein availability of 57g pppd—within 5% of NSS intake results.
Despite the acknowledged uncertainties in FAO FBS datasets (see Supplementary discussion), the strong correlation (within 5–10%) between our top-down supply model and reported household intakes (bottom-up approach) gives confidence in the use of FBS data for high-level food chain analyses such as attempted here.
The largest sources of loss identified in the Indian food system for calories and protein lie in the agricultural production and post-harvest waste stages of the chain, with lower but significant losses in processing and distribution. Consumption-phase losses are comparatively small. Higher losses of fat occur predominantly due to the allocation of oilseed crops for non-food uses; this is in contrast to digestible protein where losses to competing non-food uses are negligible.
In contrast to the average global food supply system, the conversion of crop-based animal feed to meat and dairy produce in India appears comparatively efficient, with an input-output ratio close to one for calories and protein, and an apparent small production of fats [ 31 ]. It is one of the few agricultural systems in the world where the majority of livestock feed demand is met through crop residues, byproducts and pasture lands—its lactovegetarian preferences tend to favour pasture-fed dairy cattle over grain-fed livestock such as poultry (ibid).
Average per capita supply across all macronutrients falls below average per capita minimum requirements. The magnitude of this issue in India emerges via the population-intake distributions. With extension of average macronutrient availability to availability across the population distribution (using a log-normal distribution with CV of 0.26), 66% (826 million) and 56% (703 million) of the population are at risk of falling below recommended energy and protein requirements, respectively.
Potential future pathways
Scenario results for 2030..
Results from scenario analyses for potential food waste reduction and crop yield improvements are summarised in Table 1 . Note that we have assumed no change in income/dietary inequalities, hence the CV in distribution has remained constant.
https://doi.org/10.1371/journal.pone.0193766.t001
Under all scenarios, waste or yield improvements fail to keep pace with population growth through to 2030; average per capita caloric, digestible protein and fat availability all fall below the 2011 baseline. Under current levels of dietary inequality, distribution of availability highlights even greater potential malnourishment. The majority (>75%) of the population are at risk of falling below requirements in energy and protein availability in all scenarios. This represents severe malnutrition across India in 2030, even in the case of significant and ambitious yield and efficiency improvements.
Under these scenarios, India would fall far short of reaching the SDG2 target of Zero Hunger by 2030.
Scenario results for 2050.
India’s anticipated population growth, in addition to potential impacts of climate change on crop yields, could have severe implications on household macronutrient supply by 2050. Our 2050 baseline scenario demonstrates these potential impacts, assuming gains in crop yields were to stagnate at current levels. The full supply chain pathways are shown in Fig 2A–2C . Even at the top level of the supply chain (crop production phase) mean provision per person would fall below average requirements in all macronutrients (2198kcal, 49g protein, and 60g fat per person). Although reducing food system losses plays an important role in improving availability at the household level, this result highlights the necessity of also achieving substantial crop yield improvements at the top of the supply chain.
Food pathways in (a) calories; (b) digestible protein; and (c) fat from crop production to residual food availability, normalised to average per capita levels assuming equal distribution under 2050 baseline conditions. Red bars (negative numbers) indicate food system losses; blue bars indicate system inputs; green bars indicate meat and dairy production; and grey bars indicate macronutrient availability at intermediate stages of the chain.
https://doi.org/10.1371/journal.pone.0193766.g002
How these variables impact on availability at the household level in our 2050 baseline, and three scenarios is detailed in Table 2 , with baseline distributions provided in Supplementary Fig 1A–1C . As shown, even in the case of scenario 1 (halving of supply chain loss and waste), and scenario 2 (increase to 75% of AY), in 2050 greater than 80% of the population would potentially fall below average requirements in energy and protein. Only in the case of significant yield increases to 90% AY (scenario 3) would projected levels of malnourishment approach current levels. This would still leave 62% and 56% of the population at risk of falling below recommended caloric and protein requirements, respectively.
https://doi.org/10.1371/journal.pone.0193766.t002
Our analysis utilised a framework for evaluation of the whole food system (from crop production through to residual food availability) by normalising to consistent and relatively simplistic metrics (per person per day). This holistic approach is critical for identifying levers within the food system which can be targeted for improvements in food security and efficiency of supply. The basic framework is replicable and could therefore be adapted for analysis of any dietary component (for example, micronutrients or amino acids and at a range of scales (global, regional, or national). This allows for similar analyses to be carried out for any nation, potentially allowing for improved understanding of hotspots in the food system and opportunities for improved efficiency. As such, it could then allow national food strategies to focus on components which are likely to maximise improvements.
Overall, our analyses indicate weaknesses in India’s current reliance on domestic food production. Further calculation, based on FAO FBS, make this explicit: in 2011 India’s population was 17.8% of the global total, yet produced only 10.8%, 9%, and 11.8% of the world’s total calories, digestible protein and fat respectively. Based on calculations using FAOstats global crop production data and nutritional composition factors, in 2011 world crop production totalled 1.34x10 16 kcal; 3.62x10 14 g digestible protein; and 3.33x10 14 g fat. 2011 Indian production amounted to 1.44x10 15 kcal; 3.27x10 13 g digestible protein; and 3.93x10 13 g fat. Even in a highly efficient food system, self-sufficiency is impossible to achieve based on such production levels and the need to provide sufficient nourishment for all. Likewise, even if Indian population figures were to plateau, it is unlikely that domestic production alone would be sufficient to close the current food gap.
Current malnutrition levels—defined here as insufficient macronutrient availability—in India are already high. Sufficient nutrition requires adequate availability and intake of all three macronutrients. Impacts of insufficient protein and energy intake can often be difficult to decouple, and are often termed protein-energy malnourishment (PEM)—PEM has a number of negative consequences including reduced physical and mental development [ 32 ]; increased susceptibility to disease and infection; poorer recovery and increased mortality from disease; and lower productivity [ 33 ]. Our results indicate that India’s self-sufficiency model—a reliance on domestic crop yield increases and waste reduction strategies—will be insufficient to meet requirements across all three macronutrients. Levels of undersupply and consequent malnutrition would show a significant increase in both 2030 and 2050 scenarios.
This has important implications for forward planning to effectively address malnutrition. Policy incentives in Indian agriculture since the Green Revolution have predominantly been focused on achieving caloric food security through increased production of cereals (wheat and rice) [ 9 ]. This has resulted in a heavily carbohydrate-based diet (> 65–70% total energy intake [ 34 ]) which may be significantly lacking in adequate diversity for provision of other important nutrients [ 35 ]. Widespread lactovegetarian preferences have further reduced the scope for dietary diversity [ 36 ].
If trying to address caloric inadequacy alone, efforts to increase output of energy-dense crops (i.e. cereals, roots and tubers) may seem appropriate, and has largely been India’s focus to date [ 8 ]. Our analysis, however, strongly suggests the need to shift dietary composition away from reliance on carbohydrates towards a more diversified intake of protein and fats (with diversification also contributing to a reduction in micronutrient deficiency) [ 37 ]. Forward planning therefore needs to simultaneously address caloric inadequacy and malnourishment through balanced, increased supply and intake of high-quality proteins and fats.
Our examination of macronutrient supply in India indicates large inequalities in availability across the population. This is likely to be closely coupled to the high levels of income inequality and poverty which remain in India today [ 8 ]. Large inequalities in food supply and dietary intake will make it increasingly difficult for India to address its malnutrition challenges; our assessment of potential improvement scenarios highlight that, even in cases where average macronutrient supplies meet requirements, the high CV in distribution still leaves a large proportion of the total population at risk of malnourishment. Whilst the RDA values used in this analysis account for distribution in nutritional requirements of individuals, they do not account for the distribution in intake. To meet SDG2 (whereby all individuals’ requirements are met) at current levels of inequality, the national mean intake would therefore have to increase to 3600kcal pppd; 82g pppd digestible protein; and 105g pppd fat. This is well above current national pppd supply values, even if crop production-phase level were to be at the top of the food system.
It should be emphasised that this work is a largely computational, supply-driven analysis exploring the domestic capacity of India’s food. Our results are not intended to imply actual future scenarios of Indian malnutrition. Projections of acute food shortage implied within this analysis would be likely to drive market and policy interventions including enhanced trade, in addition to changes in consumer and producer responses. The interaction between supply and demand-side measures, commodity prices, trade, and governmental policy creates an important feedback loop for food pricing, affordability and production [ 38 ]. For example, the estimated reduction in per capita food supply and domestic food shortage would be expected to drive an increase in food prices [ 39 , 40 ]. Rising food prices (as are expected across a number of countries where food demand continues to grow [ 41 ]) create a number of producer and consumer impacts, including per capita food expenditure, reduced purchasing power for expensive commodities such as meat and dairy products [ 42 ], farmer incentives and agricultural investment. Analysis of the drivers of historical food price volatility and inflation in India suggests that both supply and demand-side factors (and the interaction between) play an important role [ 40 ].
The impact of feedbacks such as reduced meat and dairy demand (thereby reducing demands for feed, with further feedbacks on food supply and commodity prices) are not reflected within these scenarios, but will play an important role in determining food system dynamics. The impact of domestic food shortages, agricultural prices and balance within international markets is particularly pronounced in India where the agricultural sector accounts for the employment and income of a large percentage of the population [ 43 ]. Literature on the interactions between poverty, agriculture and food prices is extensive; many studies indicate that, since a large share of the world’s poor are rural, high food prices have a positive long-term impact on poverty reduction. However they have negative impacts on poverty and malnutrition in the short-term [ 39 , 44 – 48 ]. The lack of domestic capacity in India to meet the full nutritional needs (balancing caloric, protein and micronutrient requirements) of its population is likely to increase the demand for commodity imports. This in turn creates further feedbacks on domestic prices, farmer income and inevitably poverty reduction [ 46 ]. Further work on the economic dimension to Indian food security—within the context of value chain potential and efficiency evaluations in this study—is therefore crucial to develop better understanding of their interactions and policy responses.
Overall, our results highlighted several key points:
- production quantities at the farm level are very low relative to global average production;
- low import and export values produce an approximately balanced trade model; this correlates with India’s self-sufficiency focused agricultural and food policies;
- harvesting, post-harvest and distribution losses in the supply chain form a large proportion of total food system inefficiencies;
- a moderate amount of energy and fat (but not protein) is allocated to non-food uses, although this is significantly less than global average non-food allocation;
- India’s caloric and protein losses in the conversion of edible crops to livestock are small due to the dominance of pasture-fed livestock such as dairy cattle. The large nutritional gains achieved through increased milk consumption in India suggest this may be a beneficial trade-off in agricultural land for provision of high-quality protein.
Our examination of the food supply chain in India identified harvesting, handling and storage losses, and top-level crop production to be the key intervention phases for improving food security. The approach not only adds value in the identification of ‘hotspots’ of wastage and inefficiency, but also allows for an understanding of the magnitude of change required to produce a certain food supply chain-wide result. Our analysis highlighted that, despite being an important mechanism for improving food security, even a 50% reduction in food loss/waste (a challenge that is achievable but would take significant economic, infrastructural and educational investment) alone would be largely insufficient in ensuring food security in India.
Increased production at the agricultural level must therefore be a focus for both near and long-term food security. The viability of achieving yields close to 75% AY in the near-term (to 2030), across the range of available crops, needs to be more closely considered. For several staple crops, a yield increase upwards of 30% and 50% would be required for attainment of 75% and 90% AY, respectively (see S2 Table ). The challenge in reaching close to 90% AY (i.e. almost maximum yield) is substantial; many developed countries have not yet reached such levels [ 23 ].
The potential resource limits and environmental implications needed to achieve such yields also need to be given consideration in order to optimise crop selection and mitigate negative impacts. The yield gap could predominantly be closed through improved water and nutrient management [ 23 ]. Depleting groundwater resources through agricultural irrigation in India raises key concerns over long-term water security [ 49 ][ 50 ], and whether water availability is likely to impose a resource limit on yield attainment. Improved yields through increased fertiliser application raise similar sustainability concerns; nitrous oxide (N 2 O) is a key source of greenhouse gas (GHG) emissions, a major source being microbially-mediated emissions as a result of nitrogen fertiliser application to agricultural soils [ 51 ]. There may therefore be a significant GHG penalty in closing the current yield gap.
It should be noted that this study has considered only yield improvements through traditional crop varieties. Genetic variation and modification of crop strains may offer further potential for yield increases, in addition to increased resilience to pests, disease and climatic impacts [ 52 ]. However, with the exception of Bt Cotton, genetically modified (GM) crop varieties are banned from commercial crop production [ 53 ]. Despite the introduction of GM field trials in recent years, they continue to face significant resistance across a range of stakeholder groups [ 54 ].
Our analyses for 2050 highlight severe food security challenges for India, even in scenarios which assume attainment of 90% AY for all crops. In addition to the hotspots identified for further focus to achieve near-term improvements, long-term strategies require increased consideration of the impact of potential climatic changes. India’s staple crops–wheat and rice—show particular vulnerability; in the near-term, CO 2 fertilisation may offer some positive yield impacts, however, simulated climate models suggest this effect is likely to be cancelled out if global mean temperature increase reaches a 3°C threshold in wheat (2°C for rice) [ 55 ]. This suggests negative climate impacts may only begin to arise from mid-century onwards. Failure to build capacity and agricultural resilience in the interim could result in severe food deficits should a 2°C or 3°C warming threshold be breached. Planning strategies should therefore not only aim to adapt to gradual near-term impacts of a changing climate, but importantly focus on capacity-building for a resilient food system in a warmer post-2050 world.
Our 2050 scenarios are based on assumptions which are sensitive to change; we have assumed BAU climatic-yield factors, and increased meat and dairy intakes in line with FAO projections. Both of these assumptions could change based on global GHG mitigation progress, and governmental or social interventions on meat consumption. In addition, it is recognised that some potential climatic impacts could be reduced through shifts in crop production regions and seasonal cropping patterns [ 24 ]. While such changes may marginally change the scale of the food supply and malnutrition challenge, the overall conclusions remain the same. Climatic and livestock impacts may serve to exacerbate the issue, however, India would continue to face a severe risk of domestic food shortages regardless of these additional pressures.
To deliver effective recommendations for addressing macronutrient undersupply and malnutrition, two key components need to be further explored. Firstly, there needs to be better understanding of optimal crop selections to maximise production and consumer supply of energy, digestible protein and fats alike. This has to be analysed with key resource and environmental constraints in mind to deliver a more optimal and sustainable domestic food system. This should include consideration of options outwith traditional domestic agricultural practice, such as genetic modification, industrial biotechnology and biofortification [ 56 , 57 ].
Secondly, India’s role within global food markets needs to be more closely assessed. To successfully address malnutrition, India will likely have to fill the gap between domestic production and food demand through increased imports. Food imports can have a significant impact on domestic prices, and the dominance of agriculture as a primary source of employment in India may be a negative influence on farmer livelihoods [ 9 ]; and further, a large increase in food imports could potentially reduce energy-protein intake for the poorest 30% of the population [ 46 ]. This means appropriate economic and social analysis must be carried out to try to optimise import quantities and products which will have minimal domestic impacts. The importance of reducing economic and dietary inequalities makes this even more crucial.
In order to ensure a resilient food system, such analyses and recommendations should be made alongside consideration of potential climatic impacts in the medium- and long-term. This would allow for appropriate choices to be made in the near-term that are also sustainable in a changing climate. The implications of our analysis for health, social, and environmental policy is discussed in detail in our Supplementary Discussion.
Closing its current food supply and nutrition gap while meeting increasing population demand will require a combination of domestic measures to improve agricultural practice and subsequent yields, in addition to a well-planned increase in food imports.
Supporting information
S1 file. supplementary discussion..
https://doi.org/10.1371/journal.pone.0193766.s001
S1 Table. Loss and waste percentages by food chain stage and commodity group for South and Southeast Asia.
Due to poor data availability on India-specific food loss figures, regional average figures from the FAO were applied to derive estimates of macronutrient losses at each stage in the Indian commodity chain.
https://doi.org/10.1371/journal.pone.0193766.s002
S2 Table. Assumptions and sources for figures used within all scenarios from 2011 baseline to 2050 scenarios.
https://doi.org/10.1371/journal.pone.0193766.s003
S3 Table. Indian baseline and attainable yield (AY) values for key crop types.
Year 2000 and all attainable yield values have been derived from Mueller et al. (2012)[ 23 ][ 23 ][ 23 ][ 23 ][ 23 ](23)(23)(23)(23)(23)(23)(23)(23)(23)(23)(22)(21)(21)(21), and 2011 yield data derived from the FAOstats database ( http://faostat.fao.org/beta/en/#home ). The necessary percentage increase in yield from 2011 levels to reach each of the AY values has also been shown.
https://doi.org/10.1371/journal.pone.0193766.s004
S4 Table. Average estimated climatic impacts on Indian crop yields in 2050.
Average values have been assumed based on the range of historic studies on yield sensitivities and climatic models within literature review [ 24 ]. These models are projected on the basis of a doubling of CO2 from pre-industrial (which is approximately equivalent to a business-as-usual scenario).
https://doi.org/10.1371/journal.pone.0193766.s005
- 1. United Nations. Road map towards the implementation of the United Nations Millennium Declaration. New York: 2001.
- 2. Klaus von Grebmer, Jill Bernstein, Nilam Prasai, Shazia Amin YY. Global Hunger Index: Getting to Zero Hunger. Washington, DC: 2016.
- View Article
- Google Scholar
- PubMed/NCBI
- 6. United Nations. UN Population Prospects 2015. http://esa.un.org/unpd/wpp/ (accessed February 6, 2016).
- 11. FAO. Food Balance Sheets Handbook. Rome: 2001.
- 12. FAO. Global food losses and food waste–Extent, causes and prevention. Rome: 2011.
- 14. World Health Organization. Energy and protein requirements. Geneva: 1991.
- 15. FAO. Refinements To the FAO Methodology for Estimating the Prevalence of Undernourishment Indicator. Rome: 2014.
- 16. FAO, IFAD, WFP. The State of Food Insecurity in the World: Meeting the 2015 international hunger targets: taking stock of uneven progress. 2015. I4646E/1/05.15.
- 17. WHO/FAO/UNU Expert Consultation. Protein and amino acid requirements in human nutrition. Geneva: 2007. ISBN 92 4 120935 6.
- 18. National Institute of Nutrition. Nutrient Requirements and Recommended Dietary Allowances for Indians. 2009.
- 22. FAO/WHO. Fats and fatty acids in human nutrition, Report of an expert consultation. vol. 91. Rome: 2008. 978-92-5-106733-8.
- 25. IPCC. Climate Change 2014 Synthesis Report Summary Chapter for Policymakers. IPCC Fifth Assess Rep 2014. 10.1017/CBO9781107415324.
- 29. Subramaniam GS, Subramaniam SR. Does India attain self sufficiency in food production? 2009.
- 30. National Sample Survey Office. Nutritional Intake in India, 2011–12. vol. 471. New Delhi: 2014.
- 38. Evans A. Rising Food Prices: Drivers and Implications for Development. London: 2008.
- 40. Sekhar CS., Roy D, Bhatt Y. Food Inflation and Food Price Volatility in India: Trends and Determinants. New Delhi: 2017.
- 43. World Bank. World Development Indicators 2017. https://data.worldbank.org/ (accessed June 6, 2017).
- 44. Polaski S. Rising Food Prices, Poverty, and the Doha Round. Washington D.C.: 2008.
- 45. Headey D. Food Prices and Poverty Reduction in the Long Run. Washington D.C.: 2014.
- 46. Panda M, Ganesh-Kumar A. Trade liberalization, poverty, and food security in India. New Delhi: 2009.
Keynote webinar | Spotlight on medication adherence
LIVE: Thursday 27th June 2024, 18:00-19:30 (CEST) Join our expert panel to discover why you need to understand the drivers of non-adherence in your patients, and how you can optimize medication adherence in your clinics to drastically improve patient outcomes.
Springer Medicine
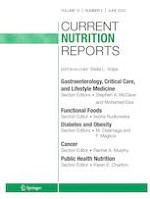
Open Access 06-04-2023 | Nutrition | REVIEW
Measuring Food Insecurity in India: A Systematic Review of the Current Evidence
Authors: Fiona H. McKay, Alice Sims, Paige van der Pligt
Published in: Current Nutrition Reports | Issue 2/2023
Purpose of Review
Recent findings, please log in to get access to this content, other articles of this issue 2/2023, nutritional quality and biological application of mushroom protein as a novel protein alternative, optimal nutrition in the older adult: beneficial versus ineffective supplements, a narrative review on the potential role of vitamin d3 in the prevention, protection, and disease mitigation of acute and long covid-19, dietary intake and systemic inflammation: can we use food as medicine, dairy milk protein–derived bioactive peptides: avengers against metabolic syndrome, dietary patterns and risk of lung cancer: a systematic review and meta-analyses of observational studies, live: thursday 27th june 2024, 18:00-19:30 (cest).
WHO estimates that half of all patients worldwide are non-adherent to their prescribed medication. The consequences of poor adherence can be catastrophic, on both the individual and population level.
Join our expert panel to discover why you need to understand the drivers of non-adherence in your patients, and how you can optimize medication adherence in your clinics to drastically improve patient outcomes.
At a glance: The STEP trials
A round-up of the STEP phase 3 clinical trials evaluating semaglutide for weight loss in people with overweight or obesity.
Highlights from the ACC 2024 Congress
Year in Review: Pediatric cardiology
Watch Dr. Anne Marie Valente present the last year's highlights in pediatric and congenital heart disease in the official ACC.24 Year in Review session.
Year in Review: Pulmonary vascular disease
The last year's highlights in pulmonary vascular disease are presented by Dr. Jane Leopold in this official video from ACC.24.
Year in Review: Valvular heart disease
Watch Prof. William Zoghbi present the last year's highlights in valvular heart disease from the official ACC.24 Year in Review session.
Year in Review: Heart failure and cardiomyopathies
Watch this official video from ACC.24. Dr. Biykem Bozkurt discusses last year's major advances in heart failure and cardiomyopathies.
ACC 2024 Congress Coverage
Semaglutide benefits people with type 2 diabetes and obesity-related heart failure, incentivised to exercise, new intervention for stemi-related cardiogenic shock, » view more highlights on the acc 2024 congress hub page.
- Medical Journals
- Webcasts & Webinars
- CME & eLearning
- Newsletters
- ESMO Congress 2023
- 2023 ERS Congress
- ESC Congress 2023
- Advances in Alzheimer’s
- About Springer Medicine
- Diabetology
- Endocrinology
- Gastroenterology
- Geriatrics and Gerontology
- Gynecology and Obstetrics
- Infectious Disease
- Internal Medicine
- Respiratory Medicine
- Rheumatology
- Reference Manager
- Simple TEXT file
People also looked at
Review article, soil salinity and food security in india.
- 1 Department of Soil Science, Chaudhary Sarwan Kumar (CSK), Himachal Pradesh Krishi Vishvavidyalaya (HPKV), Palampur, India
- 2 Sher-e-Kashmir University of Agricultural Sciences and Technology, Jammu, India
India would require around 311 million tons of food grains (cereals and pulses) during 2030 to feed around 1.43 billion people, and the requirement expectedly would further increase to 350 million tons by 2050 when India's population would be around 1.8 billion. To achieve food security in the country, the attempts need to focus on both area expansion under agriculture as well as rise in crop productivity. Massive urbanization is putting pressure on agricultural lands, resulting in shrinking of land holdings. The possibility of area expansion under agriculture, therefore, exists in restoring the degraded lands. Nearly 147 million ha of land is subjected to soil degradation, including 94 million ha from water erosion, 23 million ha from salinity/alkalinity/acidification, 14 million ha from water-logging/flooding, 9 million ha from wind erosion and 7 million ha from a combination of factors due to different forces. Government of India has fixed a target of restoring 26 million ha of degraded lands, including salt-affected soils, by the year 2030 to ensure food security for the people. Around 6.74 million ha area in the country is salt-affected. Estimates suggest that every year nearly 10% additional area is getting salinized, and by 2050, around 50% of the arable land would be salt-affected. Saline soils occupy 44% area covering 12 states and one Union Territory, while sodic soils occupy 47% area in 11 states. The ICAR-Central Soil Salinity Research Institute and many State Agricultural Universities are engaged in studying salt-affected soils and developing reclamation technologies and strategies. Several innovative technologies have been developed and on-farm tested. Gypsum-based sodic soil reclamation, sub-surface drainage of water-logged saline lands, salt tolerant crop varieties and improved agroforestry techniques are some of the well-adapted technologies in the country. Reclamation of 2.18 million ha of salt-affected soils (2.07 million ha barren sodic soils and 0.11 million ha saline soils) has contributed more than 17 million tons of food grains per annum to the country's food basket, with additional annual income of Rs. 15.5 billion, and employment generation of 2.8 million man-days. Other technologies of management of salt-affected soils, viz. alternate land-use systems, saline aquaculture, cultivation of salt tolerant crop varieties, agro-forestry, phytoremediation, bioremediation etc. have positively impacted food and nutritional security, women empowerment, involvement of landless laborers and minimizing rural migration etc. The ongoing consistent research efforts for the management and reclamation of such soils would hopefully continue ensuring food security in the country. The Government needs to make policies favorable for implementation of reclamation technologies in the country.
Introduction
India supports nearly 18% of the world's human population and 15% of the world's livestock population on merely 2.4% of the world's geographical area ( Bhattacharyya et al., 2015 ). Since independence, India has made significant achievement in agriculture sector. Food grain production increased by about 5.5 times, from merely 50 million tons in 1950 to 275 million tons in 2017, making India not only self-sufficient but net exporter of food grains. According to Tiwari (2020) , with a record production of rice and wheat at 116.48 and 103.60 million tons, respectively, the country registered record food grain production of 285.17 million tons in 2018–19. While the increase in food grain production during 1949–65 was mostly due to area expansion under cultivation ( Narain, 1977 ; Vaidyanathan, 1986 ), after mid-sixties, the adoption of a package of high yielding inputs, including use of high yielding varieties, assured irrigation, use of plant protection measures and credit support was responsible for increased production ( Dantwala, 1986 ). It ushered green revolution in India.
In spite of the technological innovations in agriculture, which dramatically increased food production in the past few decades ( Godfray et al., 2010 ), food security globally is being challenged by several factors including climate change ( Parry et al., 1999 ; Rosenzweig et al., 2004 ; Godfray et al., 2010 ), unabated land and environmental degradation ( Oldeman, 1998 ; Pimentel, 2006 ), deforestation, intensive cropping, and biodiversity loss ( Foley et al., 2005 ; Lotze-Campen et al., 2008 ; Tscharntke et al., 2012 ), land use change ( Lotze-Campen et al., 2008 ; Godfray et al., 2010 ), fresh water scarcity ( Rijsberman, 2006 ; Lotze-Campen et al., 2008 ), increased population pressure, increased urbanization and huge food wastage ( Parfitt et al., 2010 ), dietary transition ( Rijsberman, 2006 ; Godfray et al., 2010 ), poverty and social inequality ( Elobeid et al., 2000 ) etc. Sustainability of rice-wheat cropping system in the Indo-Gangetic plains of India has been challenged, as evidenced by the stagnating rice-wheat yields and declining factor productivity during the last about three decades, by the fast receding water table, climate variability, deteriorating soil health, environmental pollution, and secondary salinization ( Aggarwal et al., 2004 ).
Population growth in India has also kept pace with food production. According to the 2017 revision of the World Population Prospects, India's population stands around 1.32 billion ( UNDESA, 2017 ), although, with the Government policies and public awareness, India's population growth rate has shown decline from 2.3% in late 1970s and early 1980s to around 1.13% in 2017 ( Halawar, 2019 ). Even at this growth rate, India is projected to be the world's most populous country by 2024. The massive population increase (despite the slowing down of the growth rate) and substantial income growth demand an extra about 2.5 million tons of food grains annually, besides significant increases in the supply of livestock, fish, and horticultural products. The growth in food grain productivity has stagnated around 2% per annum.
The changing lifestyle and food habits of the people, due to the sustained economic growth, literacy and awareness, are other challenges associated with food security in India. People in general are shifting from staple food grains toward high-value horticultural and animal products ( Kumar et al., 2007 ; Mittal, 2007 ). Although it may lower per capita food grain requirement, yet overall demand for food grains would increase for increasing population and increasing food needs of livestock and poultry. The grain requirement for rearing cattle and poultry etc. is comparatively high because of low and variable efficiency with which various animals convert grains into protein. Table 1 provides global average feed conversion efficiencies for different animal categories and production systems. To produce 1 kg of beef, pork, poultry, and herbivorous species of farmed fish (such as carp, tilapia, and catfish), it takes around 7, 4, 2, and <2 kg of grains, respectively. Currently, livestock supply 13% of energy to the world's diet but consume one-half the world's production of grains to do so ( Smith et al., 2013 ). India would require around 311 million tons of food grains (including pulses) during 2030, and the requirement would further increase to 350 million tons by 2050 ( Kumar et al., 2016 ). At the current growth rate in agricultural production, food security in India appears to be a big challenge.
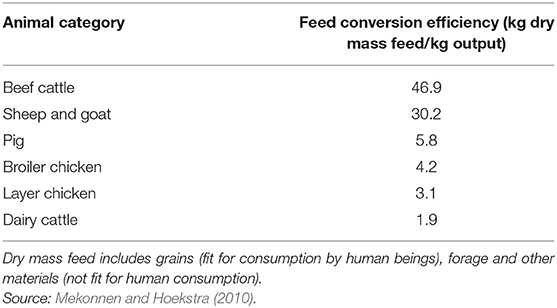
Table 1 . Global average feed conversion efficiency per animal category and production system.
The projections of higher food requirements due to demographic, economic, and trade liberalization are exerting heavy pressures on India's limited land and water resources. It is estimated that nearly 174.4 million ha of land is potentially exposed to various degradation forces. Land degradation in some regions of India, especially in arid and semi-arid tracts (desertification), is touching irreversible limits. Land degradation has become a big challenge to policy makers who need to balance the multiple goals of poverty eradication, food security, and sustainable land management.
Soil salinization alone has rendered significant chunks of land unproductive or less productive. Soil salinization is a global and dynamic problem and is projected to increase in future under climate change scenarios, viz. rise in sea level and impact on coastal areas, rise in temperature and increase in evaporation etc. Precise statistics on the recent estimates of global extent of salt-affected soils are not available and different data sources provide variable information ( Shahid et al., 2018 ). The global figure of 954.83 million ha as reported by Szabolcs (1989) has been considered authentic. However, figures such as 932.2 million ha ( Sparks, 2003 ) and 952.2 million ha ( Arora et al., 2016 ) have also been reported. According to Mandal et al. (2018) , more recent estimates show an increasing trend in global salt-affected area with an area of 1,128 million ha. According to an estimate, 20% of total cultivated and 33% of irrigated agricultural lands worldwide are afflicted by high salinity ( Shrivasata and Kumar, 2015 ). Around 52 million ha lands are salt-affected in South Asia ( Mandal et al., 2018 ). Around 85% area worldwide is only slightly to moderately affected by high salt concentrations while the remainder 15% suffers from severe to extreme limitations for crop cultivation ( Wicke et al., 2011 ). In India, the salt-affected soils constitute nearly 5% of the net cultivated area, spreading from Jammu & Kashmir (Ladakh region) in north to Kanyakumari in south, and Andaman & Nicobar Islands in the east to Gujarat in the west.
Soil salinization, in addition to reducing net cultivable area, has serious implications for agricultural productivity and quality, the choice of cultivable crops, biodiversity, water quality, supply of water for critical human needs and industry, the longevity of infrastructure and the livelihood security of the people. For all important crops, average yields in salt stressed environments are only a fraction, somewhere between 20 and 50% of record yields ( Shrivasata and Kumar, 2015 ). Estimates suggest global economic losses due to soil salinization around US $ 27.3 billion per year ( Qadir et al., 2014 ).
Growing trend in the salt-affected soils in India is becoming a threat to national food security and economic development. A paradigm shift is, therefore, needed in the policy and methodology of food production in the country. Food security attempts need to focus on both area expansions under agriculture as well as rise in crop productivity. Restoration of degraded lands, including salt-affected soils, offers a potential opportunity of sustaining food security in the country. With this thing in mind, the Government of India has fixed a target of restoring 26 million ha of degraded lands by the year 2030.

Soil Salinity and Soil Salinization
Soil salinity is an index of the concentration of salts in soil and is usually expressed as electrical conductivity (EC). Soil salinization is a process by which there is build-up of salt concentration in soil to such a level that impacts on the agricultural production, environmental health, and economics and quality of life. Soil salinization involves a combination of processes like evaporation, salt precipitation and dissolution, salt transport, and ion exchange etc.
The salt-affected soils contain excessive concentrations of either soluble salts or exchangeable sodium or both due to inadequate leaching of base forming cations. The major soluble mineral salts are the cations: sodium (Na + ), calcium (Ca 2+ ), magnesium (Mg 2+ ), potassium (K + ) and the anions: chloride (Cl − ), sulfate ( SO 4 2 - ), bicarbonate ( HCO 3 - ), carbonate ( CO 3 2 - ), and nitrate ( NO 3 - ). Hyper-saline soil water may also contain boron (B), selenium (Se), strontium (Sr), lithium (Li), silica (Si), rubidium (Rb), fluorine (F), molybdenum (Mo), manganese (Mn), barium (Ba), and aluminum (Al), some of which can be toxic to plants and animals ( Tanji, 1990 ).
Soil salinization may occur through both natural and anthropogenic reasons. Out of 932.2 million ha salt-affected soils worldwide, the extent of human-induced salinization is 76.6 million ha ( Oldeman et al., 1991 ; Mashali, 1995 ; Shahid et al., 2018 ). Arid and semi-arid regions, where evaporation rates are high and fresh waters are scanty to flush out the excess salts from soil, favor the formation of such soils. Gupta and Abrol (1990) have extensively reviewed processes of soil salinization.
a. Natural processes of soil salinization ( i.e ., primary salinization)
• Weathering of parent material : During the process of weathering of rock minerals or sediments with high salt content (physically, chemically, and biologically), salts are released and made soluble. They are transported away from their source of origin through surface or groundwater streams. In arid regions, the concentration of salts gradually increases until they start precipitating in soil due to limited natural precipitation and leaching, high evaporation and transpiration rates. Low-lying areas with high groundwater table and locked topography favor salinization.
• Fossil salts : The fossil salt deposits (e.g., marine and lacustrine deposits) are also responsible for salinization in arid regions. Fossil salts can be dissolved under water storage or water transmission structures causing salinization ( Bresler et al., 1982 ).
• Salinization in coastal lands : The ingression of sea-water along the coast increases salt contents in coastal areas ( Rao et al., 2014 ). The salt-laden winds and rains (sea sprays) along sea coasts carry oceanic salts along with them in quantities sufficient to cause salinization in coastal areas. The sea sprays may contain salt content as high as 14.2 μg m −3 ( Prospero, 1979 ), and may show impact as deep as 80 km inland or even more. The coastal regions are also exposed to the risk of progressive salinization of land due to processes like storms, cyclones, tidal surges, flooding etc.
• Transport of salts in rivers : The salts brought down from the upstream by rivers to the plains and their deposition along with alluvial materials and weathering of rocks may also cause salinization.
b. Anthropogenic reasons of soil salinization (i.e., secondary salinization)
• Land clearing for cultivation : Replacement of perennial vegetation with annual crops, may result into soil salinization due to saline seepage process. Change of land use from natural forest vegetation to annual food crops decreases evapotranspiration and increases leaching. The presence of impermeable/less permeable subsoil layers may intercept the percolating water passing through saline sediments resulting in lateral seepage, causing salinization in low lying areas ( Doering and Sandoval, 1976 ).
• Incorrect irrigation : Indiscriminate use of brackish and saline irrigation water, poor drainage conditions, rising water tables etc., lead to secondary salinization of land and water resources ( Rao et al., 2014 ). Even irrigation with good quality water over a period of time in the absence of proper soil-water-crop management practices may cause salinization. Fall of civilizations like Mesopotamia, Nile Valley, Mohanzoadaro, and Indus Valley are glaring examples of imminent occurrence of salinity following irrigation ( Dagar, 2005 ). Currently worldwide 310 million ha area is irrigated, out of which 20–33% area is estimated to be salt-affected ( Glick et al., 2007 ; Jamil et al., 2011 ; Qadir et al., 2014 ; Shahid et al., 2018 ). Irrigation with sea water causes salinization in coastal areas.
• Over extraction of groundwater : It brings salts to soil surface where they get precipitated when water evaporates ( Rao et al., 2014 ).
• Canal water seepage : It is a serious problem leading to rise in water table and salinity development along the banks of canals. Water-logging and soil salinization in the Indira Gandhi Nahar Priyojna (IGNP) area in India is a glaring example of this process. Around 50% of the command area of IGNP has experienced water-logging ( Tewari et al., 1997 ).
• Over-use of agro-chemicals : Over-use of chemical fertilizers and soil amendments (lime and gypsum) may also lead to soil salinization.
• Use of waste effluents: Use of sewage sludge and/or untreated sewage effluent, dumping of industrial brine onto the soil etc. may also cause soil salinization. Of particular concern is the entry of heavy metals into soils.
At several occasions the socio-economic and political considerations become extremely important in accelerating soil salinization processes. Many times, such factors are beyond the control of individual farmers. Some of such examples, especially in developing countries, may be the ill-conceived or poorly implemented irrigation schemes, intensive vs. extensive irrigation, over-irrigation due to zero water pricing, small and scattered land holdings etc. It is, therefore, the responsibility of respective governments to take appropriate policy decisions and corrective measures in order to keep a check on soil salinization.
Characteristics of Salt-Affected Soils
The salt-affected soils are classified into three groups depending on the nature and concentration of salts present in them:
i. Saline soils (also called “white alkali” or “solonchak” soils): soils containing calcium, magnesium, and sodium as predominant exchangeable cations (Ca and Mg more than Na), and sulfate, chloride, and nitrate the predominant anions; sodium adsorption ratio (SAR) <13; exchangeable sodium percentage (ESP) <15 of total CEC; pH <8.5; EC of saturation extract >4 dS m −1 ; white color due to white crust of salts on the surface; good permeability for water and air; salt problems in general; the salt concentration is enough to adversely affect the growth of most crop plants; mostly found in arid or semi-arid regions where less rainfall and high evaporation rates tend to concentrate the salts in soils; rarely found in humid regions.
ii. Sodic soils (also called “non-saline sodic soils” or “alkali soils,” or “solonetz”): soils high in exchangeable sodium compared to calcium and magnesium; sodium carbonate and sodium bicarbonate are the predominant salts; SAR >13; ESP >15; pH = 8.5–10.0; EC of saturation extract <4 dS m −1 ; black color; poor permeability for water and air; soils formed due to exchange of Ca 2+ and Mg 2+ ions by Na + ions; sodium problems.
iii. Saline-sodic soils : these soils are transitional between saline and sodic soils; SAR >13, ESP >15, pH >8.5; EC of saturation extract >4 dS m −1 ; air and water permeability depends on the sodium content; soils formed due to combined processes of salinization and alkalization; problems with sodium and other salts; leaching converts these soils into sodic soils.
Extent of Soil Salinization in India
Around 60% of the total geographical area of the country is cultivable (arable), of which nearly 80% (141 million ha) is under crops and about 6% (10 million ha) is under rangelands ( Mythili and Goedecke, 2016 ). The remaining arable lands are not cultivated. Nearly 147 million ha of land is subjected to soil degradation, including 94 million ha from water erosion, 23 million ha from salinity/alkalinity/acidification, 14 million ha from water-logging/ flooding, 9 million ha from wind erosion and 7 million ha from a combination of factors ( Bhattacharyya et al., 2015 ; Mythili and Goedecke, 2016 ).
Around 6.727 million ha area in India, which is around 2.1% of geographical area of the country, is salt-affected, of which 2.956 million ha is saline and the rest 3.771 million ha is sodic ( Arora et al., 2016 ; Arora and Sharma, 2017 ). Around 2.347 million ha of the salt-affected soils occur in the Indo-Gangetic plains of the country, of which 0.56 million ha are saline and 1.787 million ha are sodic ( Arora and Sharma, 2017 ). Nearly 75% of salt-affected soils in the country exist in the states of Gujarat (2.23 million ha), Uttar Pradesh (1.37 million ha), Maharashtra (0.61 million ha), West Bengal (0.44 million ha), and Rajasthan (0.38 million ha) ( Mandal et al., 2018 ).
Salt affected soils in India are spread in four major agriculturally important ecological regions in 15 states across the country and Andaman & Nicobar Islands, and they are:
i. Semi-arid Indo-Gangetic alluvial tract of Punjab, Haryana, UP, Delhi, parts of Bihar and West Bengal
ii. Arid and semi-arid tracts of Gujarat, Rajasthan, Madhya Pradesh, and Maharashtra
iii. Peninsular regions of Maharashtra, Karnataka, Tamil Nadu, Andhra Pradesh, and Orissa
iv. Coastal-alluvial region of Andhra Pradesh, Orissa, Tamil Nadu, Kerala, Karnataka, Maharashtra, Gujarat, and Island of Andaman & Nicobar.
The salt-affected soils in India broadly fall in two categories: sodic soils and saline soils. At certain places, with mean annual rainfall around 550 mm, saline-sodic soils are also found in the form of narrow band separating saline and sodic soils ( Arora and Sharma, 2017 ), but because their chemical properties and management are almost the same as the sodic soils, they are grouped with sodic soils category ( Qadir et al., 2007 ). Majority of the sodic soils occur in Indo-Gangetic region of India. They originate primarily due to weathering of rocks and minerals containing high sodium minerals, irrigation with groundwater containing excessive quantities of carbonates and bicarbonates, rise in groundwater table due to introduction of canal irrigation and salt laden run-off from the adjoining areas and un-drained basins. The saline soils are widespread in the canal irrigated arid and semi-arid regions. Table 2 shows the distribution of salt-affected soils in India.
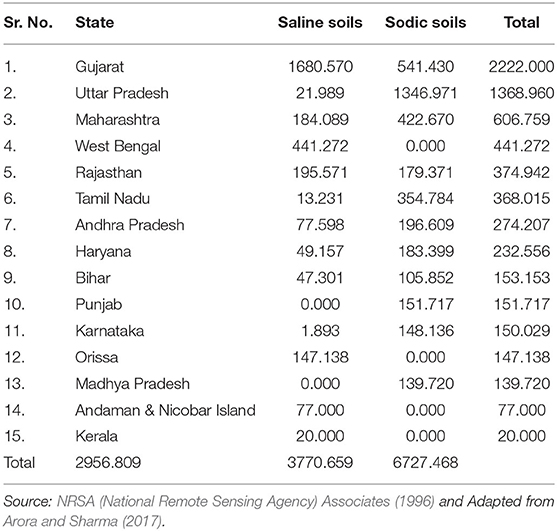
Table 2 . Extent of salt-affected soils in India (‘000 ha).
Mandal et al. (2018) distinguished salt-affected soils into three categories ( Table 3 ). According to these workers sodic, saline, and coastal saline soils are spread over 56, 25, and 19% area in the country ( Table 3 ). Sodic soils are confined in the Indo-Gangetic plains, arid and semi-arid region of western and central India, and Peninsular region in the southern India. Largest area under saline soils (71.2%) occurs in the state of Uttar Pradesh. More than 72% of coastal saline soils occur in the states of Gujarat and West Bengal. Largest area under sodic soils (35.6%) occurs in the state of Gujarat.
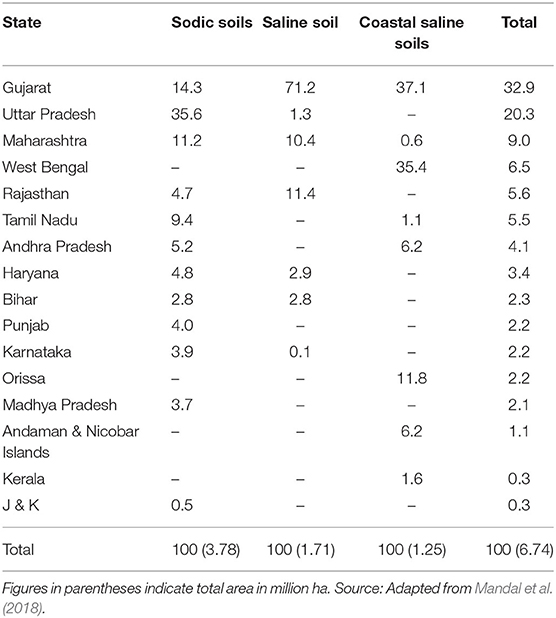
Table 3 . State-wise share (%) of salt-affected soils in India.
Introduction of the canal irrigation projects without proper provision of drainage has led to wide spread salinity in the country. Substantial salt-affected area occurs in different canal commands viz., Sharda Sahayak in Uttar Pradesh; Tungabhardra in Karnataka; Indira Gandhi Nahar Pariyojana (IGNP), Chambal and Tawa in Rajasthan and Madhya Pradesh; and Mahi and Ukai command area in Gujarat ( Mandal et al., 2010 ). Continuous seepage from the canals has resulted into rise in water tables and subsequent upward flux of salts to the surface, water-logging, formation of marshy lands, increased soil salinity, and decreased biodiversity. Two glaring examples include: (i) salinization of around 0.37 million ha area in Sharda Sahayak Canal Command region in Utter Pradesh within a span of three decades; (ii) salinization of around 0.18 million ha area in the Indira Gandhi Nahar Priyojana (IGNP) region in Rajasthan within few years of introduction of irrigation project ( Singh, 2009 ).
Use of brackish irrigation waters has caused secondary salinization in about 17% of irrigated lands in the country ( Shahid et al., 2018 ). Good quality irrigation water is scarce in the country. Increasing pressure of producing more food per unit available arable land forces for extensive use of brackish groundwater for irrigation. Ground water surveys indicate that poor quality waters being utilized in different states are 32–84% of the total groundwater development ( Minhas, 1999 ). Many more areas with good quality aquifers are endangered with contamination as a consequence of excessive withdrawals of groundwater.
The salinized areas in India continue to increase each year due to introduction of irrigation in new areas ( Patel et al., 2011 ). The rate of increase is around 10% annually ( Jamil et al., 2011 ). According to Sharma et al. (2014a) , unless preventive/ameliorative attempts are taken, the salt-affected areas are estimated to treble, i.e., increase from 6.74 to 16.2 million ha by 2050.
Delineation and digitization process of salt-affected soils in India is on. Fifteen salt- affected states have been mapped on 1:250,000 scale and digitization on 1:50,000 scale is in progress. The planning and execution of soil reclamation programmes by the policymakers and stakeholders are based on the state-wise data and maps of saline and sodic soils ( Mandal et al., 2010 ). The first approximation of water quality map of India has been published ( Sharma et al., 2014a ), adding great value in executing the plans effectively.
Ecological, Agricultural, and Social Concerns of Soil Salinization
The soil salinization has tremendous environmental, ecological, agricultural, and social impacts in terms of shrinkage of agricultural lands, low agricultural productivity, uncertain and unstable livelihood security, low economic returns, and poor quality of life. Excess salts in soil affect the metabolism of soil flora and fauna, leading ultimately to the destruction of all soil life, transforming fertile and productive lands into barren and desert lands. Soils are rendered useless agriculturally as well as for several other purposes (e.g., construction work). The salt accumulation damages existing infrastructure, farm machinery, waterways, roads etc. History records that soil salinization was partly responsible for the collapse of ancient civilizations like Mesopotamia, Nile Valley, Mohanzoadaro, and Indus Valley ( Dagar, 2005 ).
Salinity affects almost all aspects of plant development including germination, vegetative growth, and reproductive development due to drought and high soil salinity, and harsh environmental conditions ( Machado and Serralheiro, 2017 ). Plants in salt-affected environments experience two types of stress, the osmotic stress and nutrient stress. The osmotic stress is due to low osmotic potential of water in saline soils which adversely affects water absorption by plants. Nutrient stress is due to both toxicity (Na, Cl, B) and deficiency of plant nutrients (N, Ca, K, P, Fe, Zn). It also results in nutritional imbalances. Soil salinity significantly reduces phosphorus uptake by plants because phosphate ions precipitate with Ca ions ( Bano and Fatima, 2009 ). The enhanced Na + absorption in sodic soils reduces K + absorption which adversely affects the enzymatic activities involved in metabolic processes like photosynthesis and protein synthesis ( Hauser and Horie, 2010 ), which is detrimental for plant growth ( Katiyar-Agarwal et al., 2005 ). Reduced leaf area, chlorophyll content and stomatal conductance in salt-affected soils also affect photosynthesis ( Netondo et al., 2004 ).
Apart from high ESP and nutrient deficiencies and toxicities, other constraints for plant growth in sodic soils include poor soil physical conditions, viz. low water and air permeability, high runoff, low water holding capacity, surface crusting, and hard setting. They affect plant root penetration, seedling emergence, and tillage operations ( Murtaza et al., 2006 ).
Although salinization has strong implications on socio-economic aspects, yet very few publications are available in literature ( Shahid et al., 2018 ). Social consequences of soil salinization include decline in agricultural harvest, low income, change of livelihood options and related social constraints. The estimates show that the global annual cost of salt-induced land degradation in irrigated areas could be US$ 27.3 billion in terms of lost crop production ( Qadir et al., 2014 ). Annual global income loss due to salinization of irrigated lands has been estimated around US$ 12 billion ( Ghassemi et al., 1995 ). The inflation-adjusted cost of salt-induced land degradation in 2013 was estimated at US $ 441 per ha, with global economic losses pegged at US $ 27.3 billion per year ( Qadir et al., 2014 ).
The estimates based on 2012–14 moving average data suggest that due to soil salinization India loses annually 16.84 million tons of farm production (cereals, oilseeds, pulses, and cash crops) valued at Rs. 230.20 billion ( Mandal et al., 2018 ). It has strong implications on the national economy. The state of Uttar Pradesh topped the list with 7.69 million tons production loss, followed by Gujarat state with 4.83 million tons production loss. In terms of monetary loss, Gujarat topped the list with Rs. 100.63 billion loss, followed by Uttar Pradesh with Rs. 81.29 billion loss. Gujarat and Uttar Pradesh have the largest salt-affected area (>50% of cultivated area) in the country. These two states alone share around 79% monetary losses in the country. All these states deserve policy attention for management of salt-affected areas to reduce the crop production and monetary loss.
Peoples' living standard, daily life activities and socio-economic conditions are adversely affected. Farmers in response to salinity problem are forced to shift their livelihood strategies ( Ziaul Haider and Zaber Hossain, 2013 ). Farmers in salt-affected areas are generally resource constrained and require financial and technical assistance to sustain their livelihood efforts ( Oo et al., 2013 ).
Such degraded ecosystems, nevertheless, offer immense opportunities to harness the productivity potential through appropriate technological interventions. Even marginal to modest gains in crop yields in such soils would mean dramatic improvements in the lives of thousands of poor farmers in salinity affected regions in a country facing many challenges in agriculture.
Technological Interventions
After decades of experiments globally including ICAR-CSSRI, Karnal and several SAUs in India, understanding the problems of salt-affected soils, poor-quality irrigation waters, water dynamics, causes of salt accumulation and behavior of plants under salt stresses, recommendations have emerged as technologies for reclamation and management of salt-affected soils, viz. gypsum technology for reclamation of sodic soils, developing salt tolerant crop varieties, guidelines for use of poor quality waters, rehabilitation of salty lands using forestry species, etc. ( Mandal et al., 2018 ). There may be two approaches to tackle problem of soil salinity. One, to reclaim salt-affected soils; two, to manage salt-affected soils as they exist, i.e., without reclamation, using alternate suitable agricultural options such as cultivation of salt tolerant crops, saline aquaculture etc. The choice depends on the feasibility of reclamation and the cost effectiveness.
Not all salt-affected soils can be reclaimed practically and economically. While it is feasible to reclaim alkali and sodic soils by specific amendments and manage thereafter, the coastal salt-affected soils and black soils cannot be fully reclaimed. They require continuous soil and water management practices for their productive uses. Indian Council of Agricultural Research (ICAR)-Central Soil Salinity Research Institute (CSSRI) was established in India in 1969 to work exclusively on salt-affected soils. In addition, several State Agricultural Universities, especially those located in salt-affected regions, are also engaged in soil salinity research. Their efforts have resulted in the development of several technological interventions for the reclamation and management of salt-affected soils and use of poor quality water for irrigation in different agro-ecological zones of the country ( Sharma et al., 2011 ). The popularity of gypsum-based sodic soil reclamation, sub-surface drainage of water-logged saline lands, salt tolerant crop varieties and improved agroforestry techniques are a few laudable testimonies to the research credentials of these research Institutes.
Reclamation and Management of Saline Soils
Salt leaching with ponded fresh water, sub-surface drainage, mulching between two irrigations and during fallow period, irrigation management are some of the effective and well-known technological intervention to tackle the problems of water-logging and soil salinity ( Smedema and Ochs, 1998 ; Gupta, 2002 ; Arora and Sharma, 2017 ). The sub-surface drainage technology has been successfully adopted in Haryana, Rajasthan, Gujarat, Punjab, Andhra Pradesh, Maharashtra, Madhya Pradesh, and Karnataka, restoring around 110,000 ha waterlogged saline soils ( Sharma et al., 2014a ).
The adoption of sub-surface drainage technology in saline soils resulted in 3-fold increase in farmers' income. The yields of paddy, wheat and cotton increased by about 45, 111, and 215%, respectively ( Sharma et al., 2014a ). Besides, it significantly increased cropping intensity and socio-economic benefits in terms of on-farm employment generation ( Singh, 2009 ). The sub-surface drainage technology was able to generate around 128 man-days additional employment per ha per annum ( Sharma et al., 2011 ).
The cost of installation of sub-surface drainage system per hectare was estimated Rs. 74,000 for medium to coarse-textured soils with 67 m spacing and Rs. 1,15,000 for fine-textured black soils with 30 m spacing, with a benefit/cost ratio of around 2.71 ( Raju et al., 2016 ). The reclaimed area contributed about 0.56 million tons of foodgrains and an income generation of Rs. 8.60 million annually ( Raju et al., 2016 , 2017 ).
The technology is useful but constrained by bottlenecks like higher initial costs, operational difficulties, lack of community participation and the problems of safe disposal of drainage effluents, for the rapid adoption of this technology ( Singh, 2009 ). Successful implementation of sub-surface drainage projects demands a collective approach and responsibility duly supported by appropriate institutional arrangements ( Ritzema et al., 2008 ). In majority of the salinity affected regions, however, the community participation appears lacking as evidenced by the non-existent or non-functional water-user organizations for irrigation as well as drainage projects. It slows down the up-scaling of reclamation technologies in salt-affected areas.
Reclamation and Management of Sodic Soils
The technology package based on chemical amendments consists of the components such as land leveling, bunding, flushing, drainage for removal of excess water, good quality irrigation water, application of amendments, selection of crops and efficient nutrient management. Different chemical amendments used for the reclamation of sodic soils may be grouped into two categories: soluble calcium sources (e.g., gypsum, calcium chloride, and phospho-gypsum) and acids or acid formers (e.g., elemental sulfur, sulphuric acid, sulfates of iron and aluminum, pyrites and lime sulfur). Farmyard manure and pressmud are also used as amendments for reclaiming sodic soils. Chemical amendments require moisture (rainfall or irrigation) to activate the chemical processes that can reduce sodium levels or leach salts from the root zone. The organic amendments, on the other hand, are capable of alleviating problems associated with excessive salts or sodium without supplemental irrigation.
The amount and type of chemical amendments required for reclamation of sodic soils depend primarily on soil pH, EC, and ESP. Soluble calcium sources are recommended for use in non-calcareous soils while for calcareous soils acids or acid-formers are recommended. Gypsum followed by pyrites has emerged as the most preferred and acceptable chemical amendment for sodic soils in India due to their easy availability and low cost ( Abrol et al., 1988 ; Tyagi and Minhas, 1998 ). Pyrite was much less effective than gypsum ( Tyagi, 1998 ). The pyrites to be effective for reclamation must contain at least 5–6% soluble S ( Sharma and Swarup, 1990 ).
Gypsum requirement for restoring an alkali soil depends on the initial exchangeable sodium percentage (ESP), texture and mineralogy of soil, depth of soil to be reclaimed and tolerance of crops to sodicity. A good correlation exists between soil pH and gypsum requirement ( Abrol et al., 1973 ). Generally, 10–15 Mg ha −1 gypsum is required for the reclamation of alkali soils ( Abrol and Bhumbla, 1971 ).
The addition of organic materials in conjunction with gypsum hastens the reclamation process and also reduces the gypsum requirement ( Chorum and Rengasamy, 1997 ; Vance et al., 1998 ; Arora and Sharma, 2017 ). Addition of organic material increases soil microbial biomass while gypsum lowers soil pH ( Wong et al., 2009 ). Industrial byproducts such as phosphogypsum, pressmud, molasses, acid wash, and effluents from milk plants help in the reclamation of sodic soils by providing Ca directly or indirectly by dissolving soil lime ( Arora and Sharma, 2017 ). However, care should be taken that toxic elements like F, which is present in large quantities in products like phosphogypsum, are not added to soil ( Chhabra et al., 1980 ). The equivalent amounts of other amendments relative to gypsum are given in Table 4 .
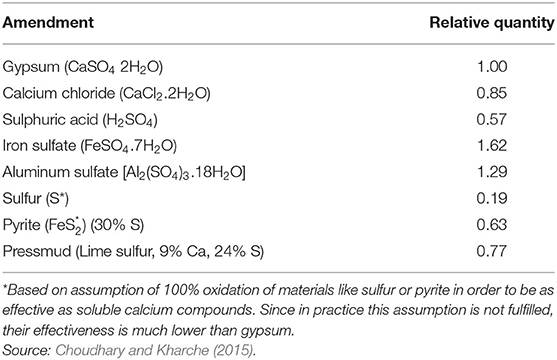
Table 4 . Equivalent quantities of some common amendments for sodic soil reclamation.
The gypsum-based alkali land reclamation technology has found large scale on-farm adoption in the country. Nearly 2.07 million ha of barren sodic soils have been brought under cultivation, contributing 16-17 million tons of paddy and wheat per annum to the country's food basket. Farmers are harvesting 5 t ha −1 of rice and 3 t ha −1 of wheat from third year onwards in such reclaimed lands ( Mandal et al., 2018 ). Besides, it also provides an employment opportunity to about 2.8 million man days annually. Financial viability for investment on gypsum technology has been found positive with benefit/cost ratio of 2.47 ( Tripathi, 2011 ). Sharma et al. (2011) calculated the economics for reclamation of sodic soils by considering 10% discount rate. The reclamation cost was estimated to be around Rs. 56,000/ha, with B:C of 1.52, internal rate of return 21.4% and payback period of 3 years.
Reclamation and Management of Coastal Saline Soils
A number of technologies have been standardized and perfected to restore coastal saline soils and sustain crop production in them. Rabi cropping in mono-cropped coastal saline soils, salt tolerant rice cultivars, rainwater harvesting in dugout farm ponds, integrated rice-fish culture and efficient nutrient management have been successfully practiced ( Sharma and Chaudhari, 2012 ). An innovative “ Doruvu ” technology has become popular in coastal regions of the country. The technology involves skimming of shallow depth fresh water floating on the saline water and storing in dug-out conical pits, locally called “ Doruvus .”
Multi-storeyed integrated agroforestry systems involving fish or shrimp culture, poultry, plantation crops, cattle, and diversified arable crops etc. seem to have potential in these areas. Khan et al. (2014) reported an average yield advantage of 20–30% over the existing rice yield of 2.9–3.3 t ha −1 by using biocompost @ 2–6 t ha −1 in sodic soils of Uttar Pradesh.
Initial cost on land excavation for constructing farm ponds, paddy-cum-fish culture and raised-sunken beds in coastal salt-affected areas was around Rs. 145, 136, and 88 thousand, respectively, with benefit/cost ratio of 1.20–1.58 ( Mandal et al., 2018 ). Such techniques in coastal areas of West Bengal increased cropping intensity from 114 to 186% which resulted in increase in farmers' income from Rs. 5,644 ha −1 (wet rice) to Rs. 1,43,982 ha −1 (wet rice-fish-vegetables) ( Mandal et al., 2017 ). Similar encouraging results were obtained through land modification technology (pond based and raised and sunken bed) under sodic soils in Uttar Pradesh ( Verma et al., 2012 ).
Phytoremediation of Salt-Affected Soils
Phytoremediation of salt-affected soils refers to the processes of removing excess salts from soil by growing different type of plants. Growing of salt tolerant trees, shrubs, and grasses is a cost-effective and environmental-friendly way of restoring salt-affected soils ( Mishra et al., 2003 ; Qadir et al., 2007 ). Different species of salt tolerant trees, shrubs, and grasses have been identified and put to use ( Table 5 ). Excellent reviews are available in literature on phytoremediation, e.g., Dagar (2014) for inland salt-affected lands, Dagar et al. (2014a) for coastal regions and Dagar and Minhas (2016) for use of poor-quality waters, etc.
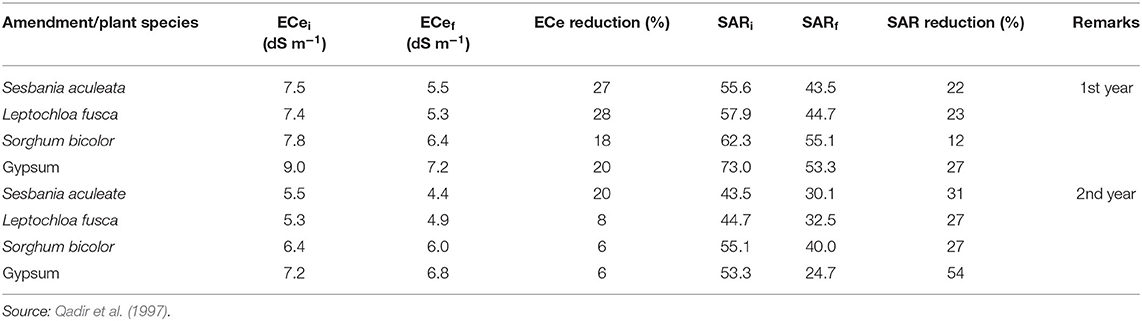
Table 5 . Soil ECe and SAR reduction through phytoremediation and chemical amendments using different plants ( i initial, f final).
Plants remove excess salts from soil through root absorption and accumulate them in their biomass, a process called phyto-accumulation or phyto-extraction . It decreases exchangeable sodium and soluble salt concentrations in soil. They also augment soil organic carbon and nutrient content thereby gradually improving physical (bulk density, porosity, infiltration, water holding capacity etc.), chemical (nutrient concentrations), and biological (microbial population) properties of soils and overall soil productivity ( Bhojvaid and Timmer, 1998 ; Kaur et al., 2000 ; Mishra et al., 2003 ; Nosetto et al., 2007 ; Qadir et al., 2007 ). Tree plantation, besides making degraded lands productive, provides fuel wood, and forage and helps in moderating climate change impacts through carbon sequestration ( Dagar, 2005 ; Sharma et al., 2011 ). It has been estimated that reforestation of 75 million ha degraded lands with suitable trees and grasses/crops has the potential to sequester carbon in above-ground as well as below-ground C biomass to the tune of about 4 Pg of carbon ( Dagar and Swarup, 2003 ).
Reclamation of sodic and saline waterlogged soils through afforestation and agroforestry practices is well established and documented ( Dagar, 2005 ; Dagar et al., 2014b ; Dagar and Minhas, 2016 ). Some of the promising species for sodic soil reclamation include Prosopis juliflora, Acacia nilotica, Casuarina equisetifolia, Tamarix articulate, Eucalyptus tereticornis , and Leptochloa fusca ( Singh et al., 1994 ; Dagar et al., 2016 ), and for waterlogged saline soils include Prosopis juliflora, Tamarix articulata, Casuarina glauca, Acacia farnesiana, Acacia nilotica, Acacia tortilis , and Parkinsonia aculeata ( Dagar and Tomar, 2002 ). Plant species like Eucalyptus tereticornis, Populus deltoids , and Tectona grandis are effective for reclaiming sodic soils ( Singh et al., 1994 ).
Dagar et al. (2001a) used raised-sunken bed technology to successfully establish trees like pomegranate (fruit tree) and Salvadora persica (a non-edible oil yielding tree) on sodic soils. These trees were grown on raised beds to avoid damage due to water stagnation. In highly sodic soils of semiarid regions, having kankar pan in upper 2-m soil layer, Dagar et al. (2001b) used auger hole planting technique for successfully planting the forest tree species like Tamarix articulata, Prosopis juliflora , and Acacia nilotica .
Many grass species suited for sodic soils have been identified but all of them could not find field application due to the reason that they absorb and accumulate sodium and other toxic elements in their foliage and, thus, are unfit as fodder. Efforts are on to identify and popularize those grass species which can retain high proportion of sodium in their root system, rendering the shoots palatable for cattle. However, Biswas and Biswas (2014) have advocated that most of the field crops are less tolerant than grasses to alkali environment. Para grass ( Brachiaria mutica ), rhodes grass ( Chloris gayana ), matricaria ( Matricaria recutila ), Karnal grass ( Leptochloa fusca ) have been found the most promising grass spp. suitable for alkali soils. Aeluropus lagopoides, Chloris barbata, Echinocloa colonum, Dicanthium annulatum, Sporobolus helvolus, Phragmites spp., and Sida spp. have been identified the other promising grasses for rehabilitation of saline soil. Large tracts of salt-affected community and government lands lying barren have been restored and put to best productive use through adoption of agroforestry techniques and agronomic practices.
Promising agro-forestry models, fruit-based agro-forestry models, silvi-pastoral models etc. along with appropriate planting and management techniques have been developed specifically for saline/sodic/saline-sodic etc. conditions ( Singh et al., 1994 ; Dagar et al., 2008 , 2015 ; Sharma et al., 2014b ). Under saline irrigation conditions medicinal and aromatic plants such as isabgol ( Plantago ovata ), aloe ( Aloe barbadensis ), kalmeg ( Andrographis paniculata ), Matricaria chamomilla, Vetiveria zizanioides, Cymbopogon martini , and Cymbopogon flexuosus have been found to produce high biomass ( Tomar et al., 2003a , b ; Dagar et al., 2004 , 2006 ; Tomar and Minhas, 2004 ).
Salt-affected Vertisols (i.e., black cotton soils) are difficult and tedious to restore compared to alluvial sandy loam soils of Indo-Gangetic Plains. The high clay content of these soils makes them vulnerable even at low salt and exchangeable sodium concentrations. Major chunk (about 1.21 million ha) of salt-affected black cotton soils (Vertisols) is found in Gujarat. Such soils also occur in appreciable extent in Karnataka, Maharashtra, and Rajasthan. Commercial cultivation of salt tolerant plants like Salvadora persica (a halophyte, non-edible oil tree), dill ( Anethum graveolens ) a spice crop, industrial species like Euphorbia and Mulethi ( Glycyrrhiza glabra ), castor and sunflower has been found useful in reclaiming these soils and have largely been practiced by the farmers ( Rao et al., 2000 , 2003 ; Sharma and Chaudhari, 2012 ; Arora et al., 2013 ).
Large stretches of canal irrigated lands in many arid and semi-arid regions have become unproductive due to water-logging and the subsequent secondary salinization. Water seepage from canals and faulty on-farm water management practices together create shallow water table conditions. Higher capillary salinization in such areas has caused significant increase in root zone salinity ( Chhabra and Thakur, 1998 ). The conventional approaches to reclaim such lands are expensive, difficult to operate and pose problems in the safe disposal of saline drainage effluents and so have necessitated interest in other viable alternatives such as bio-drainage ( Chhabra and Thakur, 1998 ; Ram et al., 2011 ). Analogous to energy-operated water pumps, bio-drainage is a proven technology to arrest salinity build-up in canal commands with growing of suitable tree species (e.g. eucalyptus, poplar, and bamboo) ( Singh, 2009 ). Efforts are on in exploring combined applications of bio-drainage and suitable land modifications to effectively utilize the water-logged salt-affected soils ( Sharma et al., 2011 ).
Bio-Remediation
The bio-remediation approach, which involves plant-microbial interaction, has received increased attention worldwide for enhancing productivity of salt-affected soils ( Arora et al., 2014 ). The microorganisms have the capability of rapid adjustment toward environmental changes and deterioration, and thus can play an important role in the maintenance and sustainability of any ecosystem. Microorganisms possess some unique properties such as salt stress tolerance, genetic diversity, synthesis of compatible solutes, production of plant growth promoting hormones, bio-control potential, and their interaction with crop plants. If these traits are suitably exploited, microorganisms can play a significant role in alleviating salt effects on crop plants ( Shrivasata and Kumar, 2015 ).
Microorganisms present in the rhizosphere could promote plant growth and yield in salt stress environment in different ways, directly and indirectly ( Dimkpa et al., 2009 ). For example, some plant growth-promoting rhizobacteria may directly stimulate plant growth and development by providing plants with fixed nitrogen, phytohormones, iron (sequestered by bacterial siderophores), and soluble phosphate ( Hayat et al., 2010 ), while others may indirectly benefit plants by protecting them against soil-borne diseases, mostly caused by pathogenic fungi ( Lutgtenberg and Kamilova, 2009 ) by inducing cell wall structural modifications, biochemical and physiological changes leading to synthesis of proteins and chemicals involved in plant defense mechanisms ( Arora and Sharma, 2017 ).
Halophilic bacteria have the potential to remove sodium ions from soil and increase metabolic and enzymatic activities in plants. Arora et al. (2016) used halophilic bacteria for the remediation of saline and sodic soils. In a field experiment, bio-inoculation of wheat seeds with halophilic bacteria increased grain and straw yield of wheat in a sodic soil by 18.1 and 24.2%, respectively. The bacterial inoculation improved soil properties by decreasing soil pH from 9.4 to 8.6, increasing microbial biomass C from 82 to 137 μg/g. Similarly, in a pot experiment irrigated with saline water (5% NaCl), inoculation of halophilic bacterial consortium increased fresh weight, dry weight, shoot length, and root length of maize plants by 194.5, 98.97%, 15.37 and 7.4 cm, respectively, compared to the uninoculated control plants. Arora et al. (2014) could enhance wheat yield by 10–12% in salt-affected soil (EC = 156 dS/m) by using phosphatic solublizing bacteria and Rhizobium strains.
A low-cost microbial bio-formulation “CSR-BIO,” a consortium of Bacillus pumilus, Bacillus thuringenesis , and Trichoderma harzianum , is rapidly becoming popular with the farmers in many states ( Damodaran et al., 2013 ). This bio-formulation acts as a soil conditioner and nutrient mobilizer and has been found to increase the productivity of the high value crops such as banana, vegetables, and gladiolus in sodic and normal soils by 22–43%.
Cultivation of Salt Tolerant Crops and Crop Varieties
Cultivation of salt tolerant crops and crop varieties is another way to address the problem of soil salinization. This technique is viable and cost effective and suits well to the small and marginal farmers who without financial support are unable to bear the high costs of chemical amendment-based reclamation technologies. Salt tolerant varieties of rice, wheat, mustard, and other crops, grasses, shrubs, fruit trees, and medicinal and aromatic plants have been developed/identified for commercial cultivation in salt-affected soils ( Singh, 2009 ; Sharma et al., 2011 ). The relative tolerance of some crops to total salinity (EC) and sodicity (ESP) is shown in Tables 6 , 7 , respectively.
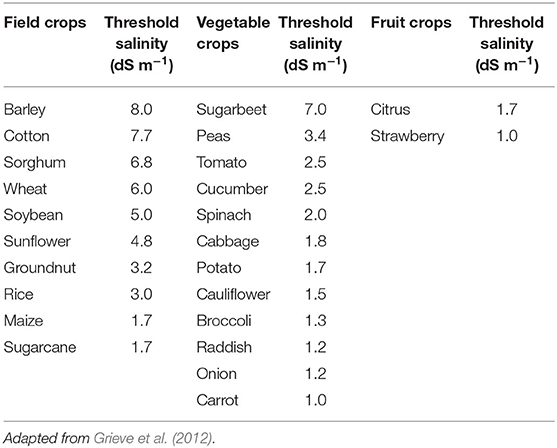
Table 6 . Relative tolerance of some crops to salinity (EC).
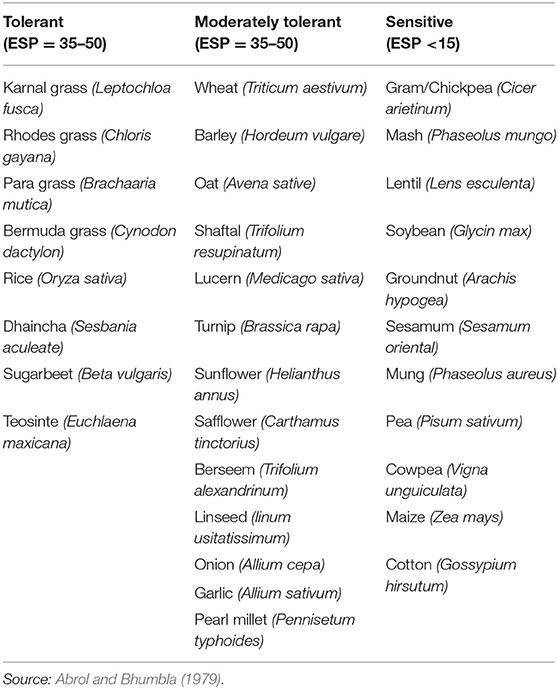
Table 7 . Relative tolerance of some crops to soil sodicity (ESP).
Use of salt tolerant varieties of field crops is another practical option to manage salt-affected soils with the poor farmers, especially small and marginal, for whom chemical amendment technologies are not feasible without Government subsidies ( Arora and Sharma, 2017 ). Several varieties of important field crops like rice, wheat and mustard, having potential to yield reasonable economic returns in saline and sodic soils, have been developed ( Singh and Sharma, 2006 ).
Cultivation of salt tolerant multipurpose trees, bushes, and grasses, fruit plants, medicinal and aromatic plants etc. on salt-affected village community lands, road-side lands, lands along the railway tracks, Government lands etc. is another opportunity of managing salt-affected soils ( Singh et al., 1994 ; Minhas et al., 1997 ; Tomar et al., 2003b ).
Tissue culture techniques find usefulness in developing suitable salt-tolerant trees and crops of high economic value.
It may be noted, however, that crop production on salt-affected soils is generally costlier and crop yields are usually low, net returns are low and the risk of crop failures continues even after suitable amendments are provided ( Minhas and Sharma, 2003 ).
Saline Aquaculture
Inland saline aquaculture (land-based aquaculture using saline groundwater) is being commercially practiced in many saline tracts of Australia, Israel, and USA ( Allan et al., 2009 ). This knowledge was used in India also to make the saline water-flooded soils profitable. The experience in many parts of south-western Haryana and Punjab have shown that the degraded soil and water resources could be put to profitable use by shrimp and fish farming ( Purushothaman et al., 2014 ). At Nain Experimental Farm, Panipat, Haryana (India), under conditions of high salinity of pond water (25 dS m −1 ), low water availability and high evaporation losses, fish growth of about 400–600 g in 6-month and 600–800 g in 1-year period was observed ( CSSRI, 2013 ).
In coastal areas of Andhra Pradesh, many farmers have converted their rice fields into brackish water fish farms for reason of high remuneration from aquaculture. They store brackish water, drawn from the sea through creeks and drains, in big tanks for raising high value prawns. Estimates suggest that nearly 0.2 million ha is under saline aquaculture in the coastal districts of Andhra Pradesh. Many small and marginal farmers (>50%), however, found this technology (prawn farming) highly risky with unstable returns, and hence abandoned after few years ( Singh, 2009 ).
Multi-Enterprise Agriculture Models
Integrated multi-enterprise models comprising different components, viz. field and horticultural crops, fishery, cattle, poultry, and beekeeping etc. are being developed and tested to address the specific needs of small and marginal farmers especially in post reclamation phase. The models have been standardized for specific conditions such as saline soils of Haryana, water-logged sodic soils of Uttar Pradesh, highly saline black soils of Gujarat and coastal saline soils of West Bengal ( Singh, 2009 ; Sharma and Chaudhari, 2012 ). The aim is to sustain resource use efficiency, high and regular income and employment generation to the farmers. The models drastically reduce the production costs by synergistic recycling of resources among different components.
A multi-enterprise model developed and evaluated by ICAR-CSSRI Karnal for reclaimed sodic land generates net annual income of Rs. 2.65 lakh ( Chinchmalatpure et al., 2015 ). The model on daily basis generates a gross income of Rs. 400–700 and net income of Rs. 250–500 from about 1.0 ha land area when fisheries, dairy, horticulture, poultry, duckery, and mushroom cultivation are integrated and by-products of these enterprises are recycled within the system. Biogas produced (2 m 3 per day) in the Model adequately meets the energy requirements of farmer's family.
Reclamation of Salt-Affected Soils and Food Security in India
It is estimated that due to soil salinization India loses around Rs. 230.20 billion annually in terms of crop production loss to the tune of 16.84 million tons ( Mandal et al., 2018 ). The Indian Government, therefore, has attached highest priority to the policy planning for the reclamation of degraded lands, including salt-affected soils in the country. The Indian Government is keen to restore 26 million ha of degraded lands by the year 2030 in order to ensure food security in the country. Significant research efforts have been made during the last 4 decades with encouraging results. The response of the farming community in salt-affected regions is overwhelming.
Sharma and Chaudhari (2012) reported reclamation of 1.5 million ha of salt-affected soils in the country, with addition of around 15 million tons of food grains to the national food basket annually. It provided additional income of around Rs. 13.5 billion per annum, and also generated 8.33 million man-days per year in terms of on-farm and off-farm rural employment opportunities. According to a recent publication of Mandal et al. (2018) , around 2.18 million ha salt-affected soils (0.11 million ha saline soils and 2.07 million ha sodic soils) have been reclaimed in India. The reclamation has been achieved through gypsum technology in saline soils and sub-surface drainage technology in sodic soils. It has contributed an estimated 17.16 million tons of food-grains per annum (16.6 million tons from saline soils and 0.56 million tons from sodic soils) to the national food basket, with additional income of as high as Rs. 15.5 billion annually ( Mandal et al., 2018 ).
The technological interventions on other aspects of salt-affected soils such as alternate land-use systems, saline aquaculture, cultivation of salt tolerant crop varieties, agroforestry, phytoremediation, bioremediation etc. have proved their worth by positively influencing food and nutritional security, women empowerment, involvement of landless laborers and minimizing rural migration, besides restoration of the ecological balance by its positive impact on environment ( Sharma and Chaudhari, 2012 ).
Soil salinization is a serious problem challenging food security in India. It is a dynamic process caused by several natural and human-induced processes, and quite often, the socio-economic and political considerations become extremely important in accelerating the processes of soil salinization. Many times, such factors are beyond the control of individual farmers and call for the attention of the policy makers. It becomes the responsibility of respective governments to take appropriate policy decisions and corrective measures in order to keep a check on soil salinization and also to restore the soils already affected by salts.
Several on-farm tested technologies are available for the reclamation and management of salt-affected soils. The efforts put-in by Government agencies and farmers for the reclamation and rehabilitation of salt-affected soils in the country so far have been encouraging. Nevertheless, in order to achieve the target of reclamation of 26 million ha of salt-affected soils, concerted efforts are needed by all the stakeholders. The site-specific restoration programmes be conceived and implemented in mission mode with the genuine participation of the local farmers. The farmers need to be incentivized rather than subsidized to undertake corrective measures. Under the scenario where the cultivable lands are shrinking due to increased urbanization, the restoration and management of salt-affected soils offer a potential hope of land expansion and production enhancement for future food security in the country.
Author Contributions
PK contributed in conception and first draft preparation. PKS reviewed, analyzed, and provided interpretation. All authors listed have made a substantial, direct and intellectual contribution to the work, and approved it for publication.
Conflict of Interest
The authors declare that the research was conducted in the absence of any commercial or financial relationships that could be construed as a potential conflict of interest.
Abrol, I. P., and Bhumbla, D. R. (1971). Saline and Alkali Soils in India: Their Occurrence and Management . FAO World Soil Research Report. 41, FAO, Rome.
Abrol, I. P., and Bhumbla, D. R. (1979). Crop responses to differential gypsum applications in highly sodic soil and the tolerance of several crops to exchangeable sodium under field conditions. Soil Sci. 127, 79–85. doi: 10.1097/00010694-197902000-00004
CrossRef Full Text | Google Scholar
Abrol, I. P., Dargan, S. K., and Bhumbla, D. R. (1973). Reclaiming Alkali Soils. Bulletin No. 2. Central Soil Salinity Research Institute, Karnal, 58.
Google Scholar
Abrol, I. P., Yadav, Y. S., and Massoud, F. I. (1988). Salt-Affected Soils and Their Management , Soils Bulletin. No. 39. FAO, Rome, 131.
Aggarwal, P. K., Joshi, P. K., Ingram, J. S. I., and Gupta, R. K. (2004). Adapting food systems of the Indo-Gangetic plains to global environmental change: key information needs to improve policy formulation. Environ. Sci. Policy 7, 487–498. doi: 10.1016/j.envsci.2004.07.006
Allan, G. L., Fielder, D. S., Fitzsimmons, K. M., Applebaum, S. L., and Raizada, S. (2009). Inland saline aquaculture. New Technol. Aquac. 6, 1119–1147. doi: 10.1533/9781845696474.6.1119
Arora, S., Bhuva, C., Slanki, R. B., and Rao, G. G. (2013). Halophytes for bio-saline agro-forestry and phytoremediation of coastal saline lands. J. Soil Water Conserv. 12, 252–259.
Arora, S., and Sharma, V. (2017). Reclamation and management of salt-affected soils for safeguarding agricultural productivity. J. Safe Agri. 1, 1–10.
Arora, S., Singh, Y. P., Vanza, M., and Sahni, D. (2016). Bioremediation of saline and sodic soils through halophilic bacteria to enhance agricultural production. J. Soil Water Conserv. 15, 302–305. doi: 10.5958/2455-7145.2016.00027.8
Arora, S., Vanza, M., Mehta, R., Bhuva, C., and Patel, P. (2014). Halophilic microbes for bio-remediation of salt-affected soils. African J. Microbio. Res. 8, 3070–3078. doi: 10.5897/AJMR2014.6960
Bano, A., and Fatima, M. (2009). Salt tolerance in Zea mays (L.) following inoculation with Rhizobium and Pseudomonas. Biol. Ferti. Soils. 45, 405–413. doi: 10.1007/s00374-008-0344-9
Bhattacharyya, R., Ghosh, B. N., Mishra, P. K., Mandal, B., Rao, C. S., Sarkar, D., et al. (2015). Soil degradation in India: Challenges and potential solutions. Sustainability 7, 3528–3570. doi: 10.3390/su7043528
Bhojvaid, P. P., and Timmer, V. R. (1998). Soil dynamics in an age sequence of Prosopis juliflora planted for sodic soil restoration in India. Fores. Eco. Manage. 106, 181–193. doi: 10.1016/S0378-1127(97)00310-1
Biswas, A., and Biswas, A. (2014). Comprehensive approaches in rehabilitating salt affected soils: a review on Indian perspective. Open Trans. Geosci. 1, 13–24. doi: 10.15764/GEOS.2014.01003
Bresler, E., McNeal, B. L., and Carter, D. L. (1982). Saline and Soidic Soils: Principles-Dyanamics-Modeling . New York, NY: Springer-Verlag, Berlin Heidelberg, 227.
Chhabra, R., Abrol, I. P., Dargan, K. S., and Gaul, B. L. (1980). Save on phosphatic fertiliozers in the initial years of alkali soils reclamation. Indian Farm. 30, 13–15.
Chhabra, R., and Thakur, N. P. (1998). Lysimeter study on the use of biodrainage to control waterlogging and secondary salinization in (canal) irrigated arid/semi-arid environment. Irri. Drain. Sys. 12, 265–288. doi: 10.1023/A:1006104428674
Chinchmalatpure, A. R., Ali, S., Kulshrestha, N., Singh, R. K., Bundela, D. S., Kumar, P., et al. (2015). Intellectual Property Management and Commercialization of ICAR-CSSRI Technologies for Management of Salt-Affected and Waterlogged Soils of India . Karnal: ICAR-Central Soil Salinity Research Institute, 62.
Chorum, M., and Rengasamy, P. (1997). Carbonate chemistry, pH and physical properties of alkaline sodic soil as affected by various amendments. Aust. J. Soil Res. 35, 149–161. doi: 10.1071/S96034
Choudhary, O. P., and Kharche, V. K. (2015). “Soil salinity and sodicity,” in Soil Science: An Introduction (New Delhi: Indian Society of Soil Science), 353–384. Available online at: http://www.isss-india.org/index.aspx
CSSRI (2013). Annual Report, 2013–14. Central Soil Salinity Research Institute, Karnal.
Dagar, J. C. (2005). Salinity research in India: an overview. Bull. Nat. Inst. Eco. 15, 69–80.
Dagar, J. C. (2014). “Greening salty and waterlogged lands through agroforestry systems for livelihood security and better environment,” in Agroforestry System in India: Livelihood Security & Ecosystem Services, Advances in agroforestry , Vol 10, eds J. C. Dagar, A. K. Singh, and A. Arunachalam (New Delhi: Springer), 273–332.
Dagar, J. C., Khajanchi, L., Jeet, R., Mukesh, K., Chaudhary, S. K., Yadav, R. K., et al. (2016). Eucalyptus geometry in agroforestry on waterlogged saline soils influences plant and soil traits in North-West India. Agric. Ecosyst. Environ. 233, 33–42. doi: 10.1016/j.agee.2016.08.025
Dagar, J. C., and Minhas, P. S. (2016). Agroforestry for the management of waterlogged saline soils and poor-quality waters. Adv. Agron. 13:210. doi: 10.1007/978-81-322-2659-8
Dagar, J. C., Pandey, C. B., and Chaturvedi, C. S. (2014a). “Agroforestry: a way forward for sustaining fragile coastal and island agro-ecosystems,” in Agroforestry System in India: Livelihood Security & Ecosystem Services, Vol. 10. eds J. C. Dagar, A. K. Singh, and A. Arunachalam (New Delhi: Springer), 185–232.
Dagar, J. C., Sharma, H. B., and Shukla, Y. K. (2001a). Raised and sunken bed technique for agroforestry on alkali soils of northwest India. Land Degrad. Dev. 12, 107–118. doi: 10.1002/ldr.442
Dagar, J. C., Singh, A. K., and Arunachalam, A. (2014b). Agroforestry system in India: livelihood security & ecosystem services. Adv. Agron. 10:400. doi: 10.1007/978-81-322-1662-9
CrossRef Full Text
Dagar, J. C., Singh, G., and Singh, N. T. (2001b). Evaluation of forest and fruit trees used for rehabilitation of semiarid alkali-sodic soils in India. Arid Land Res. Manag. 15, 115–133. doi: 10.1080/15324980151062742
Dagar, J. C., and Swarup, A. (2003). “Potential of afforestation and agroforestry in carbon sequestration for mitigating climate changes,” in Agroforestry: Potentials and Opportunities , eds P. S. Pathak and R. Newaj (Jhansi: Agrobios (India) and Indian Society of Agroforestry), 43–63.
Dagar, J. C., and Tomar, O. S. (2002). “Utilization of salt-affected soils & poor quality waters for sustainable biosaline agriculture in arid and semiarid regions of India,” in Proceedings of the ISCO Conference (Beijing).
Dagar, J. C., Tomar, O. S., and Kumar, Y. (2006). Cultivation of medicinal isabgol ( Plantago ovate ) in different alkali soils in semi-arid regions of northern India. Land Degrad. Dev. 17, 275–283. doi: 10.1002/ldr.700
Dagar, J. C., Tomar, O. S., Kumar, Y., and Yadav, R. K. (2004). Growing three aromatic grasses in different alkali soils in semi-arid regions of northern India. Land Degrad. Dev. 15, 143–151. doi: 10.1002/ldr.598
Dagar, J. C., Tomar, O. S., Minhas, P. S., and Singh, G. (2008). Dryland Biosaline Agriculture- Hisar Experience. Technical Bulletin No. 6/2008. Central Soil Salinity Research Institute, Karnal, 28.
Dagar, J. C., Yadav, R. K., Tomar, O. S., Minhas, P. S., Yadav, G., and Lal, K. (2015). Fruit-based agroforestry systems for saline water-irrigated semi-arid hyperthermic camborthids soils of north-west India. Agroforest. Syst. 90, 1123–1132. doi: 10.1007/s10457-015-9889-4
Damodaran, T., Rai, R. B., Jha, S. K., Sharma, D. K., Mishra, V. K., Dhama, K., et al. (2013). Impact of social factors in adopon of CSRBIO- A cost effective, eco-friendly bio-growth enhancer for sustainable crop production. South Asian J. Exper. Bio. 3, 158–165.
Dantwala, M. L. (1986). Strategy of agricultural development since independence, in Indian Agricultural Development Since Independence , eds M. L. Dantwala et al. (New Delhi: Oxford and lBH Publishing Co. Pvt. Ltd), l–15.
Dimkpa, C., Weinand, T., and Ash, F. (2009). Plant-rhizobacteria interactions alleviate abiotic stress conditions. Plant Cell Environ. 32, 1682–1694. doi: 10.1111/j.1365-3040.2009.02028.x
PubMed Abstract | CrossRef Full Text | Google Scholar
Doering, E. J., and Sandoval, F. M. (1976). Hydrology of saline seeps in the Northern Great Plains. Trans. ASEA . 19, 856–861. doi: 10.13031/2013.36134
Elobeid, A., Jensen, H. H., and Smith, L. C. (2000). The geography and causes of food insecurity in developing countries. Agri. Econ. 22, 199–215. doi: 10.1111/j.1574-0862.2000.tb00018.x
Foley, J. A., Defries, R., Asner, G. P., Barford, C., Bonan, G., Carpenter, S. R., et al. (2005). Global consequences of land use. Science 309, 570–574. doi: 10.1126/science.1111772
Ghassemi, F., Jakeman, A. J., and Nix, H. A. (1995). Salinisation of Land and Water Resources: Human Causes, Extent, Management and Case Studies . Wallingford: CABI Publishing.
Glick, B. R., Cheng, Z., Czarny, J., and Duan, J. (2007). Promotion of plant growth by ACC deaminase-producing soil bacteria. Eur. J. Plant Pathol. 119, 329–339. doi: 10.1007/s10658-007-9162-4
Godfray, H. C. J., Beddington, J. R., Crute, I. R., Haddad, L., Lawrence, D., Muir, J. F., et al. (2010). Food security: the challenge of feeding 9 billion people. Science 327, 812–818. doi: 10.1126/science.1185383
Grieve, C. M., Grattan, S. R., and Maas, E. V. (2012). “Plant Salt Tolerance,” in ASCE Manual and Reports on Engineering Practice No. 71 Agricultural Salinity Assessment and Management, 2nd Edn . eds W. W. Wallender and K. K. Tanji (Reston, VA: ASCE), 405–459.
Gupta, R. K., and Abrol, I. P. (1990). “Salt-affected soils: their reclamation and management for crop production,” in Advances in Soil Science, Vol. 11 . eds R. Lal and B. A. Stewart (New York, NY: Springer), 223–288. doi: 10.1007/978-1-4612-3322-0_7
Gupta, S. K. (2002). A century of subsurface drainage research in India. Irri. Drain. Sys. 16, 69–84. doi: 10.1023/A:1015525405522
Halawar, S. V. (2019). The trend analysis of major food grains in India. Int. J. Curr. Microbio. Appl. Sci. 8, 353–360. doi: 10.20546/ijcmas.2019.803.044
Hauser, F., and Horie, T. (2010). A conserved primary salt tolerance mechanism mediated by HKT transporters: a mechanism for sodium exclusion and maintenance of high K + /Na + ratio in leaves during salinity stress. Plant Cell Enviroin. 33, 552–565. doi: 10.1111/j.1365-3040.2009.02056.x
Hayat, R., Ali, S., Amara, U., Khalid, R., and Ahmed, I. (2010). Soil beneficial bacteria and their role in plant growth promotion: a review. Ann. Microbiol. 60, 579–598. doi: 10.1007/s13213-010-0117-1
Jamil, A., Riaz, S., Ashraf, M., and Foolad, M. R. (2011). Gene expression profiling of plants under salt stress. Crit. Rev. Plant Sci. 30, 435–458. doi: 10.1080/07352689.2011.605739
Katiyar-Agarwal, S., Verslues, P., and Zhu, J. K. (2005). “Plant nutrition for food security, human health and environmental protection,” in Mechanisms of Salt Tolerance in Plants , eds C. J. Li et al., (Beijing: Tsinghua University Press) 44–45.
Kaur, B., Gupta, S. R., and Singh, G. (2000). Soil carbon, microbial activity and nitrogen availability in agroforestry systems on moderately alkaline soils in northern India. Appl. Soil Ecol. 15, 283–294. doi: 10.1016/S0929-1393(00)00079-2
Khan, A. H., Singh, A. K., Mubeen Singh, S., Zaidi, N. W., Singh, U. S., et al. (2014). Response of salt-tolerant rice varieties to biocompost application in sodic soil of eastern Uttar Pradesh. Am. J. Plant Sci. 5, 7–13. doi: 10.4236/ajps.2014.51002
Kumar, P., Joshi, P. K., and Mittal, S. (2016). Demand vs supply of food in India - futuristic projection. Proc. Indian Nat. Sci. Acad. 82, 1579–1586. doi: 10.16943/ptinsa/2016/48889
Kumar, P., Mruthyunjaya, and Dey, M. M. (2007). Long-term changes in food basket and nutrition in India. Econ. Polit. Wkly. 42, 3567–3572. doi: 10.2307/40276502
Lotze-Campen, H., Müller, C., Bondeau, A., Rost, S., Popp, A., and Lucht, W. (2008). Global food demand, productivity growth, and the scarcity of land and water resources: a spatially explicit mathematical programming approach. Agric. Econ. 39, 325–338. doi: 10.1111/j.1574-0862.2008.00336.x
Lutgtenberg, B., and Kamilova, F. (2009). Plant-growth-promoting rhizobacteria. Annu. Rev. Microbiol. 63, 541–556. doi: 10.1146/annurev.micro.62.081307.162918
Machado, R. M. A., and Serralheiro, R. P. (2017). Soil salinity: effect on vegetable crop growth. management practices to prevent and mitigate soil salinization. Horticulturae 3:30. doi: 10.3390/horticulturae3020030
Mandal, A. K., Sharma, R. C., Singh, G., and Dagar, J. C. (2010). Computerized Database on Salt-Affected Soil in India . Technical Bulletin. CSSRI, Karnal, 28.
Mandal, S., Burman, D., Mandal, U. K., Lama, T. D., Maji, B., and Sharma, P. C. (2017). Challenges, options and strategies for doubling farmers' income in West Bengal- reflections from coastal region. Agri. Eco. Res. Rev. 30, 89–100. doi: 10.5958/0974-0279.2017.00024.6
Mandal, S., Raju, R., Kumar, A., Kumar, P., and Sharma, P. C. (2018). Current status of research, technology response and policy needs of salt-affected soils in India – a review. Ind. Soc. Coastal Agri. Res. 36, 40–53.
Mashali, A. M. (1995). “Integrated soil management for sustainable use of salt-affected soils and network activities,” in Proceedings of the International Workshop on Integrated Soil Management for Sustainable Use of Salt-Affected Soils (Manila: Bureau of Soils and Water Management), 55–75.
Mekonnen, M. M., and Hoekstra, A. Y. (2010). A global and high-resolution assessment of the green, blue and grey water footprint of wheat. Hydrol. Earth Syst. Sci. 14, 1259–1276. doi: 10.5194/hess-14-1259-2010
Minhas, P. S. (1999). “Use of Poor-quality Waters,” in 50 Years of Natural Resource Management Research , eds G. B. Singh and B. R. Sharma (Karnal: Central Soil Salinity Research Institute), 327–346.
PubMed Abstract
Minhas, P. S., and Sharma, O. P. (2003). Management of soil salinity and alkalinity problems in India. J. Crop Prod. 7, 181–230. doi: 10.1300/J144v07n01_07
Minhas, P. S., Singh, Y. P., Tomar, O. S., Gupta, R. K., and Gupta, R. K. (1997). Saline water irrigation for the establishment of furrow planted trees in north western India. Agrofor. Syst. 35, 177–186. doi: 10.1007/BF00122778
Mishra, A., Sharma, S. D., and Khan, G. H. (2003). Improvement in physical and chemical properties of sodic soil by 3, 6 and 9 years old plantation of Eucalyptus tereticornis : Biorejuvenation of sodic soil. Forest Eco. Manage. 184, 115–124. doi: 10.1016/S0378-1127(03)00213-5
Mittal, S. (2007). What affect changes in cereal consumption? Econ. Polit. Weekly , 444–447.
Murtaza, G., Ghafoor, A., and Qadir, M. (2006). Irrigation and soil management strategies for using saline-sodic water in a cotton-wheat rotation. Agric. Water Manage. 81, 98–114. doi: 10.1016/j.agwat.2005.03.003
Mythili, G., and Goedecke, J. (2016). “Economics of Land Degradation in India,” in Economics of Land Degradation and Improvement- A Global Assessment for Sustainable Development , eds E. Nkonya, A. Mirzabaev, and J. von Braun (Cham: Springer), 431–469.
Narain, D. (1977). Growth and productivity in Indian agriculture. Ind. J. Agric. Econ. 32, 1–44.
Netondo, G. W., Onyango, J. C., and Beck, E. (2004). Sorghum and salinity: II. Gas exchange and chlorophyll fluorescence of sorghum under salt stress. Crop Sci. 44, 806–811. doi: 10.2135/cropsci2004.8060
Nosetto, M. D., Jobbagy, E. G., Toth, T., and Di Bella, C. M. (2007). The effects of tree establishment on water and salt dynamics in naturally salt-affected grasslands. Oecologia 152, 695–705. doi: 10.1007/s00442-007-0694-2
NRSA (National Remote Sensing Agency) and Associates (1996). Mapping Salt-Affected Soils of India, 1:250,000 Mapsheets, Legend . Hyderabad: NRSA.
Oldeman, L. R. (1998). Soil Degradation: A Threat to Food Security . Wageningen: International Soil Reference and Information Centre.
Oldeman, L. R., Hakkeling, R. T. A., and Sombroek, W. G. (1991). World Map of the Status of Human-Induced Soil Degradation. An Explanatory Note. Second Revised Edition . Wageningen: International Soil Reference and Information Center (ISRIC), 35.
Oo, A. N., Iwai, C. B., and Saenjan, P. (2013). Food security and socio-economic impacts of soil salinization in northeast Thailand. Inter. J. Environ. Rural Dev. 4, 76–81.
Parfitt, J., Barthel, M., and Macnaughton, S. (2010). Food waste within food supply chains: quantification and potential for change to 2050. Philos. Trans. R. Soc. B. 365, 3065–3081. doi: 10.1098/rstb.2010.0126
Parry, M., Rosenzweig, C., Iglesias, A., Fischer, G., and Livermore, M. (1999). Climate change and world food security: a new assessment. Global Environ. Change. 9, 51–67. doi: 10.1016/S0959-3780(99)00018-7
Patel, B. B., Patel, B. B., and Dave, R. S. (2011). Studies on infiltration of saline–alkali soils of several parts of Mehsana and Patan districts of north Gujarat. J. Appl. Technol. Environ. Sanitation. 1, 87–92.
Pimentel, D. (2006). Soil erosion: a food and environmental threat. Environ. Dev. Sustain. 8, 119–137. doi: 10.1007/s10668-005-1262-8
Prospero, J. M. (1979). Mineral and sea salt aerosol concentrations in various ocean regions. J. Geophys. Res . 84, 725–731. doi: 10.1029/JC084iC02p00725
Purushothaman, C. S., Raizada, S., Sharma, V. K., Harikrishna, V., Venugopal, G., Agrahari, R. K., et al. (2014). Production of tiger shrimp ( Penaeus monodon ) in potassium supplemented inland saline sub-surface water. J. Appl. Aquacul. 26, 84–93. doi: 10.1080/10454438.2014.882214
Qadir, M., Oster, J. D., Schubert, S., Noble, A. D., and Sahrawat, K. L. (2007). Phytoremediation of sodic and saline-sodic soils. Adv. Agron. 96, 197–247. doi: 10.1016/S0065-2113(07)96006-X
Qadir, M., Quillerou, E., Nangia, V., Murtaza, G., Singh, M., Thomas, R. J., et al. (2014). Economics of salt-induced land degradation and restoration. Nat. Res. Forum . 38, 282–295. doi: 10.1111/1477-8947.12054
Qadir, M., Qureshi, R. H., and Ahmad, N. (1997). Nutrient availability in a calcareous saline-sodic soil during vegetative bioremediation. Arid Soil Res. Rehabil . 11, 343–352. doi: 10.1080/15324989709381487
Raju, R., Thimmappa, K., Kumar, P., Kumar, S., and Tripathi, R. S. (2016). Reclamation of saline soils through subsurface drainage technology in Haryana - an economic impact analysis. J. Soil Sali. Water Quali. 8, 194–201.
Raju, R., Thimmappa, K., Pathan, A. L., and Siddyya. (2017). Saline soil reclamation through subsurface drainage in Karnataka - an economic impact analysis. J. Farm. Sci. 30, 74–78.
Ram, J., Dagar, J. C., Lai, K., and Singh, G. (2011). Biodrainage to combat waterlogging, increase farm productivity and sequester carbon in canal command areas of northwest India. Curr. Sci. 100, 1673–1680.
Rao, G. G., Khandelwal, M. K., Arora, S., and Sharma, D. K. (2014). Salinity ingress in coastal Gujarat: appraisal of control measures. J. Soil Sali. Water Qual. 4, 102–113.
Rao, G. G., Nayak, A. K., and Chinchmalatpure, A. R. (2000). Dill (Anethum graveolens): A Potential Crop for Salt-Affected Black Soils . CSSRI Tech. Monograph 1, CSSRI, RRS, Anand, India.
Rao, G. G., Nayak, A. K., and Chinchmalatpure, A. R. (2003). Salvadora persica: A Life Support of Salt-Affected Black Soils . Technical Bulletin 1/2003, CSSRI, RRS, Bharuch, India.
Rijsberman, F. R. (2006). Water scarcity: fact or fiction? Agric. Water Manage. 80, 5–22. doi: 10.1016/j.agwat.2005.07.001
Ritzema, H. P., Satyanarayana, T. V., Raman, S., and Boonstra, J. (2008). Subsurface drainage to combat waterlogging and salinity in irrigated lands in India: lessons learned in farmers' fields. Agri. Water Manage. 95, 179–189. doi: 10.1016/j.agwat.2007.09.012
Rosenzweig, C., Strzepek, K. M., Major, D. C., Iglesias, A., Yates, D. N., McCluskey, A., et al. (2004). Water resources for agriculture in a changing climate: international case studies. Glob. Environ. Change A 14, 345–360. doi: 10.1016/j.gloenvcha.2004.09.003
Shahid, S. A., Zaman, M., and Heng, L. (2018). “Soil salinity: historical perspectives and a world overview of the problem,” in Guideline for Salinity Assessment, Mitigation and Adaptation using Nuclear and Related Techniques (Cham: Springer), 43–53. doi: 10.1007/978-3-319-96190-3_2
Sharma, D. K., and Chaudhari, S. K. (2012). Agronomic research in salt-affected soils of India: an overview. Ind. J. Agron. 57, 175–185.
Sharma, D. K., Chaudhari, S. K., and Singh, A. (2014a). CSSRI Vision 2050 . Central Soil Salinity Research Institute, Karnal, India.
Sharma, D. K., Chaudhari, S. K., and Singh, A. (2014b). In salt-affected soils: agroforestry is a promising option. Ind. Farming. 63, 19–22.
Sharma, D. K., Dey, P., Gupta, S. K., and Sharma, P. C. (2011). CSSRI Vision 2030. Central Soil Salinity Research Institute, Karnal, 38 . Available online at: http://krishikosh.egranth.ac.in/handle/1/50338 (accessed December 26, 2019).
Sharma, P., and Swarup, A. (1990). Microbial Oxidation of Pyrites in Relation to Its Efficiency for Reclamation of Alkali Soils . Annual Report, Central Soil Salinity Research Institute, Karnal, India.
Shrivasata, P., and Kumar, R. (2015). Soil salinity: a serious environmental issue and plant growth promoting bacteria as one of the tools for its alleviation. Saudi J. Biologi. Sci. 22, 123–131. doi: 10.1016/j.sjbs.2014.12.001
Singh, G. (2009). Salinity-related desertification and management strategies: Indian experience. Land Degrad. Dev. 20, 367–385. doi: 10.1002/ldr.933
Singh, G., Abrol, I. P., and Cheema, S. S. (1994). Agroforestry techniques for the rehabilitation of salt lands. L. Degrad. Rehabil. 5, 223–242. doi: 10.1002/ldr.3400050306
Singh, K. N., and Sharma, P. C. (2006). Salt Tolerant Varieties Released for Saline and Alkali Soils . Central Soil Salinity Research Institute, Karnal, India.
Smedema, L. K., and Ochs, W. J. (1998). Needs and prospects for improved drainage in developing countries. Irrig. Drain. Syst. 12, 359–369. doi: 10.1023/A:1006101717310
Smith, J., Sones, K., Grace, D., MacMillan, S., Tarawali, S., and Herrero, M. (2013). Beyond milk, meat, and eggs: role of livestock in food and nutrition security. Anim. Front. 3:2. doi: 10.2527/af.2013-0002
Sparks, D. L. (2003). Environmental Soil Chemistry . Elsevier Academic Press, 352. Available online at: www.elsevier.com (accessed November 14, 2019).
Szabolcs, I. (1989). Salt-Affected Soils. Boca Raton, FL: CRC Press Inc. 274.
Tanji, K. K. (1990). “Nature and Extent of Agricultural Salinity,” in Agricultural Salinity Assessment and Management, ASCE Manuals and Reports on Engineering Practice. No 71. ed K. K. Tanji (New York, NY: ASCE), 1–17.
Tewari, V. P., Arrawatia, M. L., and Kumar, K. (1997). Problem of soil salinity and water logging in Indira Gandhi Canal area of Rajasthan State. Ann. Biol .13, 7–13.
Tiwari, R. (2020). India clocks record foodgrain production in 2018-19. The Economic Times (accessed February 12, 2020).
Tomar, O. S., and Minhas, P. S. (2004). Performance of medicinal plant species under saline irrigation. Ind. J. Agron. 49, 209–211.
Tomar, O. S., Minhas, P. S., Sharma, V. K., and Gupta, R. K. (2003a). Response of nine forage grasses to saline irrigation and its schedules in a semi-arid climate of northwest India. J. Arid Environ. 55, 533–544. doi: 10.1016/S0140-1963(02)00285-9
Tomar, O. S., Minhas, P. S., Sharma, V. K., Singh, Y. P., and Gupta, R. K. (2003b). Response of 31 tree species and soil conditions in a plantation established with saline irrigation. For. Ecol. Manage. 177, 333–346. doi: 10.1016/S0378-1127(02)00437-1
Tripathi, R. S. (2011). Socio-economic impact of reclaiming salt-affected lands in India. J. Soil Salin. Water Qual. 3, 110–126.
Tscharntke, T., Clough, Y., Wanger, T. C., Jackson, L., Motzke, I., Perfecto, I., et al. (2012). Global food security, biodiversity conservation and the future of agricultural intensification. Biolog. Conserv. 151, 53–59. doi: 10.1016/j.biocon.2012.01.068
Tyagi, N. K. (1998). “Management of salt-affected soils,” in Fifty Years of Natural Resource Management Research , eds G. B. Singh and B. R. Sharma (New Delhi: Indian Council of Agricultural Research), 363–401.
Tyagi, N. K., and Minhas, P. S. (1998). Agricultural Salinity Management in India . Central Soil Salinity Research Institute, Karnal, 526.
UNDESA (2017). World Population Prospects: The 2017 Revision. ESA.UN.org (custom data acquired via website). United Nations Department of Economic and Social Affairs, Population Division (accessed September 10, 2017).
Vaidyanathan, A. (1986). India's Agricultural Development in a Regional Perspective. R.C. Dutt Lectures on Political Economy, Centre for Studies in Social Sciences, Calcutta.
Vance, W. H., Tisdeel, J. M., and McKenzie, B. M. (1998). Residual effects of surface application of organic matter and calcium salts on the sub-soil of a red-brown earth. Aust. J. Exptl. Agri. 38, 595–600. doi: 10.1071/EA97102
Verma, C. L., Sharma, D. K., Singh, Y. P., Singh, R., Mishra, V. K., Nayak, A. K., et al. (2012). “Management of seepage water in canal command through integrated farming system to harness the productivity of waterlogged sodic soils,” in Abstracts 2nd National Seminar on Management of Salt-affected Soils and Water-Challenges of 21st Century, Indian Society of Soil Salinity and Water Quality, March 16-17, 2012 (Lucknow), 136.
Wicke, B., Smeets, E., Domburg, V., Vashev, B., Gaiser, T., Turkenburg, W., et al. (2011). The global technical and economic potential of bioenergy from salt-affected soils. Energy Environ. Sci. 4, 2669–2681. doi: 10.1039/C1EE01029H
Wong, N. L., Dalal, R. C., and Greene, R. S. B. (2009). Carbon dynamics of sodic and saline soils following gypsum and organic material additions: a laboratory incubation. Appl. Soil Ecol. 40, 29–40. doi: 10.1016/j.apsoil.2008.08.006
Ziaul Haider, M., and Zaber Hossain, M. (2013). Impact of salinity on livelihood strategies of farmers. J. Soil Sci. Plant Nut. 13, 417–431. doi: 10.4067/S0718-95162013005000033
Keywords: degraded lands, salinization, saline soils, sodic soils, reclamation technologies, food security
Citation: Kumar P and Sharma PK (2020) Soil Salinity and Food Security in India. Front. Sustain. Food Syst. 4:533781. doi: 10.3389/fsufs.2020.533781
Received: 10 February 2020; Accepted: 31 August 2020; Published: 06 October 2020.
Reviewed by:
Copyright © 2020 Kumar and Sharma. This is an open-access article distributed under the terms of the Creative Commons Attribution License (CC BY) . The use, distribution or reproduction in other forums is permitted, provided the original author(s) and the copyright owner(s) are credited and that the original publication in this journal is cited, in accordance with accepted academic practice. No use, distribution or reproduction is permitted which does not comply with these terms.
*Correspondence: Pardeep Kumar, drpardeep1968@gmail.com
† Present address: Pradeep K. Sharma VPO Aima, Palampur, India
This article is part of the Research Topic
Sustaining Soil Carbon to Enhance Soil Health, Food, Nutritional Security, and Ecosystem Services
Literature Review on Food Security of India
Food security policy in india: challenges and performance[1], food security in india: trends, patterns and determinants [2], food security and nutrition: vision 2020 [3], food and nutrition in india: facts and interpretation [4], food security in india: performance and concerns, food security in india: issues and challenges[5], food security in india: concepts, realities & innovations [6], economic and environmental impact of national food security act of india [7], indian food security from problem to solution through household food security[8], food security in india: evolution, efforts and problems [9].
- Food security of a nation is ensured if all of its citizens have enough nutritious food available, all persons have the capacity to buy food of acceptable quality and there is no barrier on access to food.
- The right to food is a well established principle of international human rights law. It has evolved to include an obligation for state parties to respect, protect, and fulfil their citizens' right to food security.
- As a state party to the Universal Declaration of Human Rights and the International Covenant on Economic, Social and Cultural Rights, India has the obligation to ensure the right to be free from hunger and the right to adequate food.
- India needs to adopt a policy that brings together diverse issues such as inequality, food diversity, indigenous rights and environmental justice to ensure sustainable food security.
- https://www.researchgate.net/publication/349767066_Food_Security_Policy_in_India_Challenges_and_Performance
- https://www.researchgate.net/publication/288046176_Food_security_in_India_Trends_patterns_and_determinants
- https://www.semanticscholar.org/paper/Food-Security-and-Nutrition-%3A-Vision-2020-Radhakrishna-Reddy/3a2f8512693980d418d8bf1600b1e69a01e17006
- https://www.researchgate.net/publication/261890931_Food_and_Nutrition_in_India_Facts_and_Interpretations
- https://www.researchgate.net/publication/331523537_FOOD_SECURITY_IN_INDIA_ISSUES_AND_CHALLENGES
- https://www.researchgate.net/publication/228096218_Food_Security_in_India_Concept_Realities_innovations
- https://www.researchgate.net/publication/295861963_Economic_and_Environmental_Impact_of_National_Food_Security_Act_of_India
- http://fetrian.fisip.unand.ac.id/index.php/fetrian/article/view/207
- https://www.researchgate.net/publication/331108394_Food_Security_in_India_Evolution_Efforts_and_Problems
Law Article in India
Please drop your comments, you may like.
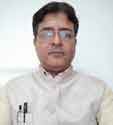
The Intersection of Tradema...
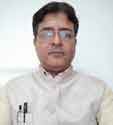
Trademark Infringement and ...
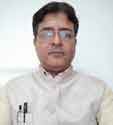
Cancellation of Registered ...
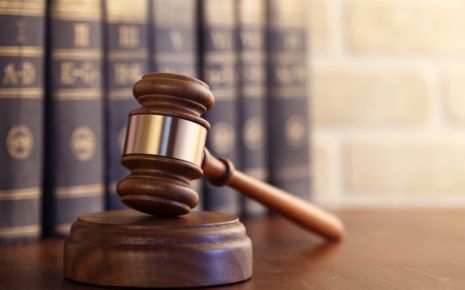
An Analysis Of The Independ...
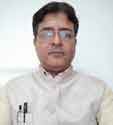
Defense of Section 28 of Tr...
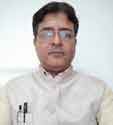
Nature and Scope of punishm...
Legal question & answers, lawyers in india - search by city.
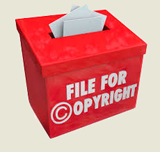
Law Articles
How to file for mutual divorce in delhi.
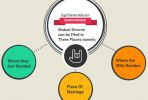
How To File For Mutual Divorce In Delhi Mutual Consent Divorce is the Simplest Way to Obtain a D...
Increased Age For Girls Marriage
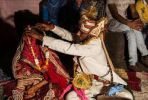
It is hoped that the Prohibition of Child Marriage (Amendment) Bill, 2021, which intends to inc...
Facade of Social Media
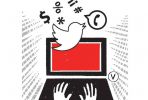
One may very easily get absorbed in the lives of others as one scrolls through a Facebook news ...
Section 482 CrPc - Quashing Of FIR: Guid...
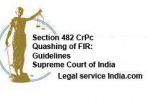
The Inherent power under Section 482 in The Code Of Criminal Procedure, 1973 (37th Chapter of t...
The Uniform Civil Code (UCC) in India: A...

The Uniform Civil Code (UCC) is a concept that proposes the unification of personal laws across...
Role Of Artificial Intelligence In Legal...
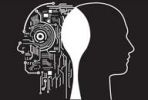
Artificial intelligence (AI) is revolutionizing various sectors of the economy, and the legal i...
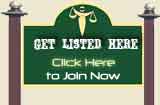
File caveat In Supreme Court Instantly
Impact of climate change on food security in India: an evidence from autoregressive distributed lag model
- Published: 27 November 2023
Cite this article
- Biswabhusan Bhuyan ORCID: orcid.org/0000-0002-3994-3538 1 ,
- Ranjan Kumar Mohanty 2 &
- Subhamitra Patra 3
446 Accesses
3 Citations
Explore all metrics
Food security is a critical global issue, particularly in India, and it is further exacerbated by the challenges posed by climate change. This study aims to examine the influence of climate change on food security in India, utilizing annual time series data spanning from 1994 to 2019. Using the Autoregressive Distributed Lag (ARDL) method, the study investigates the relationship between climate variables, area under cultivation, population growth, agricultural subsidies, and food production. The findings indicate that expanding the cultivation area for food crops significantly enhances food security, with a 1% increase leading to a 2.4% increase in the short run and a 3.2% increase in the long run. Conversely, population growth has a detrimental effect on food security, with a 1% increase resulting in a decline of 3.8% in the short run and 7.8% in the long run. Climate variables also play a crucial role, as rising temperatures adversely impact food security, leading to a decrease of 1.2% in the short run and 1.7% in the long run for every 1% temperature increase. Rainfall, on the other hand, does not significantly affect food security in the long run, but a decrease in rainfall in the preceding period negatively impacts food security in the short run. Furthermore, agricultural subsidies, particularly fertilizer subsidies, impact food security positively in the short run but have adverse effects in the long run. The study highlights the importance of sustainable land management, temperature control measures, water reservation, and effective agricultural subsidies to address food security challenges. These findings provide valuable insights for policymakers in designing effective strategies to ensure food security in India.
This is a preview of subscription content, log in via an institution to check access.
Access this article
Price excludes VAT (USA) Tax calculation will be finalised during checkout.
Instant access to the full article PDF.
Rent this article via DeepDyve
Institutional subscriptions
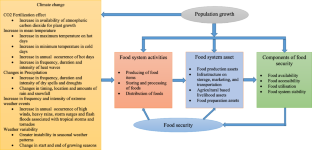
Source: https://vikaspedia.in/agriculture/crop-production/weather-information/agro-climatic-zones-in-india accessed on 13 th /June/2023 at 12.03 a.m
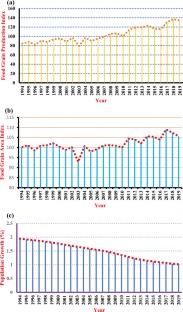
Source: Authors’ computations. CUSUM: cumulative sum. CUSUM SQ: cumulative sum of squares
Similar content being viewed by others
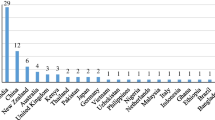
Climate-smart agriculture: adoption, impacts, and implications for sustainable development
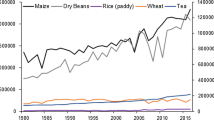
Climate change and variability in Kenya: a review of impacts on agriculture and food security
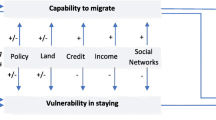
The impact of climate change on migration: a synthesis of recent empirical insights
Data availability.
The datasets used in the analysis are available in the Indiastat.com, RBI, EPWRPF, and World Development Indicators repository.
The major crops production such as wheat, rice, maize, and soybean are susceptible to changes in mean air temperature in Southeast Asia (Liu et al., 2020 ). Similarly, an Indian study predicted a decline of 2.5% in wheat production compared to the base year 2013–14 due to climate change (Amita and Surender 2020 ). Such reductions in agricultural production are likely to exacerbate food insecurity levels in India.
According to this report, drought-like situations occurred in India during the years 2002–03, 2009–10, 2014–15, and 2015–16 (GOI, 2016 ). These droughts have led to a decline in food consumption, forcing rural populations in Odisha, India, to adopt coping strategies (Sam et al., 2020 ). Unfortunately, such coping strategies often contribute to an increase in food insecurity levels.
In fact, approximately 74% of districts in India are affected by this climate variability. Source: https://theprint.in/environment/74-of-indias-districts-prone-to-extreme-climate-like-droughts-floods-cyclones-says-study/756813/ Accessed on 8 th /August/2022 at 11.44 p.m.
Furthermore, the report highlights that during the period of 1986–2015, the temperature of the coldest night increased by approximately 0.4%, while the temperature of the warmest day rose by around 0.63% (Krishnan et al., 2020 ).
The recent Food and Agriculture Organization (FAO) report shows that about 70 percent of the rural population relied on agricultural activities to provide life and livelihood. Besides, the report also revealed that 82 percent of the farmers are small and marginal. These small and marginal farmers often depend on the monsoon because rainfall is the primary water source for irrigation in agricultural activity. Due to natural and anthropogenic activities, climate change likely affected the temperature and rainfall. As a result, there is a chance of adverse impact on the agricultural sector, and hence, food security.
This method can be used for the combination of stationary and non-stationary time series, which is most advantage comparative to the other time series modeling. It provides information on the short-run and long-run impact of the predictors of importance. It is efficient in small samples. Additionally, it also reports the error correction mechanism which shows the speed of adjustment to move from disequilibrium to the equilibrium in the short-run. Due to above importance, the present study used ARDL method to examine our objective.
The information is obtained from https://datahelpdesk.worldbank.org/knowledgebase/articles/906519-world-bank-country-and-lending-groups on 08 th /Sept/2022 at 5.23 p.m.
The information is obtained from following website https://www.india.gov.in/india-glance/profile on 08 th /Sept/2022 at 4.35 p.m.
The information is obtained from http://cwc.gov.in/sites/default/files/NRLD_04012017.pdf on 30th/Sept/2022 at 6.15 p.m.
The order of integration in time series, denoted as "I(d),” refers to the number of times required to be differentiable to become stationary. Stationarity of time series refers to the certain condition fulfilment such as the mean, variance, and covariance should be time invariant. If the time series is stationary at level, it is represented as I(0) whereas if the time series is stationary at first difference, it is denoted as I(1).
Cointegration between time series refers to the fact that the time series moves together in long-run without the divergency from each other.
Abbas, S. (2020). Climate change and cotton production: An empirical investigation of Pakistan. Environmental Science and Pollution Research, 27 (23), 29580–29588. https://doi.org/10.1007/s11356-020-09222-0
Article Google Scholar
Abdelzaher, M. A., Farahat, E. M., Abdel-Ghafar, H. M., Balboul, B. A. A., & Awad, M. M. (2023). Environmental policy to develop a conceptual design for the water–energy–food nexus: A case study in Wadi-Dara on the Red Sea Coast. Egypt. Water, 15 (4), 780.
Article CAS Google Scholar
AbdelzaherShehata, M. A. N. (2022). Hydration and synergistic features of nanosilica-blended high alkaline white cement pastes composites. Applied Nanoscience, 12 (5), 1731–1746.
Ahmad, J., Alam, D., & Haseen, M. S. (2011). Impact of climate change on agriculture and food security in India. International Journal of Agriculture, Environment and Biotechnology, 4 (2), 129–137.
Google Scholar
Ali, S., Liu, Y., Ishaq, M., Shah, T., Ilyas, A., & Din, I. U. (2017). Climate change and its impact on the yield of major food crops: Evidence from Pakistan. Foods, 6 (6), 39.
Amita, R., & Surender, M. (2020). Climate variability and wheat crop yield: Forecasting Indian scenario till 2100 AD. Disaster Advances, 13 (11), 31–41.
Arora, N. K. (2019). Impact of climate change on agriculture production and its sustainable solutions. Environmental Sustainability, 2 (2), 95–96. https://doi.org/10.1007/s42398-019-00078-w
Auffhammer, M., Ramanathan, V., & Vincent, J. R. (2012). Climate change, the monsoon, and rice yield in India. Climatic Change, 111 (2), 411–424. https://doi.org/10.1007/s10584-011-0208-4
Baig, I. A., Ahmed, F., Salam, M. A., & Khan, S. M. (2020). An assessment of climate change and crop productivity in India: A multivariate cointegration framework. TEST Engineering & Management, 83 , 3438–3452.
Bandara, J. S., & Cai, Y. (2014). The impact of climate change on food crop productivity, food prices and food security in South Asia. Economic Analysis and Policy, 44 , 451–465. https://doi.org/10.1016/j.eap.2014.09.005
Barrios, S., Ouattara, B., & Strobl, E. (2008). The impact of climatic change on agricultural production: Is it different for Africa? Food Policy, 33 (4), 287–298.
Benjeddou, O., Ravindran, G., & Abdelzaher, M. A. (2023). Thermal and acoustic features of lightweight concrete based on marble wastes and expanded perlite aggregate. Buildings, 13 (4), 992.
Ben Zaied, Y., & Zouabi, O (2015) Climate change impacts on agriculture: A panel cointegration approach and application to Tunisia.
Bhuyan, B., Patra, S., & Bhuian, R. K. (2022). Measurement and determinants of total factor productivity: evidence from Indian banking industry. International Journal of Productivity and Performance Management, 71 (7), 2970–2990.
Bhuyan, B., Sahoo, B. K., & Suar, D. (2020a). Food insecurity dynamics in India: A synthetic panel approach. Social Sciences & Humanities Open, 2 (1), 100029. https://doi.org/10.1016/j.ssaho.2020.100029
Bhuyan, B., Sahoo, B. K., & Suar, D. (2020b). Nutritional status, poverty, and relative deprivation among socio-economic and gender groups in India: Is the growth inclusive? World Development Perspectives, 18 , 1–15. https://doi.org/10.1016/j.wdp.2020.100180
Bhuyan, B., Sahoo, B. K., & Suar, D. (2020c). Quantile Regression Analysis of Predictors of Calorie Demand in India: An Implication for Sustainable Development Goals. Journal of Quantitative Economics., 18 , 825–859. https://doi.org/10.1007/s40953-020-00200-4
Bhuyan, B., Patra, S., & Bhuian, R. K. (2020d). Market Adaptability and Evolving Predictability of Stock Returns: An Evidence from India. Asia-Pac Financ Markets, 27 , 605–619. https://doi.org/10.1007/s10690-020-09308-2
Birthal, P. S., Khan, T., Negi, D. S., & Agarwal, S. (2014). Impact of climate change on yields of major food crops in India: Implications for food security. Agricultural Economics Research Review, 27 (2), 145–155.
Chandio, A. A., Jiang, Y., Rehman, A., & Rauf, A. (2020a). Short and long-run impacts of climate change on agriculture: An empirical evidence from China. International Journal of Climate Change Strategies and Management, 12 (2), 201–221.
Chandio, A. A., Ozturk, I., Akram, W., Ahmad, F., & Mirani, A. A. (2020b). Empirical analysis of climate change factors affecting cereal yield: Evidence from Turkey. Environmental Science and Pollution Research, 27 (11), 11944–11957.
Chandio, A. A., Jiang, Y., Amin, A., Ahmad, M., Akram, W., & Ahmad, F. (2023). Climate change and food security of South Asia: Fresh evidence from a policy perspective using novel empirical analysis. Journal of Environmental Planning and Management, 66 (1), 169–190.
Choudhary, B. B., & Sirohi, S. (2022). Understanding vulnerability of agricultural production system to climatic stressors in North Indian Plains: a meso-analysis. Environment, Development and Sustainability, 24 , 13522–13541.
Das, M. K., & Patra, S. (2016a). Productivity and Efficiency of Public Sector Banks in India After the Global Financial Crisis. IUP Journal of Bank Management , 15(2).
Das, M. K., & Patra, S. (2016b). Productivity and efficiency of private sector banks after global financial crisis: evidence from India. Asian Journal of Research in Banking and Finance, 6 (5), 1–14.
Datta, P., & Behera, B. (2022). Climate change and Indian agriculture: A systematic review of farmers’ perception, adaptation, and transformation. Environmental Challenges, 8 , 100543.
Demeke, A. B., Keil, A., & Zeller, M. (2011). Using panel data to estimate the effect of rainfall shocks on smallholders food security and vulnerability in rural Ethiopia. Climatic Change, 108 (1), 185–206. https://doi.org/10.1007/s10584-010-9994-3
Dethier, J.-J., & Effenberger, A. (2012). Agriculture and development: A brief review of the literature. Economic Systems, 36 (2), 175–205.
Dumrul, Y., & Kilicaslan, Z. (2017). Economic impacts of climate change on agriculture: Empirical evidence from ARDL approach for Turkey. Journal of Business Economics and Finance, 6 (4), 336–347.
Elkhouly, H. I., Abdelzaher, M. A., & El-Kattan, I. M. (2021). Experimental and modeling investigation of physicomechanical properties and firing resistivity of cement pastes incorporation of micro-date seed waste. Iranian Journal of Science and Technology, Transactions of Civil Engineering, 46 , 2809–2821.
FAO WFP and IFAD. (2012). The State of Food Insecurity in the World: Economic growth is necessary but not sufficient to accelerate reduction of hunger and malnutrition . Food and Agriculture Organization of the United Nations . http://www.fao.org/3/a-i3027e.pdf
FAO. (1996). World Food Summit. http://www.fao.org/3/w3613e/w3613e00.htm . Accessed 8 December 2019
FAO. (2008). Climate change and food security: a framework document . FAO, Rome
FAO. (2015). The State of Food Insecurity in the World 2015 . http://www.fao.org/3/a-i4646e.pdf
GOI. (2016). Manual for Drought Management . https://agricoop.nic.in/sites/default/files/Manual Drought 2016.pdf. Accessed 8 Aug 2023
Grivins, M., Thorsøe, M. H., & Maye, D. (2021). Financial subjectivities in the agricultural sector: A comparative analysis of relations between farmers and banks in Latvia, Denmark and the UK. Journal of Rural Studies, 86 , 117–126.
Guntukula, R. (2020). Assessing the impact of climate change on Indian agriculture: Evidence from major crop yields. Journal of Public Affairs, 20 (1), 1–7. https://doi.org/10.1002/pa.2040
IPCC. (2014). Climate Change 2014: Synthesis Report. Contribution of Working Groups I, II and III to the Fifth Assessment Report of the Intergovernmental Panel on Climate Change [Core Writing Team, R.K. Pachauri and L.A. Meyer (eds.)]. IPCC, Geneva, Switzerland.
Janjua, P. Z., Samad, G., & Khan, N. (2014). Climate change and wheat production in Pakistan: An autoregressive distributed lag approach. NJAS - Wageningen Journal of Life Sciences, 68 , 13–19. https://doi.org/10.1016/j.njas.2013.11.002
Khatri, N., & Tyagi, S. (2015). Influences of natural and anthropogenic factors on surface and groundwater quality in rural and urban areas. Frontiers in Life Science, 8 (1), 23–39. https://doi.org/10.1080/21553769.2014.933716
Kotir, J. H. (2011). Climate change and variability in Sub-Saharan Africa: A review of current and future trends and impacts on agriculture and food security. Environment, Development and Sustainability, 13 (3), 587–605. https://doi.org/10.1007/s10668-010-9278-0
Krishnan, R., Sanjay, J., Gnanaseelan, C., Mujumdar, M., Kulkarni, A., & Chakraborty, S. (2020). Assessment of climate change over the Indian region: a report of the ministry of earth sciences (MOES), government of India . Springer.
Book Google Scholar
Kumar, S. (2021). Sources and determinants of agricultural growth in Uttar Pradesh: Agro-climatic zone level analysis. Indian Journal of Economics and Development, 17 (3), 534–543.
Kumar, S., & Upadhyay, S. K. (2019). Impact of climate change on agricultural productivity and food security in India: A State level analysis. Indian Journal of Agricultural Research, 53 (2), 133–142.
Kumar, A., Ahmad, M. M., & Sharma, P. (2017). Influence of climatic and non-climatic factors on sustainable food security in India: A statistical investigation. International Journal of Sustainable Agricultural Management and Informatics, 3 (1), 1–30.
Kumar, P., Sahu, N. C., Kumar, S., & Ansari, M. A. (2021). Impact of climate change on cereal production: Evidence from lower-middle-income countries. Environmental Science and Pollution Research, 28 (37), 51597–51611. https://doi.org/10.1007/s11356-021-14373-9
Kumar, & Sharma, P. (2013). Impact of climate variation on agricultural productivity and food security in rural India (No. 2013–43).
Liu, D., Mishra, A. K., & Ray, D. K. (2020). Sensitivity of global major crop yields to climate variables: A non-parametric elasticity analysis. Science of the Total Environment, 748 , 141431. https://doi.org/10.1016/j.scitotenv.2020.141431
Lobell, D. B., Schlenker, W., & Costa-Roberts, J. (2011). Climate trends and global crop production since 1980. Science, 333 (6042), 616–620.
Loum, A., & Fogarassy, C. (2015). The effects of climate change on cereals yield of production and food security in Gambia. Applied Studies in Agribusiness and Commerce, 9 (4), 83–92.
Mohanty, R. K., Panda, S., & Bhuyan, B. (2020). Does defence spending and its composition affect economic growth in India? Margin, 14 (1), 62–85. https://doi.org/10.1177/0973801019886486
Mozumdar, L. (2012). Agricultural productivity and food security in the developing world. Bangladesh Journal of Agricultural Economics, 35 (454-2016–36530), 53–69.
Muttreja, P., & Singh, S. (2018). Family planning in India: The way forward. The Indian Journal of Medical Research, 148 (Suppl 1), S1.
Onour, I. (2019). Effect of carbon dioxide concentration on cereal yield in Sudan. Management and Economics Research Journal, 5 (S3), 7.
Owaid, K. A., Hamdoon, A. A., Matti, R. R., Saleh, M. Y., & Abdelzaher, M. A. (2022). Waste polymer and lubricating oil used as asphalt rheological modifiers. Materials, 15 (11), 3744.
Patra, S., & Hiremath, G. S. (2022). An Entropy Approach to Measure the Dynamic Stock Market Efficiency. Journal of Quantitative Economics, 20 (2), 337–377.
Pesaran, M. H., Shin, Y., & Smith, R. J. (2001). Bounds testing approaches to the analysis of level relationships. Journal of Applied Econometrics, 16 (3), 289–326. https://doi.org/10.1002/jae.616
Praveen, B., & Sharma, P. (2020). Climate change and its impacts on Indian agriculture: An econometric analysis. Journal of Public Affairs, 20 (1), e1972.
Rao, C. A. R., Raju, B. M. K., Rao, A. V. M. S., Rao, K. V., Rao, V. U. M., Ramachandran, K., et al. (2016). A district level assessment of vulnerability of Indian agriculture to climate change. Current Science, 110 (10), 1939–1946.
Ray, L. K., Goel, N. K., & Arora, M. (2019). Trend analysis and change point detection of temperature over parts of India. Theoretical and Applied Climatology, 138 (1), 153–167. https://doi.org/10.1007/s00704-019-02819-7
Rohini, P., Rajeevan, M., & Srivastava, A. K. (2016). On the variability and increasing trends of heat waves over India. Scientific Reports, 6 (1), 26153. https://doi.org/10.1038/srep26153
Saha, A., Ghosh, S., Sahana, A. S., & Rao, E. P. (2014). Failure of CMIP5 climate models in simulating post-1950 decreasing trend of Indian monsoon. Geophysical Research Letters, 41 (20), 7323–7330.
Sam, A. S., Padmaja, S. S., Kächele, H., Kumar, R., & Müller, K. (2020). Climate change, drought and rural communities: Understanding people’s perceptions and adaptations in rural eastern India. International Journal of Disaster Risk Reduction, 44 , 101436. https://doi.org/10.1016/j.ijdrr.2019.101436
Sarker, M. A. R., Alam, K., & Gow, J. (2014). Assessing the effects of climate change on rice yields: An econometric investigation using Bangladeshi panel data. Economic Analysis and Policy, 44 (4), 405–416. https://doi.org/10.1016/j.eap.2014.11.004
Sharma, S., & Mujumdar, P. (2017). Increasing frequency and spatial extent of concurrent meteorological droughts and heatwaves in India. Scientific Reports, 7 (1), 15582. https://doi.org/10.1038/s41598-017-15896-3
Singh, G. (2016). Climate change and food security in India: Challenges and opportunities. Irrigation and Drainage, 65 , 5–10.
Singh, N. P., Singh, S., Anand, B., & Ranjith, P. C. (2019). Assessing the impact of climate change on crop yields in Gangetic Plains Region India. Journal of Agrometeorology, 21 (4), 452–461.
SinghBhuyan, K. B. (2016). Estimating Taylor type rule for India’s monetary policy using ARDL approach to co-integration. Indian Journal of Economics and Development, 12 (3), 515–520.
Timmer, C. P. (1995). Getting agriculture moving: do markets provide the right signals? Food policy, 20 (5), 455–472.
Tokunaga, S., Okiyama, M., & Ikegawa, M. (2015). Dynamic panel data analysis of the impacts of climate change on agricultural production in Japan. Japan Agricultural Research Quarterly, 49 (2), 149–157. https://doi.org/10.6090/jarq.49.149
Warsame, A. A., Sheik-Ali, I. A., Ali, A. O., & Sarkodie, S. A. (2021). Climate change and crop production nexus in Somalia: An empirical evidence from ARDL technique. Environmental Science and Pollution Research, 28 (16), 19838–19850.
Zhao, C., Liu, B., Piao, S., Wang, X., Lobell, D. B., Huang, Y., et al. (2017). Temperature increase reduces global yields of major crops in four independent estimates. Proceedings of the National Academy of Sciences, 114 (35), 9326–9331.
Download references
Author information
Authors and affiliations.
Department of Economics, Maharaja Purna Chandra (Autonomous) College, Baripada- 757003, Odisha, India
Biswabhusan Bhuyan
Xavier Institute of Management, XIM University, Bhubaneswar-751013, Odisha, India
Ranjan Kumar Mohanty
Goa Institute of Management, Sanquelim- 403505, Goa, India
Subhamitra Patra
You can also search for this author in PubMed Google Scholar
Contributions
BB was involved in conception and design , data collection and analysis, first draft of the manuscript and revision. RKM contributed to data collection and analysis and provided comments to improve the manuscript. SP was involved in conception and design, drafting and provided comments to improve the manuscript. All authors read and approved the final manuscript.
Corresponding author
Correspondence to Biswabhusan Bhuyan .
Ethics declarations
Conflict of interest.
We have no conflicts of interest to disclose.
Additional information
Publisher's note.
Springer Nature remains neutral with regard to jurisdictional claims in published maps and institutional affiliations.
Rights and permissions
Springer Nature or its licensor (e.g. a society or other partner) holds exclusive rights to this article under a publishing agreement with the author(s) or other rightsholder(s); author self-archiving of the accepted manuscript version of this article is solely governed by the terms of such publishing agreement and applicable law.
Reprints and permissions
About this article
Bhuyan, B., Mohanty, R.K. & Patra, S. Impact of climate change on food security in India: an evidence from autoregressive distributed lag model. Environ Dev Sustain (2023). https://doi.org/10.1007/s10668-023-04139-3
Download citation
Received : 12 July 2022
Accepted : 01 November 2023
Published : 27 November 2023
DOI : https://doi.org/10.1007/s10668-023-04139-3
Share this article
Anyone you share the following link with will be able to read this content:
Sorry, a shareable link is not currently available for this article.
Provided by the Springer Nature SharedIt content-sharing initiative
- Food security
- Temperature
- Fertilizer subsidies
JEL Classification
- Find a journal
- Publish with us
- Track your research

IMAGES
VIDEO
COMMENTS
Food insecurity measured via the HFIAS ranged from 77.2% in a population of 250 women who resided in an urban area in South Delhi [ 25] to 8.7% in Indian children [ 26 ]. The second most common measurement tool identified in this search is the US Household Food Security Survey Module (HFSSM).
This review draws attention to the lack of published literature in areas of PDS and food security in India. The findings of the review emphasise the role of PDS in tackling hunger and malnutrition while highlighting its limited role in improving food security and childhood mortality due to operational inefficiencies.
This systematic review investigated the peer-reviewed literature examining food insecurity in India to identify both the breadth of research being conducted as well as the instruments used and the populations under study. Recent findings: Nine databases were searched in March 2020. After excluding articles that did not meet the inclusion ...
According to its annual report, the FAO estimates there are 189.2 million undernourished people in India (The State of Food Security and Nutrition in the World 2020 ). Approximately 14% of India's population (189.2 million) is undernourished, and Between 15 and 49 years old, 51.4% of women are anemic.
Our analyses for 2050 highlight severe food security challenges for India, even in scenarios which assume attainment of 90% AY for all crops. ... Loganes C, Ballali S, Gafare CE, et al. Types of Food and Nutrient Intake in India: A Literature Review. Indian J Pediatr 2014;81:17-22. pmid:24928105 . View Article PubMed/NCBI Google Scholar 36 ...
A nine-item experience-based food security scale is constructed by adapting the United States Household Food Security Survey Module in the context of slum households of Kolkata, according to which ...
The National Food Security Act (NFSA) 2013, passed recently by the Indian Parliament, aims to ensure food security in India, chiefly by providing cereals at subsidized prices through the Targeted Public Distribution System (TPDS) for about two-thirds of households.
Literature review and hypothesis development. Previous studies of food security in India fall into two broad categories: the first, which incorporates the majority of studies, focuses on food security trends; the second, smaller body of research, focuses on examining predictors of food (in)security. 2.1. Food security trends and their determinants
This systematic review investigated the peer-reviewed literature examining food insecurity in India to identify both the breadth of research being conducted as well as the instruments used and the populations under study. ... Bantilan MCS, Kumar P, Kumar S, Jee S. Food security in India: trends, patterns and determinants. ... Harris J, Ballard ...
2. The Challenge of Food Security in India. There is a perception that China has, by and large, solved its 'food problem', whereas India has not (Timmer 2014). This rings true in a very specific sense. The crux of India's food problem today pertains not so much on increasing food availability or production but with the distribution of food.
Purpose of Review India is home to an estimated 200 million malnourished people, suggesting widespread food insecurity. However, variations in the methods used for determining food insecurity status mean there is uncertainty in the data and severity of food insecurity across the country. This systematic review investigated the peer-reviewed literature examining food insecurity in India to ...
Recent evidence on food security points to an increase in the number of undernourished people over the past few years, despite renewed global policy emphasis and consensus on the need to end hunger and poverty worldwide. This is particularly true in the South Asian context: although there have been substantial gains in food production in India over the past few decades, the region is home to ...
an overview of the severity of food insecurity in India as presented by these studies. Method A systematic search was undertaken to identify all food security research conducted at the household level in India. The search was conducted in March 2020. Key search terms were based on the FAO [] definition of food security: 8
Moving towards sustainable food systems is a complex problem, which requires high level co-ordination, coherence, and integration of national food policy. The aim of this study is to explore where environmental sustainability is integrated into national food policy in India. A scoping review of food policies was conducted, and findings mapped ...
Food security exists when all people, at all time, have physical and economic access to sufficient and nutritious food. The study aims at developing a conceptual model on determinants influencing the household food security in India. Theoretical model approach and systematic investigation of the available literature was undertaken, to identify various determinants affecting the household food ...
This study performs a systematic mapping of food security related research in Pakistan, Bangladesh, and India. Systematic mapping has been used in medical research (Kitchenham et al., 2010) but is not widely applied in the food security literature.Systematic mapping, like systematic review, uses a transparent protocol to document each step of the research process.
In this section, we present a literature review for understanding food policy induced differences in food security outcomes in India and China. We first review the effects of domestic subsidies on agricultural production and farm income, followed by a discussion of the national and international market effects of the two countries' food policies.
India would require around 311 million tons of food grains (cereals and pulses) during 2030 to feed around 1.43 billion people, and the requirement expectedly would further increase to 350 million tons by 2050 when India's population would be around 1.8 billion. To achieve food security in the country, the attempts need to focus on both area expansion under agriculture as well as rise in crop ...
The basic need includes adequate access to food, health facilities, educational opportunities, housing, community participation and social integration (Frankenberger & McCaston, Citation 1998).India has emerged as one of the fast growing economies (Dreze & Sen, Citation 2014).Despite this growth, insufficiencies in terms of income, education, health care are also visible (Kasthuri, Citation ...
PDF | On Jan 21, 2013, Supravat Bagli published A Study of Food Security in India | Find, read and cite all the research you need on ResearchGate. ... Review of Selected Literature.
It ranked India 66th among 105 nations and cited affordability rather than availability as a key food security threat for Indians. India scored highest in food availability (51.3) but lowest (38.4) in terms of food access. It also points to its poor ability to move food efficiently because of infrastructure problems.
Food security is a critical global issue, particularly in India, and it is further exacerbated by the challenges posed by climate change. This study aims to examine the influence of climate change on food security in India, utilizing annual time series data spanning from 1994 to 2019. Using the Autoregressive Distributed Lag (ARDL) method, the study investigates the relationship between ...
In 2016, Pradhan Mantri Fasal Bima Yojana (PMFBY) was introduced by the gov-ernment with new features, and an initial amount of Rs. 5500 crore was allocated in the 2016-2017 union budget (Rai 2019). This scheme provides a comprehensive risk solution at the lowest uniform premium rate across India.
Organic agriculture has gained significant attention as a potential solution to India's food security challenges. This study aims to provide a comprehensive review of the existing literature on ...