About me and why I created this physics website.

Velocity Problems
- Search Website
Real World Applications — for high school level and above
- Amusement Parks
- Battle & Weapons
- Engineering
- Miscellaneous
Education & Theory — for high school level and above
- Useful Formulas
- Physics Questions
- Example Mechanics Problems
- Learn Physics Compendium
Kids Section
- Physics For Kids
- Science Experiments
- Science Fair Ideas
- Science Quiz
- Science Toys
- Teacher Resources
- Commercial Disclosure
- Privacy Policy
© Copyright 2009-2024 real-world-physics-problems.com

2.2 Speed and Velocity
Section learning objectives.
By the end of this section, you will be able to do the following:
- Calculate the average speed of an object
- Relate displacement and average velocity
Teacher Support
The learning objectives in this section will help your students master the following standards:
- (B) describe and analyze motion in one dimension using equations with the concepts of distance, displacement, speed, average velocity, instantaneous velocity, and acceleration.
In addition, the High School Physics Laboratory Manual addresses content in this section in the lab titled: Position and Speed of an Object, as well as the following standards:
Section Key Terms
In this section, students will apply what they have learned about distance and displacement to the concepts of speed and velocity.
[BL] [OL] Before students read the section, ask them to give examples of ways they have heard the word speed used. Then ask them if they have heard the word velocity used. Explain that these words are often used interchangeably in everyday life, but their scientific definitions are different. Tell students that they will learn about these differences as they read the section.
[AL] Explain to students that velocity, like displacement, is a vector quantity. Ask them to speculate about ways that speed is different from velocity. After they share their ideas, follow up with questions that deepen their thought process, such as: Why do you think that? What is an example? How might apply these terms to motion that you see every day?
There is more to motion than distance and displacement. Questions such as, “How long does a foot race take?” and “What was the runner’s speed?” cannot be answered without an understanding of other concepts. In this section we will look at time , speed, and velocity to expand our understanding of motion.
A description of how fast or slow an object moves is its speed. Speed is the rate at which an object changes its location. Like distance, speed is a scalar because it has a magnitude but not a direction. Because speed is a rate, it depends on the time interval of motion. You can calculate the elapsed time or the change in time, Δ t Δ t , of motion as the difference between the ending time and the beginning time
The SI unit of time is the second (s), and the SI unit of speed is meters per second (m/s), but sometimes kilometers per hour (km/h), miles per hour (mph) or other units of speed are used.
When you describe an object's speed, you often describe the average over a time period. Average speed , v avg , is the distance traveled divided by the time during which the motion occurs.
You can, of course, rearrange the equation to solve for either distance or time
Suppose, for example, a car travels 150 kilometers in 3.2 hours. Its average speed for the trip is
A car's speed would likely increase and decrease many times over a 3.2 hour trip. Its speed at a specific instant in time, however, is its instantaneous speed . A car's speedometer describes its instantaneous speed.
[OL] [AL] Caution students that average speed is not always the average of an object's initial and final speeds. For example, suppose a car travels a distance of 100 km. The first 50 km it travels 30 km/h and the second 50 km it travels at 60 km/h. Its average speed would be distance /(time interval) = (100 km)/[(50 km)/(30 km/h) + (50 km)/(60 km/h)] = 40 km/h. If the car had spent equal times at 30 km and 60 km rather than equal distances at these speeds, its average speed would have been 45 km/h.
[BL] [OL] Caution students that the terms speed, average speed, and instantaneous speed are all often referred to simply as speed in everyday language. Emphasize the importance in science to use correct terminology to avoid confusion and to properly communicate ideas.
Worked Example
Calculating average speed.
A marble rolls 5.2 m in 1.8 s. What was the marble's average speed?
We know the distance the marble travels, 5.2 m, and the time interval, 1.8 s. We can use these values in the average speed equation.
Average speed is a scalar, so we do not include direction in the answer. We can check the reasonableness of the answer by estimating: 5 meters divided by 2 seconds is 2.5 m/s. Since 2.5 m/s is close to 2.9 m/s, the answer is reasonable. This is about the speed of a brisk walk, so it also makes sense.
Practice Problems
A pitcher throws a baseball from the pitcher’s mound to home plate in 0.46 s. The distance is 18.4 m. What was the average speed of the baseball?
The vector version of speed is velocity. Velocity describes the speed and direction of an object. As with speed, it is useful to describe either the average velocity over a time period or the velocity at a specific moment. Average velocity is displacement divided by the time over which the displacement occurs.
Velocity, like speed, has SI units of meters per second (m/s), but because it is a vector, you must also include a direction. Furthermore, the variable v for velocity is bold because it is a vector, which is in contrast to the variable v for speed which is italicized because it is a scalar quantity.
Tips For Success
It is important to keep in mind that the average speed is not the same thing as the average velocity without its direction. Like we saw with displacement and distance in the last section, changes in direction over a time interval have a bigger effect on speed and velocity.
Suppose a passenger moved toward the back of a plane with an average velocity of –4 m/s. We cannot tell from the average velocity whether the passenger stopped momentarily or backed up before he got to the back of the plane. To get more details, we must consider smaller segments of the trip over smaller time intervals such as those shown in Figure 2.9 . If you consider infinitesimally small intervals, you can define instantaneous velocity , which is the velocity at a specific instant in time. Instantaneous velocity and average velocity are the same if the velocity is constant.
Earlier, you have read that distance traveled can be different than the magnitude of displacement. In the same way, speed can be different than the magnitude of velocity. For example, you drive to a store and return home in half an hour. If your car’s odometer shows the total distance traveled was 6 km, then your average speed was 12 km/h. Your average velocity, however, was zero because your displacement for the round trip is zero.
Watch Physics
Calculating average velocity or speed.
This video reviews vectors and scalars and describes how to calculate average velocity and average speed when you know displacement and change in time. The video also reviews how to convert km/h to m/s.
- A scalar quantity is fully described by its magnitude, while a vector needs both magnitude and direction to fully describe it. Displacement is an example of a scalar quantity and time is an example of a vector quantity.
- A scalar quantity is fully described by its magnitude, while a vector needs both magnitude and direction to fully describe it. Time is an example of a scalar quantity and displacement is an example of a vector quantity.
- A scalar quantity is fully described by its magnitude and direction, while a vector needs only magnitude to fully describe it. Displacement is an example of a scalar quantity and time is an example of a vector quantity.
- A scalar quantity is fully described by its magnitude and direction, while a vector needs only magnitude to fully describe it. Time is an example of a scalar quantity and displacement is an example of a vector quantity.
This video does a good job of reinforcing the difference between vectors and scalars. The student is introduced to the idea of using ‘s’ to denote displacement, which you may or may not wish to encourage. Before students watch the video, point out that the instructor uses s → s → for displacement instead of d, as used in this text. Explain the use of small arrows over variables is a common way to denote vectors in higher-level physics courses. Caution students that the customary abbreviations for hour and seconds are not used in this video. Remind students that in their own work they should use the abbreviations h for hour and s for seconds.
Calculating Average Velocity
A student has a displacement of 304 m north in 180 s. What was the student's average velocity?
We know that the displacement is 304 m north and the time is 180 s. We can use the formula for average velocity to solve the problem.
Since average velocity is a vector quantity, you must include direction as well as magnitude in the answer. Notice, however, that the direction can be omitted until the end to avoid cluttering the problem. Pay attention to the significant figures in the problem. The distance 304 m has three significant figures, but the time interval 180 s has only two, so the quotient should have only two significant figures.
Note the way scalars and vectors are represented. In this book d represents distance and displacement. Similarly, v represents speed, and v represents velocity. A variable that is not bold indicates a scalar quantity, and a bold variable indicates a vector quantity. Vectors are sometimes represented by small arrows above the variable.
Use this problem to emphasize the importance of using the correct number of significant figures in calculations. Some students have a tendency to include many digits in their final calculations. They incorrectly believe they are improving the accuracy of their answer by writing many of the digits shown on the calculator. Point out that doing this introduces errors into the calculations. In more complicated calculations, these errors can propagate and cause the final answer to be wrong. Instead, remind students to always carry one or two extra digits in intermediate calculations and to round the final answer to the correct number of significant figures.
Solving for Displacement when Average Velocity and Time are Known
Layla jogs with an average velocity of 2.4 m/s east. What is her displacement after 46 seconds?
We know that Layla's average velocity is 2.4 m/s east, and the time interval is 46 seconds. We can rearrange the average velocity formula to solve for the displacement.
The answer is about 110 m east, which is a reasonable displacement for slightly less than a minute of jogging. A calculator shows the answer as 110.4 m. We chose to write the answer using scientific notation because we wanted to make it clear that we only used two significant figures.
Dimensional analysis is a good way to determine whether you solved a problem correctly. Write the calculation using only units to be sure they match on opposite sides of the equal mark. In the worked example, you have m = (m/s)(s). Since seconds is in the denominator for the average velocity and in the numerator for the time, the unit cancels out leaving only m and, of course, m = m.
Solving for Time when Displacement and Average Velocity are Known
Phillip walks along a straight path from his house to his school. How long will it take him to get to school if he walks 428 m west with an average velocity of 1.7 m/s west?
We know that Phillip's displacement is 428 m west, and his average velocity is 1.7 m/s west. We can calculate the time required for the trip by rearranging the average velocity equation.
Here again we had to use scientific notation because the answer could only have two significant figures. Since time is a scalar, the answer includes only a magnitude and not a direction.
- 4 km/h north
- 4 km/h south
- 64 km/h north
- 64 km/h south
A bird flies with an average velocity of 7.5 m/s east from one branch to another in 2.4 s. It then pauses before flying with an average velocity of 6.8 m/s east for 3.5 s to another branch. What is the bird’s total displacement from its starting point?
Virtual Physics
The walking man.
In this simulation you will put your cursor on the man and move him first in one direction and then in the opposite direction. Keep the Introduction tab active. You can use the Charts tab after you learn about graphing motion later in this chapter. Carefully watch the sign of the numbers in the position and velocity boxes. Ignore the acceleration box for now. See if you can make the man’s position positive while the velocity is negative. Then see if you can do the opposite.
Grasp Check
Which situation correctly describes when the moving man’s position was negative but his velocity was positive?
- Man moving toward 0 from left of 0
- Man moving toward 0 from right of 0
- Man moving away from 0 from left of 0
- Man moving away from 0 from right of 0
This is a powerful interactive animation, and it can be used for many lessons. At this point it can be used to show that displacement can be either positive or negative. It can also show that when displacement is negative, velocity can be either positive or negative. Later it can be used to show that velocity and acceleration can have different signs. It is strongly suggested that you keep students on the Introduction tab. The Charts tab can be used after students learn about graphing motion later in this chapter.
Check Your Understanding
- Yes, because average velocity depends on the net or total displacement.
- Yes, because average velocity depends on the total distance traveled.
- No, because the velocities of both runners must remain exactly the same throughout the journey.
- No, because the instantaneous velocities of the runners must remain the same at the midpoint but can vary at other points.
If you divide the total distance traveled on a car trip (as determined by the odometer) by the time for the trip, are you calculating the average speed or the magnitude of the average velocity, and under what circumstances are these two quantities the same?
- Average speed. Both are the same when the car is traveling at a constant speed and changing direction.
- Average speed. Both are the same when the speed is constant and the car does not change its direction.
- Magnitude of average velocity. Both are same when the car is traveling at a constant speed.
- Magnitude of average velocity. Both are same when the car does not change its direction.
- Yes, if net displacement is negative.
- Yes, if the object’s direction changes during motion.
- No, because average velocity describes only the magnitude and not the direction of motion.
- No, because average velocity only describes the magnitude in the positive direction of motion.
Use the Check Your Understanding questions to assess students’ achievement of the sections learning objectives. If students are struggling with a specific objective, the Check Your Understanding will help identify which and direct students to the relevant content. Assessment items in TUTOR will allow you to reassess.
As an Amazon Associate we earn from qualifying purchases.
This book may not be used in the training of large language models or otherwise be ingested into large language models or generative AI offerings without OpenStax's permission.
Want to cite, share, or modify this book? This book uses the Creative Commons Attribution License and you must attribute Texas Education Agency (TEA). The original material is available at: https://www.texasgateway.org/book/tea-physics . Changes were made to the original material, including updates to art, structure, and other content updates.
Access for free at https://openstax.org/books/physics/pages/1-introduction
- Authors: Paul Peter Urone, Roger Hinrichs
- Publisher/website: OpenStax
- Book title: Physics
- Publication date: Mar 26, 2020
- Location: Houston, Texas
- Book URL: https://openstax.org/books/physics/pages/1-introduction
- Section URL: https://openstax.org/books/physics/pages/2-2-speed-and-velocity
© Jan 19, 2024 Texas Education Agency (TEA). The OpenStax name, OpenStax logo, OpenStax book covers, OpenStax CNX name, and OpenStax CNX logo are not subject to the Creative Commons license and may not be reproduced without the prior and express written consent of Rice University.

Physics Problems with Solutions
Velocity and speed: solutions to problems.
Solutions to the problems on velocity and speed of moving objects. More tutorials can be found in this website.
A man walks 7 km in 2 hours and 2 km in 1 hour in the same direction. a) What is the man's average speed for the whole journey? b) What is the man's average velocity for the whole journey? Solution to Problem 1: a)

You start walking from a point on a circular field of radius 0.5 km and 1 hour later you are at the same point. a) What is your average speed for the whole journey? b) What is your average velocity for the whole journey? Solution to Problem 3: a) If you walk around a circular field and come back to the same point, you have covered a distance equal to the circumference of the circle.
John drove South 120 km at 60 km/h and then East 150 km at 50 km/h. Determine a) the average speed for the whole journey? b) the magnitude of the average velocity for the whole journey? Solution to Problem 4: a)
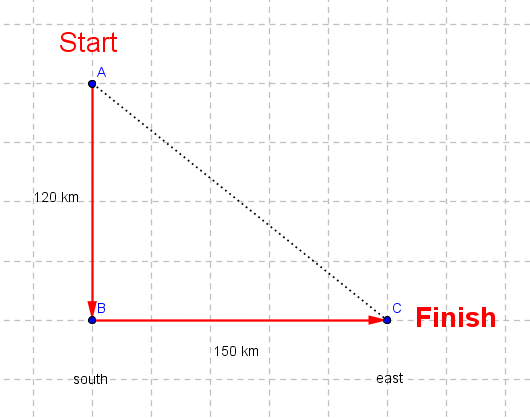
If I can walk at an average speed of 5 km/h, how many miles I can walk in two hours? Solution to Problem 5: distance = (average speed) * (time) = 5 km/h * 2 hours = 10 km using the rate of conversion 0.62 miles per km, the distance in miles is given by distance = 10 km * 0.62 miles/km = 6.2 miles
A train travels along a straight line at a constant speed of 60 mi/h for a distance d and then another distance equal to 2d in the same direction at a constant speed of 80 mi/h. a)What is the average speed of the train for the whole journey?
A car travels 22 km south, 12 km west, and 14 km north in half an hour. a) What is the average speed of the car? b) What is the final displacement of the car? c) What is the average velocity of the car?
Solution to Problem 7: a)
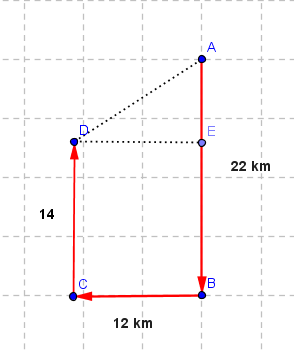
Solution to Problem 8: a)
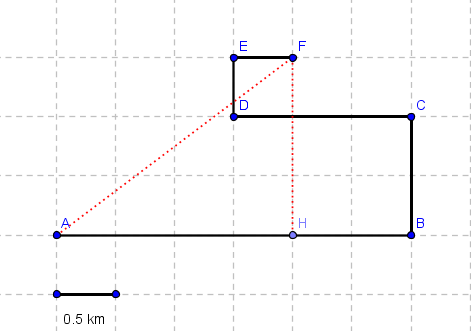
More References and links
- Velocity and Speed: Tutorials with Examples
- Velocity and Speed: Problems with Solutions
- Acceleration: Tutorials with Examples
- Uniform Acceleration Motion: Problems with Solutions
- Uniform Acceleration Motion: Equations with Explanations
Popular Pages
- Privacy Policy

- Why Does Water Expand When It Freezes
- Gold Foil Experiment
- Faraday Cage
- Oil Drop Experiment
- Magnetic Monopole
- Why Do Fireflies Light Up
- Types of Blood Cells With Their Structure, and Functions
- The Main Parts of a Plant With Their Functions
- Parts of a Flower With Their Structure and Functions
- Parts of a Leaf With Their Structure and Functions
- Why Does Ice Float on Water
- Why Does Oil Float on Water
- How Do Clouds Form
- What Causes Lightning
- How are Diamonds Made
- Types of Meteorites
- Types of Volcanoes
- Types of Rocks
Velocity is the rate at which an object changes position with time. An object is displaced when it changes its position. The amount of displacement over the time in which the displacement occurred gives the velocity. It is a vector quantity that has both magnitude and direction.
The importance of velocity is that it can give you an estimated time to go from one point to another. Suppose you are traveling from place A to place B. Velocity tells you how long it will take to arrive at your destination.
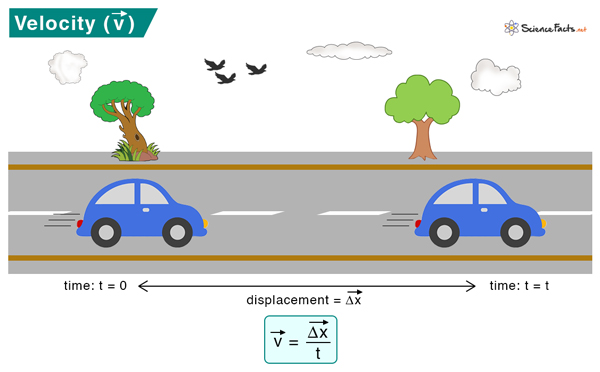
How to Calculate Velocity
Velocity can be calculated by measuring the object’s displacement over the time taken to displace it.
In vectorial notation, velocity is given by
The SI unit of velocity is Newtons per second of N/s. The cgs unit is ergs per second or ergs/s. Other units include miler per hour or mph, kilometers per hour or kph, and feet per second or fps.

- A car traveling on the highway at 60 mph toward the West
- A biker riding on the road at 35 mph toward the southwest
- A bicyclist heading northwards at 14 mph
- An airplane flying eastwards at 300 mph
- A rocket launched into the sky in the south-east direction
- A train traveling in the south direction at 80 kph
- A runner runs 420 m in 70 seconds
Average and Instantaneous Velocity
An object can move from one position to another in several steps. The average velocity is simply the change in position over the time interval within which change takes place.
v avg is the average velocity
x f is the final velocity
x i is the initial velocity
t f is the final time
t i is the initial time
If the starting time is zero, then t i = 0.
The instantaneous (v ins. ) velocity is the velocity at a particular instant of time. It can be obtained by taking the limit of the above expression in the limit Δt → 0.
Speed vs. Velocity
Both speed and velocity measure rate. Both of them depict how fast an object is moving. However, there is an essential difference. While speed tells us the magnitude of the rate at which the object moves, it does not say anything about the direction. On the other hand, velocity considers the direction of motion.
Let us take an example to illustrate this. When we say a car is moving on the road at 35 mph, we specify the magnitude but not the direction. However, when we say a car is moving on the road eastwards at 35 mph, we specify both the magnitude and direction. The first sentence refers to the speed, and the second refers to the velocity. Therefore, speed is a scalar quantity, and velocity is a vector quantity.
The formula for speed is the distance traveled over the time taken to travel that distance.
Velocity vs. Time Graph
The following graph shows how velocity changes with time. At some parts of the graph, the velocity increases linearly with time. The quotient of velocity and time is called acceleration . When the velocity decreases with time, the phenomenon is known as deceleration. Regions of constant velocity are also indicated on the graph.

Solved Problems
Problem 1. Suppose a runner moves along the x-direction over some time.During a 5.00 s interval, the runner’s position changes from x 1 = 75 m to x 2 = 35 m. What was the runner’s average velocity?
Given x 1 = 75 m, x 2 = 35 m, Δt = 5 s
The average velocity is given by
v avg = (x 2 – x 1 )/Δt = (35 m – 75 m)/5s = – 8 m/s
Therefore, the runner is running in the negative x-direction.
Problem 2 . A commuter train travels from New York to Philadelphia in 1 hour and 25 minutes and from Philadelphia to New York in 1 hour and 35 minutes. The distance between the two stations is approximately 96 miles. What is (a) the average velocity of the train and (b) the average speed of the train in m/s? (1 mile = 1.6 km)
a) The average velocity of the train is zero since the displacement is zero. (The train returns to New York).
d = 96 miles = 96 x 1.6 = 153.6 km = 153.6 x 10 3 m
t 1 = 1 hr 25 mins = 60 mins + 25 mins = 85 mins = 85 x 60 = 5100 s
Therefore, v 1 = 153.6 x 10 3 m / 5100 s = 30.2 m/s
t 2 = 1 hr 35 mins = 60 mins + 35 mins = 95 mins = 95 x 60 = 5700 s
Therefore, v 2 = 153.6 x 10 3 m / 5700 s = 26.9 m/s
The average speed is (30.2 m/s + 26.9 m/s)/2 = 28.55 m/s
- Speed vs. Velocity – Physicsclassroom.com
- What is Velocity? – Khanacademy.org
- What is Velocity in Physics? – Thoughtco.com
- Speed and Velocity – Physics.info
- Instantaneous Velocity and Speed – Pressbooks.online.ucf.edu
- Time, Velocity, and Speed – Phys.libretexts.org
- Velocity – Hyperphysics.phy-astr.gsu.edu
Article was last reviewed on Friday, July 28, 2023
Related articles

2 responses to “Velocity”
on concept of vector
A vector is a physical quantity that has both magnitude and direction.
Leave a Reply Cancel reply
Your email address will not be published. Required fields are marked *
Save my name, email, and website in this browser for the next time I comment.
Popular Articles

Join our Newsletter
Fill your E-mail Address
Related Worksheets
- Privacy Policy
© 2024 ( Science Facts ). All rights reserved. Reproduction in whole or in part without permission is prohibited.
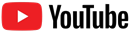
- TPC and eLearning
- What's NEW at TPC?
- Read Watch Interact
- Practice Review Test
- Teacher-Tools
- Subscription Selection
- Seat Calculator
- Ad Free Account
- Edit Profile Settings
- Classes (Version 2)
- Student Progress Edit
- Task Properties
- Export Student Progress
- Task, Activities, and Scores
- Metric Conversions Questions
- Metric System Questions
- Metric Estimation Questions
- Significant Digits Questions
- Proportional Reasoning
- Acceleration
- Distance-Displacement
- Dots and Graphs
- Graph That Motion
- Match That Graph
- Name That Motion
- Motion Diagrams
- Pos'n Time Graphs Numerical
- Pos'n Time Graphs Conceptual
- Up And Down - Questions
- Balanced vs. Unbalanced Forces
- Change of State
- Force and Motion
- Mass and Weight
- Match That Free-Body Diagram
- Net Force (and Acceleration) Ranking Tasks
- Newton's Second Law
- Normal Force Card Sort
- Recognizing Forces
- Air Resistance and Skydiving
- Solve It! with Newton's Second Law
- Which One Doesn't Belong?
- Component Addition Questions
- Head-to-Tail Vector Addition
- Projectile Mathematics
- Trajectory - Angle Launched Projectiles
- Trajectory - Horizontally Launched Projectiles
- Vector Addition
- Vector Direction
- Which One Doesn't Belong? Projectile Motion
- Forces in 2-Dimensions
- Being Impulsive About Momentum
- Explosions - Law Breakers
- Hit and Stick Collisions - Law Breakers
- Case Studies: Impulse and Force
- Impulse-Momentum Change Table
- Keeping Track of Momentum - Hit and Stick
- Keeping Track of Momentum - Hit and Bounce
- What's Up (and Down) with KE and PE?
- Energy Conservation Questions
- Energy Dissipation Questions
- Energy Ranking Tasks
- LOL Charts (a.k.a., Energy Bar Charts)
- Match That Bar Chart
- Words and Charts Questions
- Name That Energy
- Stepping Up with PE and KE Questions
- Case Studies - Circular Motion
- Circular Logic
- Forces and Free-Body Diagrams in Circular Motion
- Gravitational Field Strength
- Universal Gravitation
- Angular Position and Displacement
- Linear and Angular Velocity
- Angular Acceleration
- Rotational Inertia
- Balanced vs. Unbalanced Torques
- Getting a Handle on Torque
- Torque-ing About Rotation
- Properties of Matter
- Fluid Pressure
- Buoyant Force
- Sinking, Floating, and Hanging
- Pascal's Principle
- Flow Velocity
- Bernoulli's Principle
- Balloon Interactions
- Charge and Charging
- Charge Interactions
- Charging by Induction
- Conductors and Insulators
- Coulombs Law
- Electric Field
- Electric Field Intensity
- Polarization
- Case Studies: Electric Power
- Know Your Potential
- Light Bulb Anatomy
- I = ∆V/R Equations as a Guide to Thinking
- Parallel Circuits - ∆V = I•R Calculations
- Resistance Ranking Tasks
- Series Circuits - ∆V = I•R Calculations
- Series vs. Parallel Circuits
- Equivalent Resistance
- Period and Frequency of a Pendulum
- Pendulum Motion: Velocity and Force
- Energy of a Pendulum
- Period and Frequency of a Mass on a Spring
- Horizontal Springs: Velocity and Force
- Vertical Springs: Velocity and Force
- Energy of a Mass on a Spring
- Decibel Scale
- Frequency and Period
- Closed-End Air Columns
- Name That Harmonic: Strings
- Rocking the Boat
- Wave Basics
- Matching Pairs: Wave Characteristics
- Wave Interference
- Waves - Case Studies
- Color Addition and Subtraction
- Color Filters
- If This, Then That: Color Subtraction
- Light Intensity
- Color Pigments
- Converging Lenses
- Curved Mirror Images
- Law of Reflection
- Refraction and Lenses
- Total Internal Reflection
- Who Can See Who?
- Formulas and Atom Counting
- Atomic Models
- Bond Polarity
- Entropy Questions
- Cell Voltage Questions
- Heat of Formation Questions
- Reduction Potential Questions
- Oxidation States Questions
- Measuring the Quantity of Heat
- Hess's Law
- Oxidation-Reduction Questions
- Galvanic Cells Questions
- Thermal Stoichiometry
- Molecular Polarity
- Quantum Mechanics
- Balancing Chemical Equations
- Bronsted-Lowry Model of Acids and Bases
- Classification of Matter
- Collision Model of Reaction Rates
- Density Ranking Tasks
- Dissociation Reactions
- Complete Electron Configurations
- Elemental Measures
- Enthalpy Change Questions
- Equilibrium Concept
- Equilibrium Constant Expression
- Equilibrium Calculations - Questions
- Equilibrium ICE Table
- Intermolecular Forces Questions
- Ionic Bonding
- Lewis Electron Dot Structures
- Limiting Reactants
- Line Spectra Questions
- Mass Stoichiometry
- Measurement and Numbers
- Metals, Nonmetals, and Metalloids
- Metric Estimations
- Metric System
- Molarity Ranking Tasks
- Mole Conversions
- Name That Element
- Names to Formulas
- Names to Formulas 2
- Nuclear Decay
- Particles, Words, and Formulas
- Periodic Trends
- Precipitation Reactions and Net Ionic Equations
- Pressure Concepts
- Pressure-Temperature Gas Law
- Pressure-Volume Gas Law
- Chemical Reaction Types
- Significant Digits and Measurement
- States Of Matter Exercise
- Stoichiometry Law Breakers
- Stoichiometry - Math Relationships
- Subatomic Particles
- Spontaneity and Driving Forces
- Gibbs Free Energy
- Volume-Temperature Gas Law
- Acid-Base Properties
- Energy and Chemical Reactions
- Chemical and Physical Properties
- Valence Shell Electron Pair Repulsion Theory
- Writing Balanced Chemical Equations
- Mission CG1
- Mission CG10
- Mission CG2
- Mission CG3
- Mission CG4
- Mission CG5
- Mission CG6
- Mission CG7
- Mission CG8
- Mission CG9
- Mission EC1
- Mission EC10
- Mission EC11
- Mission EC12
- Mission EC2
- Mission EC3
- Mission EC4
- Mission EC5
- Mission EC6
- Mission EC7
- Mission EC8
- Mission EC9
- Mission RL1
- Mission RL2
- Mission RL3
- Mission RL4
- Mission RL5
- Mission RL6
- Mission KG7
- Mission RL8
- Mission KG9
- Mission RL10
- Mission RL11
- Mission RM1
- Mission RM2
- Mission RM3
- Mission RM4
- Mission RM5
- Mission RM6
- Mission RM8
- Mission RM10
- Mission LC1
- Mission RM11
- Mission LC2
- Mission LC3
- Mission LC4
- Mission LC5
- Mission LC6
- Mission LC8
- Mission SM1
- Mission SM2
- Mission SM3
- Mission SM4
- Mission SM5
- Mission SM6
- Mission SM8
- Mission SM10
- Mission KG10
- Mission SM11
- Mission KG2
- Mission KG3
- Mission KG4
- Mission KG5
- Mission KG6
- Mission KG8
- Mission KG11
- Mission F2D1
- Mission F2D2
- Mission F2D3
- Mission F2D4
- Mission F2D5
- Mission F2D6
- Mission KC1
- Mission KC2
- Mission KC3
- Mission KC4
- Mission KC5
- Mission KC6
- Mission KC7
- Mission KC8
- Mission AAA
- Mission SM9
- Mission LC7
- Mission LC9
- Mission NL1
- Mission NL2
- Mission NL3
- Mission NL4
- Mission NL5
- Mission NL6
- Mission NL7
- Mission NL8
- Mission NL9
- Mission NL10
- Mission NL11
- Mission NL12
- Mission MC1
- Mission MC10
- Mission MC2
- Mission MC3
- Mission MC4
- Mission MC5
- Mission MC6
- Mission MC7
- Mission MC8
- Mission MC9
- Mission RM7
- Mission RM9
- Mission RL7
- Mission RL9
- Mission SM7
- Mission SE1
- Mission SE10
- Mission SE11
- Mission SE12
- Mission SE2
- Mission SE3
- Mission SE4
- Mission SE5
- Mission SE6
- Mission SE7
- Mission SE8
- Mission SE9
- Mission VP1
- Mission VP10
- Mission VP2
- Mission VP3
- Mission VP4
- Mission VP5
- Mission VP6
- Mission VP7
- Mission VP8
- Mission VP9
- Mission WM1
- Mission WM2
- Mission WM3
- Mission WM4
- Mission WM5
- Mission WM6
- Mission WM7
- Mission WM8
- Mission WE1
- Mission WE10
- Mission WE2
- Mission WE3
- Mission WE4
- Mission WE5
- Mission WE6
- Mission WE7
- Mission WE8
- Mission WE9
- Vector Walk Interactive
- Name That Motion Interactive
- Kinematic Graphing 1 Concept Checker
- Kinematic Graphing 2 Concept Checker
- Graph That Motion Interactive
- Two Stage Rocket Interactive
- Rocket Sled Concept Checker
- Force Concept Checker
- Free-Body Diagrams Concept Checker
- Free-Body Diagrams The Sequel Concept Checker
- Skydiving Concept Checker
- Elevator Ride Concept Checker
- Vector Addition Concept Checker
- Vector Walk in Two Dimensions Interactive
- Name That Vector Interactive
- River Boat Simulator Concept Checker
- Projectile Simulator 2 Concept Checker
- Projectile Simulator 3 Concept Checker
- Hit the Target Interactive
- Turd the Target 1 Interactive
- Turd the Target 2 Interactive
- Balance It Interactive
- Go For The Gold Interactive
- Egg Drop Concept Checker
- Fish Catch Concept Checker
- Exploding Carts Concept Checker
- Collision Carts - Inelastic Collisions Concept Checker
- Its All Uphill Concept Checker
- Stopping Distance Concept Checker
- Chart That Motion Interactive
- Roller Coaster Model Concept Checker
- Uniform Circular Motion Concept Checker
- Horizontal Circle Simulation Concept Checker
- Vertical Circle Simulation Concept Checker
- Race Track Concept Checker
- Gravitational Fields Concept Checker
- Orbital Motion Concept Checker
- Angular Acceleration Concept Checker
- Balance Beam Concept Checker
- Torque Balancer Concept Checker
- Aluminum Can Polarization Concept Checker
- Charging Concept Checker
- Name That Charge Simulation
- Coulomb's Law Concept Checker
- Electric Field Lines Concept Checker
- Put the Charge in the Goal Concept Checker
- Circuit Builder Concept Checker (Series Circuits)
- Circuit Builder Concept Checker (Parallel Circuits)
- Circuit Builder Concept Checker (∆V-I-R)
- Circuit Builder Concept Checker (Voltage Drop)
- Equivalent Resistance Interactive
- Pendulum Motion Simulation Concept Checker
- Mass on a Spring Simulation Concept Checker
- Particle Wave Simulation Concept Checker
- Boundary Behavior Simulation Concept Checker
- Slinky Wave Simulator Concept Checker
- Simple Wave Simulator Concept Checker
- Wave Addition Simulation Concept Checker
- Standing Wave Maker Simulation Concept Checker
- Color Addition Concept Checker
- Painting With CMY Concept Checker
- Stage Lighting Concept Checker
- Filtering Away Concept Checker
- InterferencePatterns Concept Checker
- Young's Experiment Interactive
- Plane Mirror Images Interactive
- Who Can See Who Concept Checker
- Optics Bench (Mirrors) Concept Checker
- Name That Image (Mirrors) Interactive
- Refraction Concept Checker
- Total Internal Reflection Concept Checker
- Optics Bench (Lenses) Concept Checker
- Kinematics Preview
- Velocity Time Graphs Preview
- Moving Cart on an Inclined Plane Preview
- Stopping Distance Preview
- Cart, Bricks, and Bands Preview
- Fan Cart Study Preview
- Friction Preview
- Coffee Filter Lab Preview
- Friction, Speed, and Stopping Distance Preview
- Up and Down Preview
- Projectile Range Preview
- Ballistics Preview
- Juggling Preview
- Marshmallow Launcher Preview
- Air Bag Safety Preview
- Colliding Carts Preview
- Collisions Preview
- Engineering Safer Helmets Preview
- Push the Plow Preview
- Its All Uphill Preview
- Energy on an Incline Preview
- Modeling Roller Coasters Preview
- Hot Wheels Stopping Distance Preview
- Ball Bat Collision Preview
- Energy in Fields Preview
- Weightlessness Training Preview
- Roller Coaster Loops Preview
- Universal Gravitation Preview
- Keplers Laws Preview
- Kepler's Third Law Preview
- Charge Interactions Preview
- Sticky Tape Experiments Preview
- Wire Gauge Preview
- Voltage, Current, and Resistance Preview
- Light Bulb Resistance Preview
- Series and Parallel Circuits Preview
- Thermal Equilibrium Preview
- Linear Expansion Preview
- Heating Curves Preview
- Electricity and Magnetism - Part 1 Preview
- Electricity and Magnetism - Part 2 Preview
- Vibrating Mass on a Spring Preview
- Period of a Pendulum Preview
- Wave Speed Preview
- Slinky-Experiments Preview
- Standing Waves in a Rope Preview
- Sound as a Pressure Wave Preview
- DeciBel Scale Preview
- DeciBels, Phons, and Sones Preview
- Sound of Music Preview
- Shedding Light on Light Bulbs Preview
- Models of Light Preview
- Electromagnetic Radiation Preview
- Electromagnetic Spectrum Preview
- EM Wave Communication Preview
- Digitized Data Preview
- Light Intensity Preview
- Concave Mirrors Preview
- Object Image Relations Preview
- Snells Law Preview
- Reflection vs. Transmission Preview
- Magnification Lab Preview
- Reactivity Preview
- Ions and the Periodic Table Preview
- Periodic Trends Preview
- Intermolecular Forces Preview
- Melting Points and Boiling Points Preview
- Reaction Rates Preview
- Ammonia Factory Preview
- Stoichiometry Preview
- Nuclear Chemistry Preview
- Gaining Teacher Access
- Tasks and Classes
- Tasks - Classic
- Subscription
- Subscription Locator
- 1-D Kinematics
- Newton's Laws
- Vectors - Motion and Forces in Two Dimensions
- Momentum and Its Conservation
- Work and Energy
- Circular Motion and Satellite Motion
- Thermal Physics
- Static Electricity
- Electric Circuits
- Vibrations and Waves
- Sound Waves and Music
- Light and Color
- Reflection and Mirrors
- About the Physics Interactives
- Task Tracker
- Usage Policy
- Newtons Laws
- Vectors and Projectiles
- Forces in 2D
- Momentum and Collisions
- Circular and Satellite Motion
- Balance and Rotation
- Electromagnetism
- Waves and Sound
- Atomic Physics
- Forces in Two Dimensions
- Work, Energy, and Power
- Circular Motion and Gravitation
- Sound Waves
- 1-Dimensional Kinematics
- Circular, Satellite, and Rotational Motion
- Einstein's Theory of Special Relativity
- Waves, Sound and Light
- QuickTime Movies
- About the Concept Builders
- Pricing For Schools
- Directions for Version 2
- Measurement and Units
- Relationships and Graphs
- Rotation and Balance
- Vibrational Motion
- Reflection and Refraction
- Teacher Accounts
- Task Tracker Directions
- Kinematic Concepts
- Kinematic Graphing
- Wave Motion
- Sound and Music
- About CalcPad
- 1D Kinematics
- Vectors and Forces in 2D
- Simple Harmonic Motion
- Rotational Kinematics
- Rotation and Torque
- Rotational Dynamics
- Electric Fields, Potential, and Capacitance
- Transient RC Circuits
- Light Waves
- Units and Measurement
- Stoichiometry
- Molarity and Solutions
- Thermal Chemistry
- Acids and Bases
- Kinetics and Equilibrium
- Solution Equilibria
- Oxidation-Reduction
- Nuclear Chemistry
- Newton's Laws of Motion
- Work and Energy Packet
- Static Electricity Review
- NGSS Alignments
- 1D-Kinematics
- Projectiles
- Circular Motion
- Magnetism and Electromagnetism
- Graphing Practice
- About the ACT
- ACT Preparation
- For Teachers
- Other Resources
- Solutions Guide
- Solutions Guide Digital Download
- Motion in One Dimension
- Work, Energy and Power
- Algebra Based Physics
- Other Tools
- Frequently Asked Questions
- Purchasing the Download
- Purchasing the CD
- Purchasing the Digital Download
- About the NGSS Corner
- NGSS Search
- Force and Motion DCIs - High School
- Energy DCIs - High School
- Wave Applications DCIs - High School
- Force and Motion PEs - High School
- Energy PEs - High School
- Wave Applications PEs - High School
- Crosscutting Concepts
- The Practices
- Physics Topics
- NGSS Corner: Activity List
- NGSS Corner: Infographics
- About the Toolkits
- Position-Velocity-Acceleration
- Position-Time Graphs
- Velocity-Time Graphs
- Newton's First Law
- Newton's Second Law
- Newton's Third Law
- Terminal Velocity
- Projectile Motion
- Forces in 2 Dimensions
- Impulse and Momentum Change
- Momentum Conservation
- Work-Energy Fundamentals
- Work-Energy Relationship
- Roller Coaster Physics
- Satellite Motion
- Electric Fields
- Circuit Concepts
- Series Circuits
- Parallel Circuits
- Describing-Waves
- Wave Behavior Toolkit
- Standing Wave Patterns
- Resonating Air Columns
- Wave Model of Light
- Plane Mirrors
- Curved Mirrors
- Teacher Guide
- Using Lab Notebooks
- Current Electricity
- Light Waves and Color
- Reflection and Ray Model of Light
- Refraction and Ray Model of Light
- Classes (Legacy Version)
- Teacher Resources
- Subscriptions

- Newton's Laws
- Einstein's Theory of Special Relativity
- About Concept Checkers
- School Pricing
- Newton's Laws of Motion
- Newton's First Law
- Newton's Third Law
Kinematic Equations and Problem-Solving
- Kinematic Equations Introduction
- Solving Problems with Kinematic Equations
- Kinematic Equations and Free Fall
- Sample Problems and Solutions
- Kinematic Equations and Kinematic Graphs
The four kinematic equations that describe the mathematical relationship between the parameters that describe an object's motion were introduced in the previous part of Lesson 6 . The four kinematic equations are:
In the above equations, the symbol d stands for the displacement of the object. The symbol t stands for the time for which the object moved. The symbol a stands for the acceleration of the object. And the symbol v stands for the instantaneous velocity of the object; a subscript of i after the v (as in v i ) indicates that the velocity value is the initial velocity value and a subscript of f (as in v f ) indicates that the velocity value is the final velocity value.
Problem-Solving Strategy
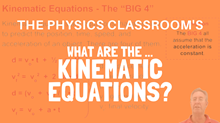
- Construct an informative diagram of the physical situation.
- Identify and list the given information in variable form.
- Identify and list the unknown information in variable form.
- Identify and list the equation that will be used to determine unknown information from known information.
- Substitute known values into the equation and use appropriate algebraic steps to solve for the unknown information.
- Check your answer to insure that it is reasonable and mathematically correct.
The use of this problem-solving strategy in the solution of the following problem is modeled in Examples A and B below.
Example Problem A
Ima Hurryin is approaching a stoplight moving with a velocity of +30.0 m/s. The light turns yellow, and Ima applies the brakes and skids to a stop. If Ima's acceleration is -8.00 m/s 2 , then determine the displacement of the car during the skidding process. (Note that the direction of the velocity and the acceleration vectors are denoted by a + and a - sign.)
The solution to this problem begins by the construction of an informative diagram of the physical situation. This is shown below. The second step involves the identification and listing of known information in variable form. Note that the v f value can be inferred to be 0 m/s since Ima's car comes to a stop. The initial velocity ( v i ) of the car is +30.0 m/s since this is the velocity at the beginning of the motion (the skidding motion). And the acceleration ( a ) of the car is given as - 8.00 m/s 2 . (Always pay careful attention to the + and - signs for the given quantities.) The next step of the strategy involves the listing of the unknown (or desired) information in variable form. In this case, the problem requests information about the displacement of the car. So d is the unknown quantity. The results of the first three steps are shown in the table below.
The next step of the strategy involves identifying a kinematic equation that would allow you to determine the unknown quantity. There are four kinematic equations to choose from. In general, you will always choose the equation that contains the three known and the one unknown variable. In this specific case, the three known variables and the one unknown variable are v f , v i , a , and d . Thus, you will look for an equation that has these four variables listed in it. An inspection of the four equations above reveals that the equation on the top right contains all four variables.
Once the equation is identified and written down, the next step of the strategy involves substituting known values into the equation and using proper algebraic steps to solve for the unknown information. This step is shown below.
(0 m/s) 2 = (30.0 m/s) 2 + 2 • (-8.00 m/s 2 ) • d
0 m 2 /s 2 = 900 m 2 /s 2 + (-16.0 m/s 2 ) • d
(16.0 m/s 2 ) • d = 900 m 2 /s 2 - 0 m 2 /s 2
(16.0 m/s 2 )*d = 900 m 2 /s 2
d = (900 m 2 /s 2 )/ (16.0 m/s 2 )
The solution above reveals that the car will skid a distance of 56.3 meters. (Note that this value is rounded to the third digit.)
The last step of the problem-solving strategy involves checking the answer to assure that it is both reasonable and accurate. The value seems reasonable enough. It takes a car a considerable distance to skid from 30.0 m/s (approximately 65 mi/hr) to a stop. The calculated distance is approximately one-half a football field, making this a very reasonable skidding distance. Checking for accuracy involves substituting the calculated value back into the equation for displacement and insuring that the left side of the equation is equal to the right side of the equation. Indeed it is!
Example Problem B
Ben Rushin is waiting at a stoplight. When it finally turns green, Ben accelerated from rest at a rate of a 6.00 m/s 2 for a time of 4.10 seconds. Determine the displacement of Ben's car during this time period.
Once more, the solution to this problem begins by the construction of an informative diagram of the physical situation. This is shown below. The second step of the strategy involves the identification and listing of known information in variable form. Note that the v i value can be inferred to be 0 m/s since Ben's car is initially at rest. The acceleration ( a ) of the car is 6.00 m/s 2 . And the time ( t ) is given as 4.10 s. The next step of the strategy involves the listing of the unknown (or desired) information in variable form. In this case, the problem requests information about the displacement of the car. So d is the unknown information. The results of the first three steps are shown in the table below.
The next step of the strategy involves identifying a kinematic equation that would allow you to determine the unknown quantity. There are four kinematic equations to choose from. Again, you will always search for an equation that contains the three known variables and the one unknown variable. In this specific case, the three known variables and the one unknown variable are t, v i , a, and d. An inspection of the four equations above reveals that the equation on the top left contains all four variables.
d = (0 m/s) • (4.1 s) + ½ • (6.00 m/s 2 ) • (4.10 s) 2
d = (0 m) + ½ • (6.00 m/s 2 ) • (16.81 s 2 )
d = 0 m + 50.43 m
The solution above reveals that the car will travel a distance of 50.4 meters. (Note that this value is rounded to the third digit.)
The last step of the problem-solving strategy involves checking the answer to assure that it is both reasonable and accurate. The value seems reasonable enough. A car with an acceleration of 6.00 m/s/s will reach a speed of approximately 24 m/s (approximately 50 mi/hr) in 4.10 s. The distance over which such a car would be displaced during this time period would be approximately one-half a football field, making this a very reasonable distance. Checking for accuracy involves substituting the calculated value back into the equation for displacement and insuring that the left side of the equation is equal to the right side of the equation. Indeed it is!
The two example problems above illustrate how the kinematic equations can be combined with a simple problem-solving strategy to predict unknown motion parameters for a moving object. Provided that three motion parameters are known, any of the remaining values can be determined. In the next part of Lesson 6 , we will see how this strategy can be applied to free fall situations. Or if interested, you can try some practice problems and check your answer against the given solutions.
Speed and Velocity
Speed is how fast something moves.
Velocity is speed with a direction .
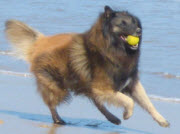
Saying Ariel the Dog runs at 9 km/h (kilometers per hour) is a speed.
But saying he runs 9 km/h Westwards is a velocity.
Imagine something moving back and forth very fast: it has a high speed, but a low (or zero) velocity.
Speed is measured as distance moved over time.
Speed = Distance Time
Example: A car travels 50 km in one hour.
Its average speed is 50 km per hour (50 km/h)
Speed = Distance Time = 50 km 1 hour
We can also use these symbols:
Speed = Δs Δt
Where Δ (" Delta ") means "change in", and
- s means distance ("s" for "space")
- t means time
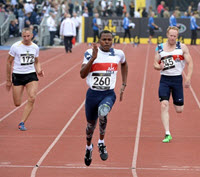
Example: You run 360 m in 60 seconds.
So your speed is 6 meters per second (6 m/s).
Speed is commonly measured in:
- meters per second (m/s or m s -1 ), or
- kilometers per hour (km/h or km h -1 )
A km is 1000 m, and there are 3600 seconds in an hour, so we can convert like this (see Unit Conversion Method to learn more):
So 1 m/s is equal to 3.6 km/h
Example: What is 20 m/s in km/h ?
20 m/s × 3.6 km/h 1 m/s = 72 km/h
Example: What is 120 km/h in m/s ?
120 km/h × 1 m/s 3.6 km/h = 33.333... m/s
Average vs Instantaneous Speed
The examples so far calculate average speed : how far something travels over a period of time.
But speed can change as time goes by. A car can go faster and slower, maybe even stop at lights.
So there is also instantaneous speed : the speed at an instant in time. We can try to measure it by using a very short span of time (the shorter the better).
Example: Sam uses a stopwatch and measures 1.6 seconds as the car travels between two posts 20 m apart. What is the instantaneous speed ?
Well, we don't know exactly, as the car may have been speeding up or slowing down during that time, but we can estimate:
20 m 1.6 s = 12.5 m/s = 45 km/h
It is really still an average, but is close to an instantaneous speed.
Constant Speed
When the speed does not change it is constant .
For constant speed, the average and instantaneous speeds are the same.
Because the direction is important velocity uses displacement instead of distance:
Velocity = Displacement Time in a direction.
Example: You walk from home to the shop in 100 seconds, what is your speed and what is your velocity?
Speed = 220 m 100 s = 2.2 m/s
Velocity = 130 m 100 s East = 1.3 m/s East
You forgot your money so you turn around and go back home in 120 more seconds: what is your round-trip speed and velocity?
The total time is 100 s + 120 s = 220 s:
Speed = 440 m 220 s = 2.0 m/s
Velocity = 0 m 220 s = 0 m/s
Yes, the velocity is zero as you ended up where you started.
Learn more at Vectors .
Motion is relative. When we say something is "at rest" or "moving at 4 m/s" we forget to say "in relation to me" or "in relation to the ground", etc.
Think about this: are you really standing still? You are on planet Earth which is spinning at 40,075 km per day (about 1675 km/h or 465 m/s), and moving around the Sun at about 100,000 km/h, which is itself moving through the Galaxy.
Next time you are out walking, imagine you are still and it is the world that moves under your feet. Feels great.
It is all relative!
- PRO Courses Guides New Tech Help Pro Expert Videos About wikiHow Pro Upgrade Sign In
- EDIT Edit this Article
- EXPLORE Tech Help Pro About Us Random Article Quizzes Request a New Article Community Dashboard This Or That Game Popular Categories Arts and Entertainment Artwork Books Movies Computers and Electronics Computers Phone Skills Technology Hacks Health Men's Health Mental Health Women's Health Relationships Dating Love Relationship Issues Hobbies and Crafts Crafts Drawing Games Education & Communication Communication Skills Personal Development Studying Personal Care and Style Fashion Hair Care Personal Hygiene Youth Personal Care School Stuff Dating All Categories Arts and Entertainment Finance and Business Home and Garden Relationship Quizzes Cars & Other Vehicles Food and Entertaining Personal Care and Style Sports and Fitness Computers and Electronics Health Pets and Animals Travel Education & Communication Hobbies and Crafts Philosophy and Religion Work World Family Life Holidays and Traditions Relationships Youth
- Browse Articles
- Learn Something New
- Quizzes Hot
- This Or That Game
- Train Your Brain
- Explore More
- Support wikiHow
- About wikiHow
- Log in / Sign up
- Education and Communications
- Classical Mechanics
How to Calculate Velocity
Last Updated: May 2, 2024 Fact Checked
This article was co-authored by Sean Alexander, MS . Sean Alexander is an Academic Tutor specializing in teaching mathematics and physics. Sean is the Owner of Alexander Tutoring, an academic tutoring business that provides personalized studying sessions focused on mathematics and physics. With over 15 years of experience, Sean has worked as a physics and math instructor and tutor for Stanford University, San Francisco State University, and Stanbridge Academy. He holds a BS in Physics from the University of California, Santa Barbara and an MS in Theoretical Physics from San Francisco State University. There are 10 references cited in this article, which can be found at the bottom of the page. This article has been fact-checked, ensuring the accuracy of any cited facts and confirming the authority of its sources. This article has been viewed 1,536,854 times.

Quick Formulas
- a = acceleration t = time
Finding Average Velocity

- The car was displaced by (41m - 5m) = 36 meters east.
- The diver ended up 4 meters below the starting point, so her displacement is 4 meters downward, or -4 meters. (0 + 1 - 5 = -4). Even though the diver traveled six meters (one up, then five down), what matters is that the end point is four meters below the start point.

- Example 1 (cont.): The problem tells us that the car took 8 seconds to go from the start point to the end point, so this is the change in time.
- Example 2 (cont.): If the diver jumped at t = 7 seconds and hits the water at t = 8 seconds, the change in time = 8s - 7s = 1 second.

- Draw a diagram and connect the start point and end point with a straight line. This is the hypotenuse of a triangle, so solve for its length of this line using properties of right triangles . In this case, the displacement is 5 meters northeast.
- At some point, your math teacher may require you to find the exact direction traveled (the angle above the horizontal). You can do this by using geometry or by adding vectors.
Finding Velocity from Acceleration

- a = 10 m/s 2
- (a * t) = (10 m/s 2 * 5 s) = 50 m/s increase in velocity.

- In our example, since the ship started going north and did not change direction, its final velocity is 52 m/s north.

Circular Velocity

- The circular velocity of an object is calculated by dividing the circumference of the circular path by the time period over which the object travels.
- v = (2πr) / T
- Note that 2πr equals the circumference of the circular path.
- r stands for "radius"
- T stands for "time period"

- Circumference = 2πr = ~ (2)(3.14)(8 m) = 50.24 m

- The circular velocity of the object is 1.12 m/s.
Calculator, Practice Problems, and Answers

Community Q&A

- Average velocity measures the average velocity an object travels over the full course of its path. Instantaneous velocity measures the velocity of an object at a specific moment along its path. Thanks Helpful 0 Not Helpful 0

You Might Also Like

- ↑ Sean Alexander, MS. Academic Tutor. Expert Interview. 14 May 2020.
- ↑ https://www.khanacademy.org/science/physics/one-dimensional-motion/displacement-velocity-time/a/what-is-velocity
- ↑ https://www.physicsclassroom.com/class/1DKin/Lesson-1/Speed-and-Velocity
- ↑ https://openstax.org/books/university-physics-volume-1/pages/3-1-position-displacement-and-average-velocity
- ↑ http://www.physicsclassroom.com/Class/1DKin/U1L1c.cfm
- ↑ https://www.omnicalculator.com/physics/velocity
- ↑ http://easycalculation.com/physics/classical-physics/circular-velocity.php
- ↑ https://www.omnicalculator.com/physics/angular-velocity
- ↑ https://math.libretexts.org/Bookshelves/Precalculus/Book%3A_Trigonometry_(Sundstrom_and_Schlicker)/01%3A_The_Trigonometric_Functions/1.04%3A_Velocity_and_Angular_Velocity
- ↑ https://physics.info/velocity/
About This Article

Velocity is defined as the speed at which an object travels in a given direction. The right formula to use for calculating velocity depends on a few different factors, such as whether the object is accelerating at a constant rate, or whether it is moving in a circle as opposed to a line. The most basic formula for calculating velocity is velocity (v) = distance (d)/time (t). If you don’t already know the time and distance, you’ll need to calculate them first. Subtract the initial position from the final position to find distance, and subtract the start time from the end time to find the time. For instance, if a runner began sprinting due east at the 22-meter mark along a track and ended up at the 52-meter mark, you’d subtract 22 from 52 to find the distance, or displacement, of 30 meters. Similarly, if they began their sprint at 5:35:01 pm and ended it at 5:35:06 pm, you can find the time by subtracting 1 from 6, giving you 5 seconds. This will tell you that they ran 30 meters in 5 seconds, which means that they maintained an average velocity of 6 m/s east. If you’re finding the velocity of an object that’s accelerating instead of moving at a constant rate, things get a little more complicated. If you know the acceleration rate of the object, you can find the final velocity using the formula vf (final velocity) = vi (initial velocity) + a(t) (acceleration x time). For example, if an object accelerated north at a rate of 5m/s2 over 5 seconds and had a starting velocity of 6 m/s, its final velocity would be 6m/s + (5m/s2 x 5s), or 31m/s north. Once you know both the final and initial velocity, you can calculate the average velocity of an accelerating object. To do this, add initial velocity to final velocity and divide the result by 2. In this case, 6m/s + 30m/s divided by 2 = 18 m/s north. The method for finding the velocity of an object around a circle is a little different. To do this, use the formula v (velocity) = 2πr (the circumference of the circle)/t (time). For example, an object that moves around a circle with a radius of 50 meters in 13 seconds would have a velocity of 2π(50)m/13s, or approximately 24.17 m/s. To learn more, such as how to calculate average or circular velocity, keep reading the article! Did this summary help you? Yes No
- Send fan mail to authors
Reader Success Stories

Tommie Depew
May 27, 2017
Did this article help you?

Nkateko Maluleke
Nov 9, 2017

Nicholas Kingsley
Nov 12, 2016

Dec 5, 2016
Oct 27, 2017

Featured Articles

Trending Articles

Watch Articles

- Terms of Use
- Privacy Policy
- Do Not Sell or Share My Info
- Not Selling Info
Don’t miss out! Sign up for
wikiHow’s newsletter
Home of Science
- WAVES/ACOUSTICS
- THERMODYNAMICS
- ELECTROMAGNETISM
- SOLAR ENERGY
- MODERN PHYSICS
- QUESTIONS AND ANSWERS
- Privacy Policy
- Terms and Conditions
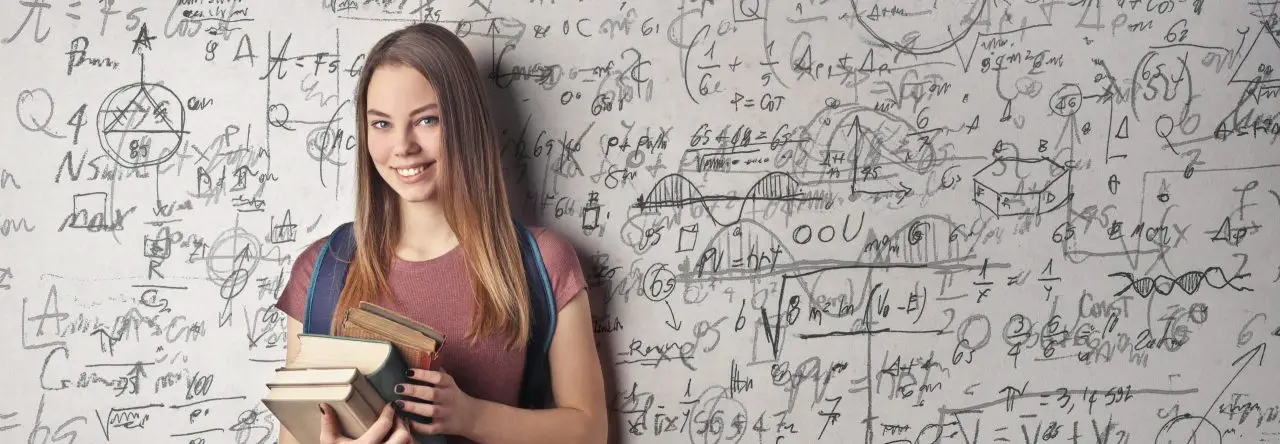
Speed, Velocity, and Acceleration Problems
By Abnurlion
On December 26, 2022
In MECHANICS
Table of Contents

What is Speed?
Definition of Speed : Speed is the rate of change of distance with time . Speed is different from velocity because it’s not in a specified direction. In this article, you will learn how to solve speed, velocity, and acceleration problems.
Additionally, you need to know that speed is a scalar quantity and we can write its symbol as S. The formula for calculating the speed of an object is:
Speed, S = Distance (d) / Time (t)
Thus, s = d/t
Note: In most cases, we also use S as a symbol for distance.
The S.I unit for speed is meter per second (m/s) or ms -1
Non-Uniform or Average Speed : This is a non-steady distance covered by an object at a particular period of time. We can also define non-uniform speed as the type of distance that an object covered at an equal interval of time.
The formula for calculating non-uniform speed is
Average speed = Total distance covered by the object / Total time taken
Actual speed: This is also known as the instantaneous speed of an object which is the distance covered by an object over a short interval of time.
You may also like to read:
How to Find Displacement in Physics
also How to Find a Position in Physics
and How to Calculate Bearing in Physics
What is Velocity?
Definition of Velocity: Velocity is the rate of displacement with time. Velocity is the speed of an object in a specified direction. The unit of velocity is the same as that of speed which is meter per second (ms -1 ). We use V as a symbol for velocity.
Note: We often use U to indicate initial speed, and V to indicate final speed.
The formula for calculating Velocity (V) = displacement (S) / time (t)
The difference between velocity and speed is the presence of displacement and distance respectively. Because displacement is a measure of separation in a specified direction, while distance is not in a specified direction. Velocity is a vector quantity.
Uniform Velocity
Definition of uniform velocity: The rate of change of displacement is constant no matter how small the time interval may be. Also, uniform velocity is the distance covered by an object in a specified direction in an equal time interval.
The formula for uniform velocity = Total displacement / Total time taken
What is Acceleration?
Definition of Acceleration: Acceleration is the rate of change of velocity with time. Acceleration is measured in meters per second square (ms -2 ). The symbol for acceleration is a. Acceleration is also a vector quantity.
The formula for acceleration , a = change in velocity (v)/time taken (t)
Thus, a = v/t
We can also write acceleration as
a = change in velocity/time = ΔV/Δt = (v – u)/t
[where v = final velocity, u = initial velocity, and t = time taken]
Uniform Acceleration
In the case of uniform acceleration , the rate of change of velocity with time is constant.
Average velocity of the object = (Initial velocity + final velocity)/2
Average velocity = (v + u)/2
What is Retardation?
Retardation is the decreasing rate of change in velocity moved, covered, or traveled by an object.
The formula for calculating retardation is
Retardation = Change in a decrease in velocity/time taken
Equations of Motion
You can also apply the following equations of motion to calculate the speed, velocity, and acceleration of the body:
- s = [ (v + u)/2 ]t
- v 2 = u 2 + 2as
s = ut + (1/2)at 2
Where v = final velocity, u = initial velocity, t = time, a = acceleration, and s = distance
How to Calculate Maximum height
What are Prefixes in Physics
How to Calculate Dimension in Physics
Solved Problems of Speed, Velocity, and Acceleration
Here are solved problems to help you understand how to calculate speed, velocity, and acceleration:
A train moves at a speed of 54km/h for a one-quarter minute. Find the distance travelled by train.
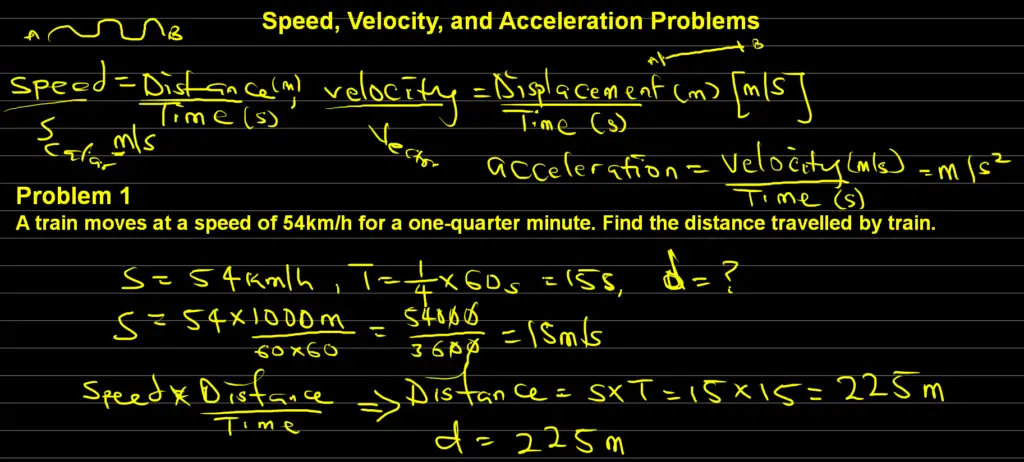
From the question above
Speed = 54 km/h = (54 x 1000)/(60 x 60) = 54,000/3,600 = 15 m/s
Time = one quarter minute = 1/4 minute = (1/4) x 60 = 15 seconds
Since we have
speed = distance/time
After cross-multiplication, we will now have
Distance = speed x time
We can now insert our data into the above expression
Distance = 15 m/s x 15 s = 225 m
Therefore, the distance travelled by train is 225 meters.
A car travelled a distance of 5km in 50 seconds. Find the speed in meters per second.
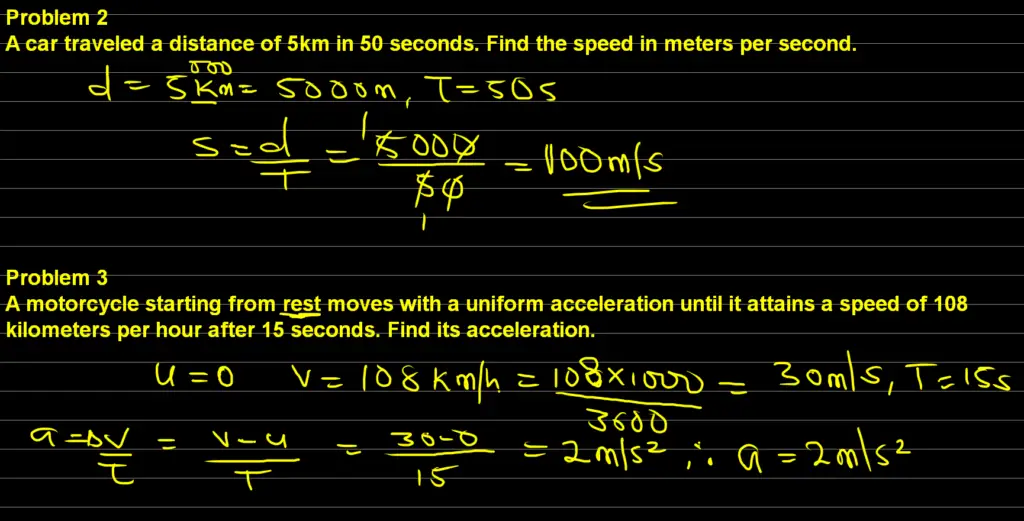
Distance = 5km = 5 x 1000m = 5,000m
Time = 50 seconds
and the formula for speed
speed = distance/time = 5000/50 = 100m/s
A motorcycle starting from rest moves with a uniform acceleration until it attains a speed of 108 kilometres per hour after 15 seconds. Find its acceleration.
Initial velocity, u = 0 (because the motorcycle starts from rest)
Final velocity, v = speed = 108 km/h = (108 x 1000m) / (60 x 60s) = 108,000/3,600 = 30m/s
Time taken, t = 15 seconds
Therefore, we can now apply the formula that says
Acceleration = change in velocity/ time = (v-u)/t = (30-0)/15 =30/15 = 2ms -2
Therefore, the acceleration of the motorcycle is 2ms -2
A bus covers 50 kilometres in 1 hour. What is it is the average speed?
Total distance covered = 50 km = 50 x 1000m = 50,000m
Time taken = 1 hour = 1 x 60 x 60s =3,600s
Therefore, we can now calculate the average speed of the bus by substituting our data into the above formula
Average speed = 50,000/3,600 = 13.9 m/s
Therefore, the average speed of the bus is 13.9m/s or approximately 14 meters per second.
A car travels 80 km in 1 hour and then another 20 km in the next hour. Find the average velocity of the car.
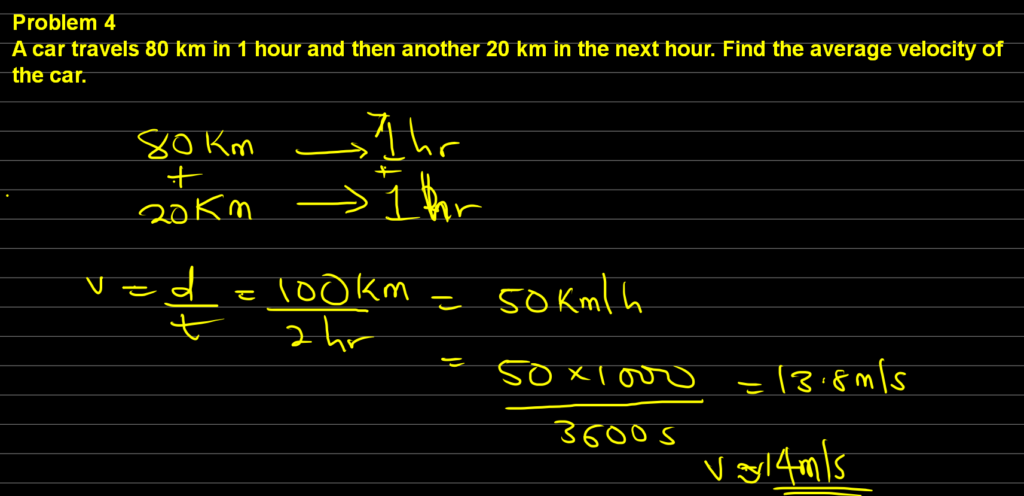
Initial displacement of the car = 80km
Final displacement of the car = 20km
The total displacement of the car = initial displacement of the car + final displacement of the car
The total displacement of the car = 80km + 20km = 100km
The time for 80km is 1hr
And the time for 20km is 1hr
Total time taken = The time for 80km (1hr) + The time for 20km (1hr)
Total time taken = 1hr + 1hr = 2hrs
Now, to calculate the average velocity of the car, we apply the formula that says
Average velocity = total displacement/total time taken = 100km/2hrs = 50km/h
We can further convert the above answer into meters per second
Average velocity = 50km/h = (50 x 1000m)/(1 x 60 x 60s) = 50,000/3,600 = 13.9m/s or 14ms -1
Therefore, the average velocity of the car is 14ms -1
How to Conduct Physics Practical
and How to Calculate Velocity Ratio of an Inclined Plane
A body falls from the top of a tower 100 meters high and hits the ground in 5 seconds. Find its acceleration.
Displacement = 100m
Time = 5 seconds
and we can apply the formula for acceleration that says
acceleration, a = Displacement/time 2 = 100/5 2 = 100/25 = 4ms -2
Therefore the acceleration due to the gravity of the body is 4ms -2
Note: The acceleration due to the gravity of a body on the surface of the earth is constant (9.8ms -2 ), even though there may be a slight difference due to the mass and altitude of the body.
An object is thrown vertically upward at an initial velocity of 10ms -1 and reaches a maximum height of 50 meters. Find its initial upward acceleration.
Initial velocity, u = 10ms -1
Final velocity, v = 0
maximum height = displacement = 50m
Initial upward acceleration, a =?
When we apply the formula that says a = (v 2 – u 2 )/2d we will have
a = (0 – 10 2 )/(2 x 50) = -100/100 = -1 ms -2
Hence, since our acceleration is negative, we can now say that we are dealing with retardation or deceleration.
Therefore, the retardation is -1ms -2
Note: Retardation is the negative of acceleration, thus it is written in negative form.
A car is traveling at a velocity of 8ms -1 and experiences an acceleration of 5ms -2 . How far does it travel in 4 seconds?
Initial velocity, u = 8ms -1
acceleration, a = 5ms -2
Distance, s =?
time, t = 4s
We can apply the formula that says
s = 8 x 4 + (1/2) x 5 x 4 2
s = 32 + (1/2) x 80 = 32 + 40 = 72m
Therefore, the distance covered by the car in 4s is 72 meters.
A body is traveling at a velocity of 10m/s and experiences a deceleration of 5ms -2 . How long does it take the body to come to a complete stop?
Initial velocity, u = 10m/s
acceleration , a = retardation = -5ms -2
time, t = ?
We already know that acceleration, a = change in velocity/time
This implies that
Time, t = change in velocity/acceleration
t = (v – u)/t = (0-10)/-5 = -10/-5 = 2s
Therefore, the time it takes the car to stop is 2 seconds .
A body is traveling at a velocity of 20 m/s and has a mass of 10 kg. How much force is required to change its velocity by 10 m/s in 5 seconds?
Change in velocity, v =10 m/s
mass of the object, m = 10kg
time, t = 5s
We can apply newton’s second law of motion which says f = ma
and since a = change in velocity/time
we will have an acceleration equal to
a = 10/5 = 2ms -2
Therefore, to find the force, we can now say
f = ma = 10 x 2 = 20N
Therefore, the force that can help us to change the velocity by 10 m/s in 5 seconds is 20-Newton.
Drop a comment if there is anything you don’t understand about speed, velocity, and acceleration Problems.
How to Calculate Cost of Electricity Per kwh
also How to Calculate the Efficiency of a Transformer
and How to Calculate Escape Velocity of a Satellite
Check our Website:
Wokminer – Apply for jobs
Share this post: on Twitter on Facebook on Google+
How to Calculate Centripetal Force
Powered by WordPress & Theme by Anders Norén

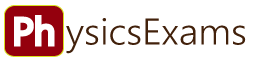
- Exam Center
- Ticket Center
- Flash Cards
- Straight Line Motion
Solved Speed, Velocity, and Acceleration Problems
Simple problems on speed, velocity, and acceleration with descriptive answers are presented for the AP Physics 1 exam and college students. In each solution, you can find a brief tutorial.
Speed and velocity Problems:
Problem (1): What is the speed of a rocket that travels $8000\,{\rm m}$ in $13\,{\rm s}$?
Solution : Speed is defined in physics as the total distance divided by the elapsed time, so the rocket's speed is \[\text{speed}=\frac{8000}{13}=615.38\,{\rm m/s}\]
Problem (2): How long will it take if you travel $400\,{\rm km}$ with an average speed of $100\,{\rm m/s}$?
Solution : Average speed is the ratio of the total distance to the total time. Thus, the elapsed time is \begin{align*} t&=\frac{\text{total distance}}{\text{average speed}}\\ \\ &=\frac{400\times 10^{3}\,{\rm m}}{100\,{\rm m/s}}\\ \\ &=4000\,{\rm s}\end{align*} To convert it to hours, it must be divided by $3600\,{\rm s}$ which gives $t=1.11\,{\rm h}$.
Problem (3): A person walks $100\,{\rm m}$ in $5$ minutes, then $200\,{\rm m}$ in $7$ minutes, and finally $50\,{\rm m}$ in $4$ minutes. Find its average speed.
Solution : First find its total distance traveled ($D$) by summing all distances in each section, which gets $D=100+200+50=350\,{\rm m}$. Now, by definition of average speed, divide it by the total time elapsed $T=5+7+4=16$ minutes.
But keep in mind that since the distance is in SI units, so the time traveled must also be in SI units, which is $\rm s$. Therefore, we have\begin{align*}\text{average speed}&=\frac{\text{total distance} }{\text{total time} }\\ \\ &=\frac{350\,{\rm m}}{16\times 60\,{\rm s}}\\ \\&=0.36\,{\rm m/s}\end{align*}
Problem (4): A person walks $750\,{\rm m}$ due north, then $250\,{\rm m}$ due east. If the entire walk takes $12$ minutes, find the person's average velocity.
Solution : Average velocity , $\bar{v}=\frac{\Delta x}{\Delta t}$, is displacement divided by the elapsed time. Displacement is also a vector that obeys the addition vector rules. Thus, in this velocity problem, add each displacement to get the total displacement .
In the first part, displacement is $\Delta x_1=750\,\hat{j}$ (due north) and in the second part $\Delta x_2=250\,\hat{i}$ (due east). The total displacement vector is $\Delta x=\Delta x_1+\Delta x_2=750\,\hat{i}+250\,\hat{j}$ with magnitude of \begin{align*}|\Delta x|&=\sqrt{(750)^{2}+(250)^{2}}\\ \\&=790.5\,{\rm m}\end{align*} In addition, the total elapsed time is $t=12\times 60$ seconds. Therefore, the magnitude of the average velocity is \[\bar{v}=\frac{790.5}{12\times 60}=1.09\,{\rm m/s}\]
Problem (5): An object moves along a straight line. First, it travels at a velocity of $12\,{\rm m/s}$ for $5\,{\rm s}$ and then continues in the same direction with $20\,{\rm m/s}$ for $3\,{\rm s}$. What is its average speed?
Solution: Average velocity is displacement divided by elapsed time, i.e., $\bar{v}\equiv \frac{\Delta x_{tot}}{\Delta t_{tot}}$.
Here, the object goes through two stages with two different displacements, so add them to find the total displacement. Thus,\[\bar{v}=\frac{x_1 + x_2}{t_1 +t_2}\] Again, to find the displacement, we use the same equation as the average velocity formula, i.e., $x=vt$. Thus, displacements are obtained as $x_1=v_1\,t_1=12\times 5=60\,{\rm m}$ and $x_2=v_2\,t_2=20\times 3=60\,{\rm m}$. Therefore, we have \begin{align*} \bar{v}&=\frac{x_1+x_2}{t_1+t_2}\\ \\&=\frac{60+60}{5+3}\\ \\&=\boxed{15\,{\rm m/s}}\end{align*}
Problem (6): A plane flies the distance between two cities in $1$ hour and $30$ minutes with a velocity of $900\,{\rm km/h}$. Another plane covers that distance at $600\,{\rm km/h}$. What is the flight time of the second plane?
Solution: first find the distance between two cities using the average velocity formula $\bar{v}=\frac{\Delta x}{\Delta t}$ as below \begin{align*} x&=vt\\&=900\times 1.5\\&=1350\,{\rm km}\end{align*} where we wrote one hour and a half minutes as $1.5\,\rm h$. Now use again the same kinematic equation above to find the time required for another plane \begin{align*} t&=\frac xv\\ \\ &=\frac{1350\,\rm km}{600\,\rm km/h}\\ \\&=2.25\,{\rm h}\end{align*} Thus, the time for the second plane is $2$ hours and $0.25$ of an hour, which converts to minutes as $2$ hours and ($0.25\times 60=15$) minutes.
Problem (7): To reach a park located south of his jogging path, Henry runs along a 15-kilometer route. If he completes the journey in 1.5 hours, determine his speed and velocity.
Solution: Henry travels his route to the park without changing direction along a straight line. Therefore, the total distance traveled in one direction equals the displacement, i.e, \[\text{distance traveled}=\Delta x=15\,\rm km\]Velocity is displacement divided by the time of travel \begin{align*} \text{velocity}&=\frac{\text{displacement}}{\text{time of travel}} \\\\ &=\frac{15\,\rm km}{1.5\,\rm h} \\\\ &=\boxed{10\,\rm km/h}\end{align*} and by definition, its average speed is \begin{align*} \text{speed}&=\frac{\text{distance covered}}{\text{time interval}}\\\\&=\frac{15\,\rm km}{1.5\,\rm h}\\\\&=\boxed{10\,\rm km/h}\end{align*} Thus, Henry's velocity is $10\,\rm km/h$ to the south, and its speed is $10\,\rm km/h$. As you can see, speed is simply a positive number, with units but velocity specifies the direction in which the object is moving.
Problem (8): In 15 seconds, a football player covers the distance from his team's goal line to the opposing team's goal line and back to the midway point of the field having 100-yard-length. Find, (a) his average speed, and (b) the magnitude of the average velocity.
Solution: The total length of the football field is $100$ yards or in meters, $L=91.44\,\rm m$. Going from one goal's line to the other and back to the midpoint of the field takes $15\,\rm s$ and covers a distance of $D=100+50=150\,\rm yd$.
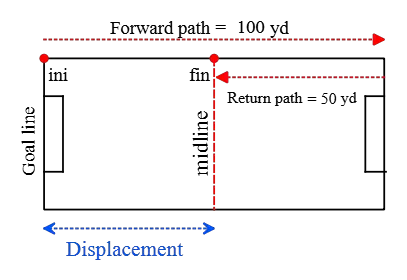
Distance divided by the time of travel gets the average speed, \[\text{speed}=\frac{150\times 0.91}{15}=9.1\,\rm m/s\] To find the average velocity, we must find the displacement of the player between the initial and final points.
The initial point is her own goal line and her final position is the midpoint of the field, so she has displaced a distance of $\Delta x=50\,\rm yd$ or $\Delta x=50\times 0.91=45.5\,\rm m$. Therefore, her velocity is calculated as follows \begin{align*} \text{velocity}&=\frac{\text{displacement}}{\text{time elapsed}} \\\\ &=\frac{45.5\,\rm m}{15\,\rm s} \\\\&=\boxed{3.03\quad \rm m/s}\end{align*} Contrary to the previous problem, here the motion is not in one direction, hence, the displacement is not equal to the distance traveled. Accordingly, the average speed is not equal to the magnitude of the average velocity.
Problem (9): You begin at a pillar and run towards the east (the positive $x$ direction) for $250\,\rm m$ at an average speed of $5\,\rm m/s$. After that, you run towards the west for $300\,\rm m$ at an average speed of $4\,\rm m/s$ until you reach a post. Calculate (a) your average speed from pillar to post, and (b) your average velocity from pillar to post.
Solution : First, you traveled a distance of $L_1=250\,\rm m$ toward east (or $+x$ direction) at $5\,\rm m/s$. Time of travel in this route is obtained as follows \begin{align*} t_1&=\frac{L_1}{v_1}\\\\ &=\frac{250}{5}\\\\&=50\,\rm s\end{align*} Likewise, traveling a distance of $L_2=300\,\rm m$ at $v_2=4\,\rm m/s$ takes \[t_2=\frac{300}{4}=75\,\rm s\] (a) Average speed is defined as the distance traveled (or path length) divided by the total time of travel \begin{align*} v&=\frac{\text{path length}}{\text{time of travel}} \\\\ &=\frac{L_1+L_2}{t_1+t_2}\\\\&=\frac{250+300}{50+75} \\\\&=4.4\,\rm m/s\end{align*} Therefore, you travel between these two pillars in $125\,\rm s$ and with an average speed of $4.4\,\rm m/s$.
(b) Average velocity requires finding the displacement between those two points. In the first case, you move $250\,\rm m$ toward $+x$ direction, i.e., $L_1=+250\,\rm m$. Similarly, on the way back, you move $300\,\rm m$ toward the west ($-x$ direction) or $L_2=-300\,\rm m$. Adding these two gives us the total displacement between the initial point and the final point, \begin{align*} L&=L_1+L_2 \\\\&=(+250)+(-300) \\\\ &=-50\,\rm m\end{align*} The minus sign indicates that you are generally displaced toward the west.
Finally, the average velocity is obtained as follows: \begin{align*} \text{average velocity}&=\frac{\text{displacement}}{\text{time of travel}} \\\\ &=\frac{-50}{125} \\\\&=-0.4\,\rm m/s\end{align*} A negative average velocity indicating motion to the left along the $x$-axis.
This speed problem better makes it clear to us the difference between average speed and average speed. Unlike average speed, which is always a positive number, the average velocity in a straight line can be either positive or negative.
Problem (10): What is the average speed for the round trip of a car moving uphill at 40 km/h and then back downhill at 60 km/h?
Solution : Assuming the length of the hill to be $L$, the total distance traveled during this round trip is $2L$ since $L_{up}=L_{down}=L$. However, the time taken for going uphill and downhill was not provided. We can write them in terms of the hill's length $L$ as $t=\frac L v$.
Applying the definition of average speed gives us \begin{align*} v&=\frac{\text{distance traveled}}{\text{total time}} \\\\ &=\frac{L_{up}+L_{down}}{t_{up}+t_{down}} \\\\ &=\cfrac{2L}{\cfrac{L}{v_{up}}+\cfrac{L}{v_{down}}} \end{align*} By reorganizing this expression, we obtain a formula that is useful for solving similar problems in the AP Physics 1 exams. \[\text{average speed}=\frac{2v_{up} \times v_{down}}{v_{up}+v_{down}}\] Substituting the numerical values into this, yields \begin{align*} v&=\frac{2(40\times 60)}{40+60} \\\\ &=\boxed{48\,\rm m/s}\end{align*} What if we were asked for the average velocity instead? During this round trip, the car returns to its original position, and thus its displacement, which defines the average velocity, is zero. Therefore, \[\text{average velocity}=0\,\rm m/s\]
Acceleration Problems
Problem (9): A car moves from rest to a speed of $45\,\rm m/s$ in a time interval of $15\,\rm s$. At what rate does the car accelerate?
Solution : The car is initially at rest, $v_1=0$, and finally reaches $v_2=45\,\rm m/s$ in a time interval $\Delta t=15\,\rm s$. Average acceleration is the change in velocity, $\Delta v=v_2-v_1$, divided by the elapsed time $\Delta t$, so \[\bar{a}=\frac{45-0}{15}=\boxed{3\,\rm m/s^2} \]
Problem (10): A car moving at a velocity of $15\,{\rm m/s}$, uniformly slows down. It comes to a complete stop in $10\,{\rm s}$. What is its acceleration?
Solution: Let the car's uniform velocity be $v_1$ and its final velocity $v_2=0$. Average acceleration is the difference in velocities divided by the time taken, so we have: \begin{align*}\bar{a}&=\frac{\Delta v}{\Delta t}\\\\&=\frac{v_2-v_1}{\Delta t}\\\\&=\frac{0-15}{10}\\\\ &=\boxed{-1.5\,{\rm m/s^2}}\end{align*}The minus sign indicates the direction of the acceleration vector, which is toward the $-x$ direction.
Problem (11): A car moves from rest to a speed of $72\,{\rm km/h}$ in $4\,{\rm s}$. Find the acceleration of the car.
Solution: Known: $v_1=0$, $v_2=72\,{\rm km/h}$, $\Delta t=4\,{\rm s}$. Average acceleration is defined as the difference in velocities divided by the time interval between those points \begin{align*}\bar{a}&=\frac{v_2-v_1}{t_2-t_1}\\\\&=\frac{20-0}{4}\\\\&=5\,{\rm m/s^2}\end{align*} In above, we converted $\rm km/h$ to the SI unit of velocity ($\rm m/s$) as \[1\,\frac{km}{h}=\frac {1000\,m}{3600\,s}=\frac{10}{36}\, \rm m/s\] so we get \[72\,\rm km/h=72\times \frac{10}{36}=20\,\rm m/s\]
Problem (12): A race car accelerates from an initial velocity of $v_i=10\,{\rm m/s}$ to a final velocity of $v_f = 30\,{\rm m/s}$ in a time interval of $2\,{\rm s}$. Determine its average acceleration.
Solution: A change in the velocity of an object $\Delta v$ over a time interval $\Delta t$ is defined as an average acceleration. Known: $v_i=10\,{\rm m/s}$, $v_f = 30\,{\rm m/s}$, $\Delta t=2\,{\rm s}$. Applying definition of average acceleration, we get \begin{align*}\bar{a}&=\frac{v_f-v_i}{\Delta t}\\&=\frac{30-10}{2}\\&=10\,{\rm m/s^2}\end{align*}
Problem (13): A motorcycle starts its trip along a straight line with a velocity of $10\,{\rm m/s}$ and ends with $20\,{\rm m/s}$ in the opposite direction in a time interval of $2\,{\rm s}$. What is the average acceleration of the car?
Solution: Known: $v_i=10\,{\rm m/s}$, $v_f=-20\,{\rm m/s}$, $\Delta t=2\,{\rm s}$, $\bar{a}=?$. Using average acceleration definition we have \begin{align*}\bar{a}&=\frac{v_f-v_i}{\Delta t}\\\\&=\frac{(-20)-10}{2}\\\\ &=\boxed{-15\,{\rm m/s^2}}\end{align*}Recall that in the definition above, velocities are vector quantities. The final velocity is in the opposite direction from the initial velocity so a negative must be included.
Problem (14): A ball is thrown vertically up into the air by a boy. After $4$ seconds, it reaches the highest point of its path. How fast does the ball leave the boy's hand?
Solution : At the highest point, the ball has zero speed, $v_2=0$. It takes the ball $4\,\rm s$ to reach that point. In this problem, our unknown is the initial speed of the ball, $v_1=?$. Here, the ball accelerates at a constant rate of $g=-9.8\,\rm m/s^2$ in the presence of gravity.
When the ball is tossed upward, the only external force that acts on it is the gravity force.
Using the average acceleration formula $\bar{a}=\frac{\Delta v}{\Delta t}$ and substituting the numerical values into this, we will have \begin{gather*} \bar{a}=\frac{\Delta v}{\Delta t} \\\\ -9.8=\frac{0-v_1}{4} \\\\ \Rightarrow \boxed{v_1=39.2\,\rm m/s} \end{gather*} Note that $\Delta v=v_2-v_1$.
Problem (15): A child drops crumpled paper from a window. The paper hit the ground in $3\,\rm s$. What is the velocity of the crumpled paper just before it strikes the ground?
Solution : The crumpled paper is initially in the child's hand, so $v_1=0$. Let its speed just before striking be $v_2$. In this case, we have an object accelerating down in the presence of gravitational force at a constant rate of $g=-9.8\,\rm m/s^2$. Using the definition of average acceleration, we can find $v_2$ as below \begin{gather*} \bar{a}=\frac{\Delta v}{\Delta t} \\\\ -9.8=\frac{v_2-0}{3} \\\\ \Rightarrow v_2=3\times (-9.8)=\boxed{-29.4\,\rm m/s} \end{gather*} The negative shows us that the velocity must be downward, as expected!
Problem (16): A car travels along the $x$-axis for $4\,{\rm s}$ at an average velocity of $10\,{\rm m/s}$ and $2\,{\rm s}$ with an average velocity of $30\,{\rm m/s}$ and finally $4\,{\rm s}$ with an average velocity $25\,{\rm m/s}$. What is its average velocity across the whole path?
Solution: There are three different parts with different average velocities. Assume each trip is done in one dimension without changing direction. Thus, displacements associated with each segment are the same as the distance traveled in that direction and is calculated as below: \begin{align*}\Delta x_1&=v_1\,\Delta t_1\\&=10\times 4=40\,{\rm m}\\ \\ \Delta x_2&=v_2\,\Delta t_2\\&=30\times 2=60\,{\rm m}\\ \\ \Delta x_3&=v_3\,\Delta t_3\\&=25\times 4=100\,{\rm m}\end{align*}Now use the definition of average velocity, $\bar{v}=\frac{\Delta x_{tot}}{\Delta t_{tot}}$, to find it over the whole path\begin{align*}\bar{v}&=\frac{\Delta x_{tot}}{\Delta t_{tot}}\\ \\&=\frac{\Delta x_1+\Delta x_2+\Delta x_3}{\Delta t_1+\Delta t_2+\Delta t_3}\\ \\&=\frac{40+60+100}{4+2+4}\\ \\ &=\boxed{20\,{\rm m/s}}\end{align*}
Problem (17): An object moving along a straight-line path. It travels with an average velocity $2\,{\rm m/s}$ for $20\,{\rm s}$ and $12\,{\rm m/s}$ for $t$ seconds. If the total average velocity across the whole path is $10\,{\rm m/s}$, then find the unknown time $t$.
Solution: In this velocity problem, the whole path $\Delta x$ is divided into two parts $\Delta x_1$ and $\Delta x_2$ with different average velocities and times elapsed, so the total average velocity across the whole path is obtained as \begin{align*}\bar{v}&=\frac{\Delta x}{\Delta t}\\\\&=\frac{\Delta x_1+\Delta x_2}{\Delta t_1+\Delta t_2}\\\\&=\frac{\bar{v}_1\,t_1+\bar{v}_2\,t_2}{t_1+t_2}\\\\10&=\frac{2\times 20+12\times t}{20+t}\\\Rightarrow t&=80\,{\rm s}\end{align*}
Note : whenever a moving object, covers distances $x_1,x_2,x_3,\cdots$ in $t_1,t_2,t_3,\cdots$ with constant or average velocities $v_1,v_2,v_3,\cdots$ along a straight-line without changing its direction, then its total average velocity across the whole path is obtained by one of the following formulas
- Distances and times are known:\[\bar{v}=\frac{x_1+x_2+x_3+\cdots}{t_1+t_2+t_3+\cdots}\]
- Velocities and times are known: \[\bar{v}=\frac{v_1\,t_1+v_2\,t_2+v_3\,t_3+\cdots}{t_1+t_2+t_3+\cdots}\]
- Distances and velocities are known:\[\bar{v}=\frac{x_1+x_2+x_3+\cdots}{\frac{x_1}{v_1}+\frac{x_2}{v_2}+\frac{x_3}{v_3}+\cdots}\]
Problem (18): A car travels one-fourth of its path with a constant velocity of $10\,{\rm m/s}$, and the remaining with a constant velocity of $v_2$. If the total average velocity across the whole path is $16\,{\rm m/s}$, then find the $v_2$?
Solution: This is the third case of the preceding note. Let the length of the path be $L$ so \begin{align*}\bar{v}&=\frac{x_1+x_2}{\frac{x_1}{v_1}+\frac{x_2}{v_2}}\\\\16&=\frac{\frac 14\,L+\frac 34\,L}{\frac{\frac 14\,L}{10}+\frac{\frac 34\,L}{v_2}}\\\\\Rightarrow v_2&=20\,{\rm m/s}\end{align*}
Problem (19): An object moves along a straight-line path. It travels for $t_1$ seconds with an average velocity $50\,{\rm m/s}$ and $t_2$ seconds with a constant velocity of $25\,{\rm m/s}$. If the total average velocity across the whole path is $30\,{\rm m/s}$, then find the ratio $\frac{t_2}{t_1}$?
Solution: the velocities and times are known, so we have \begin{align*}\bar{v}&=\frac{v_1\,t_1+v_2\,t_2}{t_1+t_2}\\\\30&=\frac{50\,t_1+25\,t_2}{t_1+t_2}\\\\ \Rightarrow \frac{t_2}{t_1}&=4\end{align*}
Read more related articles:
Kinematics Equations: Problems and Solutions
Position vs. Time Graphs
Velocity vs. Time Graphs
In the following section, some sample AP Physics 1 problems on acceleration are provided.
Problem (20): An object moves with constant acceleration along a straight line. If its velocity at instant of $t_1 = 3\,{\rm s}$ is $10\,{\rm m/s}$ and at the moment of $t_2 = 8\,{\rm s}$ is $20\,{\rm m/s}$, then what is its initial speed?
Solution: Let the initial speed at time $t=0$ be $v_0$. Now apply average acceleration definition in the time intervals $[t_0,t_1]$ and $[t_0,t_2]$ and equate them.\begin{align*}\text{average acceleration}\ \bar{a}&=\frac{\Delta v}{\Delta t}\\\\\frac{v_1 - v_0}{t_1-t_0}&=\frac{v_2-v_0}{t_2-t_0}\\\\ \frac{10-v_0}{3-0}&=\frac{20-v_0}{8-0}\\\\ \Rightarrow v_0 &=4\,{\rm m/s}\end{align*} In the above, $v_1$ and $v_2$ are the velocities at moments $t_1$ and $t_2$, respectively.
Problem (21): For $10\,{\rm s}$, the velocity of a car that travels with a constant acceleration, changes from $10\,{\rm m/s}$ to $30\,{\rm m/s}$. How far does the car travel?
Solution: Known: $\Delta t=10\,{\rm s}$, $v_1=10\,{\rm m/s}$ and $v_2=30\,{\rm m/s}$.
Method (I) Without computing the acceleration: Recall that in the case of constant acceleration, we have the following kinematic equations for average velocity and displacement:\begin{align*}\text{average velocity}:\,\bar{v}&=\frac{v_1+v_2}{2}\\\text{displacement}:\,\Delta x&=\frac{v_1+v_2}{2}\times \Delta t\\\end{align*}where $v_1$ and $v_2$ are the velocities in a given time interval. Now we have \begin{align*} \Delta x&=\frac{v_1+v_2}{2}\\&=\frac{10+30}{2}\times 10\\&=200\,{\rm m}\end{align*}
Method (II) with computing acceleration: Using the definition of average acceleration, first determine it as below \begin{align*}\bar{a}&=\frac{\Delta v}{\Delta t}\\\\&=\frac{30-10}{10}\\\\&=2\,{\rm m/s^2}\end{align*} Since the velocities at the initial and final points of the problem are given so use the below time-independent kinematic equation to find the required displacement \begin{align*} v_2^{2}-v_1^{2}&=2\,a\Delta x\\\\ (30)^{2}-(10)^{2}&=2(2)\,\Delta x\\\\ \Rightarrow \Delta x&=\boxed{200\,{\rm m}}\end{align*}
Problem (22): A car travels along a straight line with uniform acceleration. If its velocity at the instant of $t_1=2\,{\rm s}$ is $36\,{\rm km/s}$ and at the moment $t_2=6\,{\rm s}$ is $72\,{\rm km/h}$, then find its initial velocity (at $t_0=0$)?
Solution: Use the equality of definition of average acceleration $a=\frac{v_f-v_i}{t_f-t_i}$ in the time intervals $[t_0,t_1]$ and $[t_0,t_2]$ to find the initial velocity as below \begin{align*}\frac{v_2-v_0}{t_2-t_0}&=\frac{v_1-v_0}{t_1-t_0}\\\\ \frac{20-v_0}{6-0}&=\frac{10-v_0}{2-0}\\\\ \Rightarrow v_0&=\boxed{5\,{\rm m/s}}\end{align*}
All these kinematic problems on speed, velocity, and acceleration are easily solved by choosing an appropriate kinematic equation. Keep in mind that these motion problems in one dimension are of the uniform or constant acceleration type. Projectiles are also another type of motion in two dimensions with constant acceleration.
Author: Dr. Ali Nemati
Date Published: 9/6/2020
Updated: Jun 28, 2023
© 2015 All rights reserved. by Physexams.com
HIGH SCHOOL
- ACT Tutoring
- SAT Tutoring
- PSAT Tutoring
- ASPIRE Tutoring
- SHSAT Tutoring
- STAAR Tutoring
GRADUATE SCHOOL
- MCAT Tutoring
- GRE Tutoring
- LSAT Tutoring
- GMAT Tutoring
- AIMS Tutoring
- HSPT Tutoring
- ISAT Tutoring
- SSAT Tutoring
Search 50+ Tests
Loading Page
math tutoring
- Elementary Math
- Pre-Calculus
- Trigonometry
science tutoring
Foreign languages.
- Mandarin Chinese
elementary tutoring
- Computer Science
Search 350+ Subjects
- Video Overview
- Tutor Selection Process
- Online Tutoring
- Mobile Tutoring
- Instant Tutoring
- How We Operate
- Our Guarantee
- Impact of Tutoring
- Reviews & Testimonials
- Media Coverage
- About Varsity Tutors
Precalculus : Solve Linear Velocity Problems
Study concepts, example questions & explanations for precalculus, all precalculus resources, example questions, example question #1 : solve linear velocity problems.
None of the other answers
Since the plane is traveling NE, we know that it is traveling at an angle of 45 degrees north of east. Therefore, we can represent this in a diagram:
We have all of the information we need to solve for the velocity of the plane. We have an angle and a side adjacent to that angle. Therefore, we can use the cosine function to solve for the velocity.
Rearranging for v, we get:
Example Question #1 : Angular And Linear Velocity
Example Question #3 : Angular And Linear Velocity
An object is moving in a circular path. How will its linear velocity change if the diameter of the circular path was decreased by one half?
Write the formula for the linear velocity.
The diameter is twice the radius.
If the diameter was decreased by one half, the radius will also decrease by one half.
The linear velocity will decrease by one half.
Write the linear velocity formula.
Since the answer is required in meters per second, two conversions will need to be made.
Convert 30 kilometers to meters.
Convert 2 hours to seconds.
Apply the formula.
Example Question #5 : Angular And Linear Velocity
None of the other answers.
And how long does it take for the hand to go around? Linear speed of the clock second hand is
A clock has a second hand measuring 12cm. What is the linear speed of the tip of the second hand?
None of these/
Linear speed is equal to the arc length traversed divided by the time. We use s to denote arc length.
Report an issue with this question
If you've found an issue with this question, please let us know. With the help of the community we can continue to improve our educational resources.
DMCA Complaint
If you believe that content available by means of the Website (as defined in our Terms of Service) infringes one or more of your copyrights, please notify us by providing a written notice (“Infringement Notice”) containing the information described below to the designated agent listed below. If Varsity Tutors takes action in response to an Infringement Notice, it will make a good faith attempt to contact the party that made such content available by means of the most recent email address, if any, provided by such party to Varsity Tutors.
Your Infringement Notice may be forwarded to the party that made the content available or to third parties such as ChillingEffects.org.
Please be advised that you will be liable for damages (including costs and attorneys’ fees) if you materially misrepresent that a product or activity is infringing your copyrights. Thus, if you are not sure content located on or linked-to by the Website infringes your copyright, you should consider first contacting an attorney.
Please follow these steps to file a notice:
You must include the following:
A physical or electronic signature of the copyright owner or a person authorized to act on their behalf; An identification of the copyright claimed to have been infringed; A description of the nature and exact location of the content that you claim to infringe your copyright, in \ sufficient detail to permit Varsity Tutors to find and positively identify that content; for example we require a link to the specific question (not just the name of the question) that contains the content and a description of which specific portion of the question – an image, a link, the text, etc – your complaint refers to; Your name, address, telephone number and email address; and A statement by you: (a) that you believe in good faith that the use of the content that you claim to infringe your copyright is not authorized by law, or by the copyright owner or such owner’s agent; (b) that all of the information contained in your Infringement Notice is accurate, and (c) under penalty of perjury, that you are either the copyright owner or a person authorized to act on their behalf.
Send your complaint to our designated agent at:
Charles Cohn Varsity Tutors LLC 101 S. Hanley Rd, Suite 300 St. Louis, MO 63105
Or fill out the form below:
Contact Information
Complaint details.
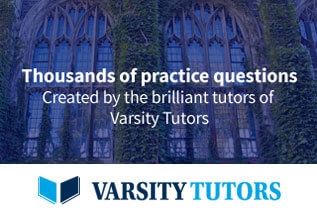

- HW Guidelines
- Study Skills Quiz
- Find Local Tutors
- Demo MathHelp.com
- Join MathHelp.com
Select a Course Below
- ACCUPLACER Math
- Math Placement Test
- PRAXIS Math
- + more tests
- 5th Grade Math
- 6th Grade Math
- Pre-Algebra
- College Pre-Algebra
- Introductory Algebra
- Intermediate Algebra
- College Algebra
Projectile Motion & Quadratic Equations
Projectiles General Word Problems Max/Min Problems
Calculus involves lots of finding maximums, minimums, and zeroes. You will get a taste of that with quadratic word problems. In fact, if/when you reach calculus, you will discover that some of the homework exercises will be identical to those you're doing now; it's just that you'll have new tools for finding the answers.
Content Continues Below
MathHelp.com
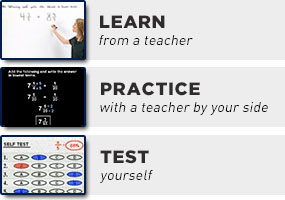
A major category of quadratic-equation word problems relates to what is called projectile motion. For our purposes, a projectile is any object that is thrown, shot, or dropped. Almost always, in this context, the object is initially moving directly up or straight down. (If it starts by going up then, naturally, it will later be coming back down.) This initial movement speed is the velocity.
What does the velocity's sign say about the object?
The initial velocity of the object, in these exercises, tells us how the object was released. The initial value of the velocity will be either zero (so the object was just dropped), positive (so it was thrown or shot upward), or negative (so the object was thrown downward).
What does " g " stand for?
Advertisement
In projectile-motion exercises, the coefficient on the squared term is −½ g . The g stands for the constant of gravity (on Earth), which is −9.8 meters per second square (that is meters per second per second) in metric terms, or −32 feet per second squared in Imperial terms. The "minus" signs reflect the fact that Earth's gravity pulls us, and the object in question, downward.
What does "per second squared" mean?
Acceleration (being the change in speed, rather than the speed itself) is measured in terms of how much the velocity changes per unit time. So, if the velocity of an object is measured in feet per second, then that object's acceleration says how much that velocity changes per unit time; that is, acceleration measures how much the feet per second changes per second. And this duplicate "per second" is how we get "second squared". It's from the physics of the situation.
Which value should I use for gravity?
If a projectile-motion exercise is stated in terms of feet, miles, or some other Imperial unit, then use −32 for gravity; if the units are meters, centimeters, or some other metric unit, then use −9.8 for gravity.
What is the projectile-motion equation?
The projectile-motion equation is s ( t ) = −½ g x 2 + v 0 x + h 0 , where g is the constant of gravity, v 0 is the initial velocity (that is, the velocity at time t = 0 ), and h 0 is the initial height of the object (that is, the height at of the object at t = 0 , the time of release).
Yes, you'll need to keep track of all of this stuff when working with projectile motion.
- An object is launched at 19.6 meters per second (m/s) from a 58.8 -meter tall platform. The equation for the object's height s at time t seconds after launch is s ( t ) = −4.9 t 2 + 19.6 t + 58.8 , where s is in meters. When does the object strike the ground?
What is the height (above ground level) when the object smacks into the ground? Well, zero, obviously. So I'm looking for the time when the height is s = 0 . I'll set s equal to zero, and solve:
0 = −4.9 t 2 + 19.6 t + 58.8 0 = t 2 − 4 t − 12 0 = ( t − 6)( t + 2)
Then t = 6 or t = −2 . The second solution is from two seconds before launch, which doesn't make sense in this context. (It makes sense on the graph, because the line crosses the x -axis at −2 , but negative time won't work in this word problem.) So " t = −2 " is an extraneous solution, and I'll ignore it.
Instead, my answer to this exercise (that is, the correct answer in context) is the other solution value. They asked me for the time, and time here is measured in seconds, so my answer is:
The object strikes the ground six seconds after launch.
Note the construction of the height equation in the problem above. (Yes, we went over this at the beginning, but you're really gonna need this info, so we're revisiting.)
The initial launch height was 58.8 meters, and the constant term was " 58.8 ". The initial velocity (or launch speed) was 19.6 m/s, and the coefficient on the linear term was " 19.6 ". This is always true for these up/down projectile motion problems. (If you have an exercise with sideways motion, the equation will have a different form, but they'll always give you that equation.) The initial velocity is the coefficient for the middle term, and the initial height is the constant term.
And the coefficient on the leading term comes from the force of gravity. This coefficient is negative, since gravity pulls downward, and the value will either be " −4.9 " (if your units are "meters") or " −16 " (if your units are "feet"). Yes, these values are half of the values listed for the gravity constant at the beginning of this page; they've had the ½ multiplied through.
In general, the projectile-motion equation's format is:
s ( t ) = − gt 2 + v 0 t + h 0
...where " g " here is the " 4.9 " or the " 16 " derived from the value of the force of gravity (technically, it's the force of gravity on Earth ), " v 0 " ("vee-naught", or "vee-sub-zero") is the initial velocity, and " h 0 " ("aitch-naught", or "aitch-sub-zero") is the initial height.
Memorize this equation (or at least its meaning), because you may need to know this on the test.
- An object in launched directly upward at 64 feet per second (ft/s) from a platform 80 feet high. What will be the object's maximum height? When will it attain this height?
Hmm... They didn't give me the equation this time. But that's okay, because I can create the equation from the information that they did give me. The initial height is 80 feet above ground and the initial speed is 64 ft/s upward. Since my units are feet, then the number for gravity will be −16 , and my equation is:
s ( t ) = −16 t 2 + 64 t + 80
They want me to find the maximum height. For a negative quadratic like this, the maximum will be at the vertex of the upside-down parabola. So they really want me to find the vertex. From graphing , I know how to find the vertex; in this case, the vertex is at ( h , k ) = (2, 144):
h = − b / 2 a = −(64)/2(−16) = −64/−32 = 2 k = s (2) = −16(2) 2 + 64(2) + 80 = −16(4) + 128 + 80 = 208 − 64 = 144
But what do the coordinates of this vertex tell me? According to my equation, I'm plugging in time values and extracting height values, so the input 2 was the time and the output 144 is the height.
It takes two seconds to reach the maximum height of 144 feet.
As long as you label clearly, you don't need a complete sentence (like I used above) for your hand-in answer. So you could also give you answer as, "time: 2 secs; height: 144 ft".
- An object is launched from ground level directly upward at 39.2 m/s. For how long is the object at or above a height of 34.3 meters?
My units this time are "meters", so the gravity number will be −4.9 . Since the object started at ground level, the initial height was 0 . Then my equation is:
s ( t ) = −4.9 t 2 + 39.2 t
Since this is a negative quadratic, the graph is an upside-down parabola. I can find the two times when the object is exactly 34.3 meters high, and I know that the object will be above 34.3 meters the whole time in between.
Why "two times", and how do I know that the time period is between those two times? Because the first time will be when the object passes a height of 34.3 meters on its way up to its maximum height, and the second time when be when it passes 34.3 meters as it is falling back down to the ground. So I have to solve the following:
−4.9 t 2 + 39.2 t = 34.3 t 2 − 8 t + 7 = 0 ( t − 7)( t − 1) = 0
The two solutions are at times t = 1 and t = 7 . So the object is at 34.3 meters at one second after launch (going up) and againt at seven seconds after launch (coming back down). Subtracting to find the difference, I find that:
The object is at or above 34.3 meters for six seconds.
Again, you don't technically need a complete sentence for your hand-in answer; saying " 6 secs" is probably good enough. But definitely do include the unit "seconds" on your answer.
Don't be surprised if many of your exercises work out as "neatly" as the above examples have. Many textbooks still engineer their exercises carefully, so that you can solve by factoring (that is, by quickly doing the algebra).
However (fair warning!), heavy dependence on calculators is leading more texts to create "interesting" (that is to say, needlessly complicated) exercises, so some (or all) of your exercises may involve much more messy computations than have been displayed here. If so, study these "neat" examples carefully, until you are quite sure you follow the reasoning.

- After the semester is over, you discover that the math department has changed textbooks (again) so the bookstore won't buy back your nearly-new book. You and your friend Herman decide to get creative. You go to the roof of a twelve-story building and look over the edge to the reflecting pool 160 feet below. You drop your book over the edge at the same instant that Herman chucks his book straight down at 48 feet per second. By how many seconds does his book beat yours into the water? (Round your answer to two decimal places.)
Our initial launch heights will be the same: we're both launching from 160 feet above ground. And the gravity number, since we're working in feet, will be −16 . My initial velocity is zero, since I just dropped my book, but my buddy Herman's velocity is a negative 48 , the negative coming from the fact that he chucked his book down rather than up. So our "height" equations are:
mine: s ( t ) = −16 t 2 + 160 his: s ( t ) = −16 t 2 − 48 t + 160
In each case, I need to find the time for the books to reach a height of zero (a height of zero being "ground level"), so:
mine: 0 = −16 t 2 + 160
t 2 − 10 = 0
his: 0 = −16 t 2 − 48 t + 160
t 2 + 3 t − 10 = 0
( t + 5)( t − 2) = 0
t = −5, +2
I will ignore the negative time values as being irrelevant in this context. (There may be a case, at some point, in some exercise, where a negative value does happen to be useful within a given context, so I do need to remember to think about the values and the context, rather than reflexively discarding any and all negative solutions.)
Herman's book hits the water about 1.16 seconds sooner than mine does.
Every once in a while, they'll get clever and put a "projectile" problem into a different environment. The equation will remain the same in structure, but you may have to account for a different value for gravity.
- The International Space Agency has finally landed a robotic explorer on an extra-solar planet. Some probes are extended from the lander's body to conduct various tests. To demonstrate the crushing weight of gravity on this planet, the lander's camera is aimed at a probe's ground-level ejection port, and the port launches a baseball directly upwards at 147 feet per second (ft/s), about the top speed of a professional pitcher. The force due to gravity on this planet is 98 ft/s 2 . Assuming no winds and that the probe can scurry out of the way in time, how long will it take for the ball to smack back into the surface?
To set up my equation for this exercise, I need to keep in mind that the value of the coefficient g from the projectile-motion equation above is one-half of the value of the force due to gravity in a given locale.
In physics, there is the "universal gravitational constant " G , being the gravitational pull inherent to our universe (or at least our region of it). Every object exerts its own gravitational force, which is related to its own mass and the universal constant G . In the "projectile motion" formula, the " g " is half of the value of the gravitational force for that particular body. For instance, the gravitational force on Earth is a downward 32 ft/s 2 , but we used 16 in the equation.
So g for my equation this time will by 98 ÷ 2 = 49 feet per second squared. Then:
s = −49 t 2 + 147 t 0 = −49 t 2 + 147 t 0 = t 2 − 3 t 0 = t ( t − 3)
Then t = 0 or t = 3 . The first solution represents when the ball was launched, so the second solution is the one I want.
It takes three seconds for the ball to hit the ground.
Note: On Earth, it would take a little over nine seconds for the ball to fall back to the ground.
URL: https://www.purplemath.com/modules/quadprob.htm
Page 1 Page 2 Page 3
Standardized Test Prep
College math, homeschool math, share this page.
- Terms of Use
- Privacy / Cookies
- About Purplemath
- About the Author
- Tutoring from PM
- Advertising
- Linking to PM
- Site licencing
Visit Our Profiles

If you're seeing this message, it means we're having trouble loading external resources on our website.
If you're behind a web filter, please make sure that the domains *.kastatic.org and *.kasandbox.org are unblocked.
To log in and use all the features of Khan Academy, please enable JavaScript in your browser.
Mechanics (Essentials) - Class 11th
Course: mechanics (essentials) - class 11th > unit 4.
- Intro to vectors and scalars
- Average speed & velocity (with examples)
Average velocity and speed in one direction: word problems
- Average velocity and speed with direction changes: word problems
- Average speed for entire journey - solved numerical
- Average velocity and speed review
- Your answer should be
- an exact decimal, like 0.75
Fill out the form to download
- Professional
- Academic (Researcher / Student)
I would like to sign up to receive news and updates from SimScale. See Privacy Policy .
- 1-99 employees
- 100-249 employees
- 250-499 employees
- 500+ employees
- Chemical Processing
- Electromagnetic
- Engineering Office
- Heat Exchangers
- Heavy Industry
- Lighting LED
- Machine Tool Building
- Material Processing
- No Industry Focus
- Oil & Gas industry
- Pharmaceutical Production
- Power Generation
- Process Engineering
- Pumps and Valves
- Rapid Prototyping
- Turbomachinery
- Fluid Dynamics (CFD)
- Multiple Disciplines
- Structural Mechanics (FEA)
- Thermodynamics
- Intermediate
Thank you. We will contact you shortly.
Set up your own cloud-native simulation in minutes.
Impeller Design: Types, Applications, and Simulation
Samir Jaber
published on
May 15th, 2024
approx reading time
An impeller is a rotating component designed to transfer energy from the motor to the fluid, increasing its velocity and pressure as it moves through the machine. A good impeller design ensures optimal fluid dynamics, minimizes energy losses, and contributes to the longevity of the turbomachinery equipment.
In this article, we will discuss the details of impeller design, its challenges, and their solutions. We will also examine how engineering simulation, especially cloud-native simulation, enables engineers to create more efficient and reliable impellers.
Basic Principles of Impeller Design
Impeller design uses fundamental fluid dynamics and energy transfer principles to function effectively. The primary function of an impeller is to convert mechanical energy from a motor into kinetic energy in the fluid. This process is governed by several fundamental principles and equations.
Bernoulli’s Equation
One of the foundational equations in fluid dynamics is Bernoulli’s equation, which describes energy conservation in a flowing fluid. It states that the total mechanical energy of the fluid remains constant along a streamline. The equation is given by:
$$ P = \frac{1}{2}\rho v^2 + \rho gh = constant $$
- \(P\) is the static pressure.
- \(\rho\) is the fluid density.
- \(v\) is the fluid velocity.
- \(g\) is the acceleration due to gravity.
- \(h\) is the height above a reference point.
Euler’s Turbomachinery Equation
Another critical principle is Euler’s turbomachinery equation, which relates the change in fluid energy to the impeller’s geometry and rotational speed. It is given by:
$$ \Delta H = \frac{U_2 V_{u2} – U_1 V_{u1}}{g} $$
- \(\Delta H\) is the head increase imparted to the fluid.
- \(U\) is the tangential velocity of the impeller.
- \(V_u\) is the tangential component of the absolute velocity of the fluid at the inlet (1) and outlet (2) of the impeller.
This equation is essential for determining the work done by the impeller on the fluid and is used to calculate the pressure increase provided by the impeller.
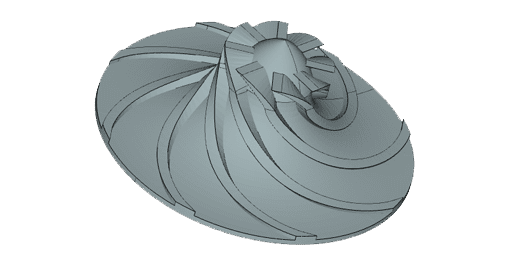
Key Design Parameters
The design of the impeller itself involves several key geometric parameters that influence its performance. These include:
- Impeller Diameter: The impeller diameter impacts both the head and flow rate. Larger diameters increase head and flow but also raise energy consumption. The relationship is approximated by the affinity law: \(H \propto D^2\)
- Blade Angle: Blade angles at the inlet (\(\beta_1)\) and outlet (\(\beta_2)\) are crucial for smooth fluid entry and exit, minimizing flow separation and turbulence. Optimized angles enhance energy transfer efficiency.
- Number of Blades: More blades reduce fluid slip and improve efficiency but increase manufacturing complexity. The optimal number balances efficiency and practical considerations.
- Blade Shape and Curvature: Curved blades guide fluid better than straight ones, reducing turbulence and energy losses. The blade shape is tailored to specific applications, such as radial, mixed-flow, or axial-flow impellers.
- Impeller Width: Impeller width affects flow rate and efficiency. Wider impellers handle larger flow rates but may increase friction losses. Narrower impellers are more efficient but support lower flow rates.
- Material Selection: Material choice impacts durability and resistance to wear and corrosion. Common materials include stainless steel, cast iron, and various alloys, selected based on operating conditions.
- Surface Finish: A smooth surface finish on blades and shrouds reduces friction and turbulence, enhancing hydraulic efficiency. Precision casting and surface coatings can improve the surface finish.
Types of Impellers
Table 1 below shows the types of impellers used in turbomachinery equipment (pumps or turbines).
Role of Engineering Simulation in Impeller Design
Simulating and evaluating a pump impeller early in the design process is crucial for determining the optimal design. However, traditional on-premises simulation tools are often costly and have steep learning curves.
Cloud-native simulation solutions offer significant advantages over traditional on-premises simulations. They provide scalable computing power, enabling engineers to run large-scale and complex simulations without local hardware limitations. Tools like SimScale eliminate these barriers by leveraging the power of the cloud.
With SimScale, engineers can:
- Optimize impeller designs faster by running several simulations simultaneously
- Use FEA and thermal analysis to test the stress and strain applied to pump impellers
- Get started quickly on an easy-to-use interface without extensive training
- Access cost-effective solutions and faster processing times
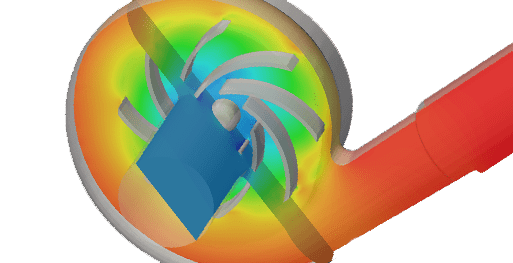
Solving Cavitation Problems in Pumps
Cavitation is the formation of vapor bubbles inside a liquid with low pressure and high flow velocity. It is the leading cause of performance deterioration in pumps and turbines, significantly affecting impellers.
SimScale offers advanced simulation tools that allow engineers to model and analyze cavitation effects in pumps and turbomachinery. Using SimScale, engineers can:
- Conduct Comprehensive Analyses: Utilize computational fluid dynamics (CFD) , structural (FEA) , and thermal analyses through automated workflows and intuitive interfaces.
- Model Cavitation Phenomena: Understand the impact of cavitation on performance by simulating cavitating flow and studying parameters like the net positive suction head required (NPSHR), cavitation number, and inlet sizing.
- Optimize Pump Efficiency: Use the Subsonic CFD solver to study and optimize pressure drop, fluid flow patterns, and cavitation effects. The solver’s robust meshing strategy ensures high-quality meshes and faster simulations.
- Import and Edit CAD Models: Easily import CAD models from various software and perform essential operations like flow volume extraction and defining rotating zones.
- Visualize Results: Leverage advanced visualization tools to analyze flow behavior, pressure distribution, velocity vectors, and cavitation effects.
Tecnalia’s Use of Cloud-Native Simulation in UAS Development
Tecnalia is a leading global research firm and Spain’s largest applied research and technological development organization. It designs and develops new technologies for drones and unmanned aircraft systems (UAS) for inspection, transport, and maintenance tasks. Tecnalia collaborates with industrial partners, local administrations, and end-users to improve and optimize transportation vehicles and their integration into urban ecosystems. It aims to develop efficient, environmentally friendly solutions for future sustainable and intelligent urban mobility.

Using SimScale, the Tecnalia team conducted detailed CFD simulations to optimize the design early in the process for the aerial vehicle from the Concept Integrity demonstrator. Extensive CFD analysis ensured aerodynamic efficiency and stable performance in various conditions, saving significant time and cost compared to physical testing. Read more in Tecnalia’s success story with SimScale .
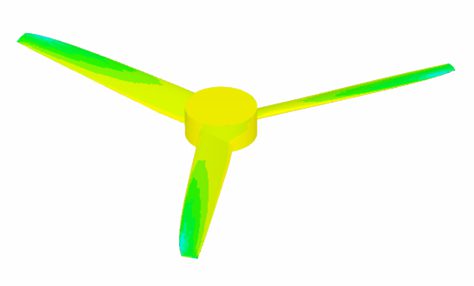
Optimize Impeller Design With SimScale Cloud-Native Simulation
Are you seeking faster innovation and higher impeller design efficiency? SimScale offers a robust solution for reducing the time and cost associated with design and prototyping while maximizing accuracy and enhancing decision-making.
For engineers and designers aiming to push the boundaries of impeller design, SimScale provides the flexibility to explore innovative turbomachinery modeling . With SimScale’s integration with CFturbo , users can boost their impeller design modeling, benefiting from a seamless workflow that allows for faster and more accurate design and simulations.
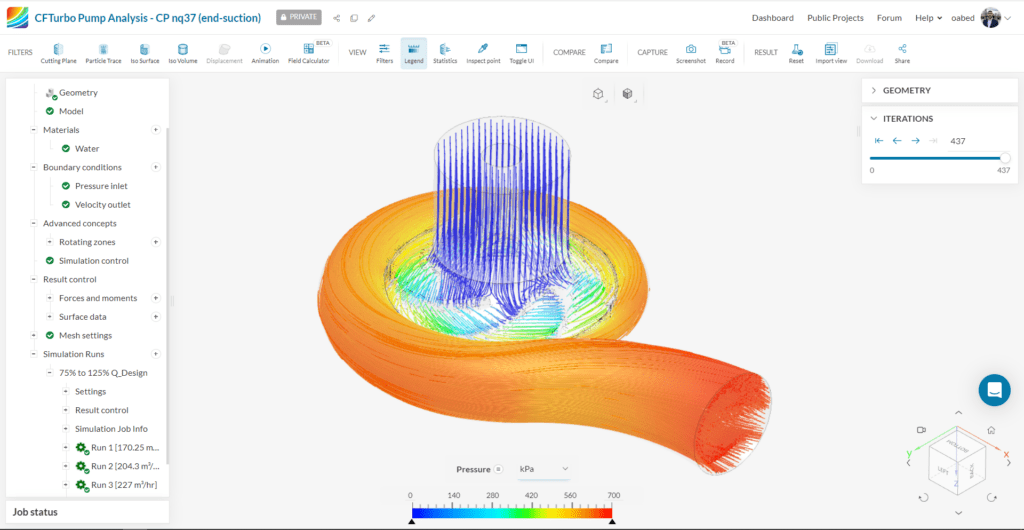
SimScale’s advanced simulation capabilities enable you to test and validate innovative concepts that might be impractical or too risky to prototype traditionally. Experience the power of cloud-native simulation with SimScale. Sign up below and start simulating today.
Set up your own cloud-native simulation via the web in minutes by creating an account on the SimScale platform. No installation, special hardware, or credit card is required.
Stay updated and never miss an article!
Other 'computational fluid dynamics (cfd)' stories.
Your hub for everything you need to know about simulation and the world of CAE
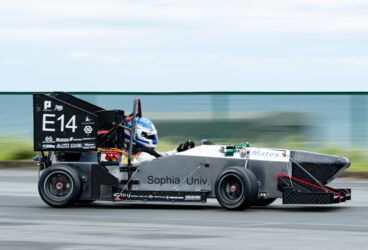
Team Sophia Racing: Student Success Story
EV Battery Cooling: Enhancing Efficiency with Simulation
Building Downwash: 5 Key Strategies to Counteract Urban Wind Discomfort
Sign up for SimScale
and start simulating now
A Reds-A's trade to solve David Bell's Alexis Diaz problem
By zach pressnell | may 15, 2024.
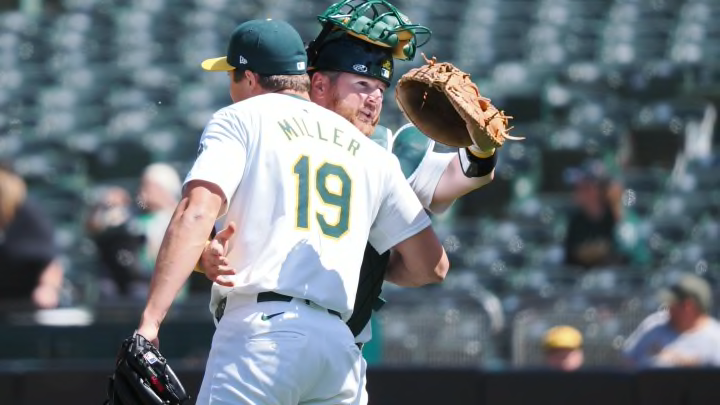
To put it simply, the Cincinnati Reds have an Alexis Diaz problem. During the first half of 2023, their All-Star closer was lights out. Across his first 45 appearances of 2023, he held an ERA of 1.85 and had slammed the door nearly every time he took the mound.
Since that day, Diaz has been horrendous for Cincinnati. Across the last 42 appearances, Diaz has suffered seven losses, has an ERA near six and has only recorded 16 saves. In 2024, Diaz's ERA is a 6.75 and he's blown two of his nine save attempts. His 7.4 BB/9 is a horrendous number for Reds fans to look at.
What better way to solve this problem than by adding one of the game's most dominant bullpen arms of 2024?
A Reds-A's trade that would solve the Alexis Diaz problem in Cincinnati
There isn't a more popular bullpen arm in the media than that of the Oakland A's Mason Miller. Miller has seen his name attached to teams like the Red Sox, Cubs, and Phillies. Now, the idea of moving him to the Reds can be pitched.
The asking price for Miller is higher than most teams may be willing to pay. But most teams don't have the prospect capital that Cincinnati does. Last season, the Reds held one of the top farm systems in the entire league, graduating names like Christian Encarnacion-Strand, Elly De La Cruz and Matt McLain.
Reds-A's trade for Mason Miller
To acquire a 25-year-old closer like Mason Miller, Cincinnati could put together a trade package of Lyon Richardson and Blake Dunn to send to Oakland. Both Dunn and Richardson are MLB ready prospects listed in the Reds top 15 prospects, according to MLB Pipeline.
Oakland may be more attracted to some of the Reds' younger, higher ceiling prospects like Carlos Jorge or Hector Rodriguez, but it's quite unlikely that Cincinnati's front office would part ways with those two for a reliever, no matter how dominant.
Cincinnati could absolutely use the services of an improving 25-year-old closer that's been as impressive as Miller has been. Adding him to the bullpen would take stress off the shoulders of Alexis Diaz, giving Cincinnati a trio of Fernando Cruz, Alexis Diaz and Mason Miller that could shut down games in the Queen City throughout 2024.
Oakland will likely look to capitalize on Miller's value being higher than it ever will be. Cincinnati needs to start working to turn their train wreck of a season around before it's too late.
Help | Advanced Search
Mathematics > Analysis of PDEs
Title: the obstacle problem for linear scalar conservation laws with constant velocity.
Abstract: In this contribution, we present a novel approach for solving the obstacle problem for (linear) conservation laws. Usually, given a conservation law with an initial datum, the solution is uniquely determined. How to incorporate obstacles, i.e., inequality constraints on the solution so that the resulting solution is still "physically reasonable" and obeys the obstacle, is unclear. The proposed approach involves scaling down the velocity of the conservation law when the solution approaches the obstacle. We demonstrate that this leads to a reasonable solution and show that, when scaling down is performed in a discontinuous fashion, we still obtain a suitable velocity - and the solution satisfying a discontinuous conservation law. We illustrate the developed solution concept using numerical approximations.
Submission history
Access paper:.
- HTML (experimental)
- Other Formats

References & Citations
- Google Scholar
- Semantic Scholar
BibTeX formatted citation

Bibliographic and Citation Tools
Code, data and media associated with this article, recommenders and search tools.
- Institution
arXivLabs: experimental projects with community collaborators
arXivLabs is a framework that allows collaborators to develop and share new arXiv features directly on our website.
Both individuals and organizations that work with arXivLabs have embraced and accepted our values of openness, community, excellence, and user data privacy. arXiv is committed to these values and only works with partners that adhere to them.
Have an idea for a project that will add value for arXiv's community? Learn more about arXivLabs .

IMAGES
VIDEO
COMMENTS
The required equations and background reading to solve these problems is given on the kinematics page. Problem # 1 A car travels at uniform velocity a distance of 100 m in 4 seconds. What is the velocity of the car? (Answer: 25 m/s) Problem # 2 A sailboat is traveling north at 10 km/h, relative to the water. The water is flowing north at 5 km/h.
This is a powerful interactive animation, and it can be used for many lessons. At this point it can be used to show that displacement can be either positive or negative. It can also show that when displacement is negative, velocity can be either positive or negative. Later it can be used to show that velocity and acceleration can have different ...
Kinematic equations relate the variables of motion to one another. Each equation contains four variables. The variables include acceleration (a), time (t), displacement (d), final velocity (vf), and initial velocity (vi). If values of three variables are known, then the others can be calculated using the equations. This page demonstrates the process with 20 sample problems and accompanying ...
Problem 8: A man walked from point A to F following the route in the grid below in 3250 seconds. Determine a) the average speed, in m/s, for the whole journey. b) the magnitude of the displacement. c) the magnitude of the average velocity, in m/s, for the whole journey. Solution to Problem 8: a)
The first answer was correct, displacement does need a quantity and direction. Displacement can be calculated by measuring the final distance away from a point, and then subtracting the initial distance. Displacement is key when determining velocity (which is also a vector). Velocity = displacement/time whereas speed is distance/time.
In This video lesson of the Basic Physics Series, DrD "The Explainer" explains how to set up and SOLVE 8 velocity problems in a guided practice . He teaches ...
How to use the velocity equation, v=D/t to solve simple velocity. This video is a great help for students of Physical Science and beginning Physics. The vide...
Velocity is defined as the rate of travel of an object, along with its direction. Velocity tells you how fast an object is moving as well as in what direction it is moving. It is similar to speed ...
Velocity (v) is a vector quantity that measures displacement (or change in position, Δs) over the change in time (Δt), represented by the equation v = Δs/Δt. Speed (or rate, r) is a scalar quantity that measures the distance traveled (d) over the change in time (Δt), represented by the equation r = d/Δt. Created by Sal Khan.
Velocity can be calculated by measuring the object's displacement over the time taken to displace it. Velocity= Displacement Time =>v = d t Velocity = Displacement Time => v = d t. In vectorial notation, velocity is given by. →v = Δ→x t v → = Δ x → t. Units. The SI unit of velocity is Newtons per second of N/s.
Solving for time. Rate of change in position, or speed, is equal to distance traveled divided by time. To solve for time, divide the distance traveled by the rate. For example, if Cole drives his car 45 km per hour and travels a total of 225 km, then he traveled for 225/45 = 5 hours. Created by Sal Khan.
The use of this problem-solving strategy in the solution of the following problem is modeled in Examples A and B below. Example Problem A. Ima Hurryin is approaching a stoplight moving with a velocity of +30.0 m/s. The light turns yellow, and Ima applies the brakes and skids to a stop.
Courses on Khan Academy are always 100% free. Start practicing—and saving your progress—now: https://www.khanacademy.org/science/physics/one-dimensional-mot...
Velocity = 130 m 100 s East = 1.3 m/s East. You forgot your money so you turn around and go back home in 120 more seconds: what is your round-trip speed and velocity? The total time is 100 s + 120 s = 220 s: Speed = 440 m 220 s = 2.0 m/s. Velocity = 0 m 220 s = 0 m/s. Yes, the velocity is zero as you ended up where you started.
Velocity is an object's speed in a particular direction. Mathematically, velocity is often described as the change in position over the change in time. ... Solve problems in two dimensions. Not all word problems involve movement back along one line. If the object turns at some point, you may need to draw a diagram and solve a geometry problem ...
Problem 8: A man walked from point A to F following the route in the grid below in 3250 seconds. Determine. a) the average speed, in m/s, for the whole journey. b) the magnitude of the displacement. c) the magnitude of the average velocity, in m/s, for the whole journey. Solution to Problem 8.
In this article, you will learn how to solve speed, velocity, and acceleration problems. Additionally, you need to know that speed is a scalar quantity and we can write its symbol as S. The formula for calculating the speed of an object is: ... Solved Problems of Speed, Velocity, and Acceleration. Here are solved problems to help you understand ...
During this round trip, the car returns to its original position, and thus its displacement, which defines the average velocity, is zero. Therefore, \[\text{average velocity}=0\,\rm m/s\] Acceleration Problems. Problem (9): A car moves from rest to a speed of $45\,\rm m/s$ in a time interval of $15\,\rm s$. At what rate does the car accelerate?
Position, velocity, acceleration problems and solutions. When solving a Physics problem in general and one of Kinematics in particular, it is important that you follow an order. Get used to being organized when you solve problems, and you will see how it gives good results. It is worth spending a little time on the previous analysis of a ...
Example Question #1 : Solve Linear Velocity Problems. Find the linear velocity in meters per second of an object if it took hours to travel a distance of kilometers. Round to the nearest integer. Possible Answers: Correct answer: Explanation: Write the linear velocity formula.
The projectile-motion equation is s(t) = −½ gx2 + v0x + h0, where g is the constant of gravity, v0 is the initial velocity (that is, the velocity at time t = 0 ), and h0 is the initial height of the object (that is, the height at of the object at t = 0, the time of release). Yes, you'll need to keep track of all of this stuff when working ...
Learn for free about math, art, computer programming, economics, physics, chemistry, biology, medicine, finance, history, and more. Khan Academy is a nonprofit with the mission of providing a free, world-class education for anyone, anywhere.
Solving Cavitation Problems in Pumps. Cavitation is the formation of vapor bubbles inside a liquid with low pressure and high flow velocity. It is the leading cause of performance deterioration in pumps and turbines, significantly affecting impellers.
Learn how to solve a velocity, distance, and time problem with a simple equation and clear examples in this video.
A Reds-A's trade that would solve the Alexis Diaz problem in Cincinnati. There isn't a more popular bullpen arm in the media than that of the Oakland A's Mason Miller.
In this contribution, we present a novel approach for solving the obstacle problem for (linear) conservation laws. Usually, given a conservation law with an initial datum, the solution is uniquely determined. How to incorporate obstacles, i.e., inequality constraints on the solution so that the resulting solution is still "physically reasonable" and obeys the obstacle, is unclear. The proposed ...
Abstract. In this paper, a control scheme is presented to solve the problem of path tracking for varying-velocity autonomous vehicles with higher robust performance while friction force constraints of vehicle tires and ground are considered to avoid appearing the steering lost situation actively in the limit of the tire friction forces by using four wheel steering.
The force decomposition approach is extended to solve the two-dimensional (2D) water entry and exit problems. Two typical scenarios, direct water exit and continuous water entry and exit, are examined. It reveals that the total force is irrelevant to the body velocity but to the acceleration. More specifically, the force can be expressed as the multiplication of constant values, body ...