1.7 Solving Problems in Physics
Learning objectives.
By the end of this section, you will be able to:
- Describe the process for developing a problem-solving strategy.
- Explain how to find the numerical solution to a problem.
- Summarize the process for assessing the significance of the numerical solution to a problem.
Problem-solving skills are clearly essential to success in a quantitative course in physics. More important, the ability to apply broad physical principles—usually represented by equations—to specific situations is a very powerful form of knowledge. It is much more powerful than memorizing a list of facts. Analytical skills and problem-solving abilities can be applied to new situations whereas a list of facts cannot be made long enough to contain every possible circumstance. Such analytical skills are useful both for solving problems in this text and for applying physics in everyday life.
As you are probably well aware, a certain amount of creativity and insight is required to solve problems. No rigid procedure works every time. Creativity and insight grow with experience. With practice, the basics of problem solving become almost automatic. One way to get practice is to work out the text’s examples for yourself as you read. Another is to work as many end-of-section problems as possible, starting with the easiest to build confidence and then progressing to the more difficult. After you become involved in physics, you will see it all around you, and you can begin to apply it to situations you encounter outside the classroom, just as is done in many of the applications in this text.
Although there is no simple step-by-step method that works for every problem, the following three-stage process facilitates problem solving and makes it more meaningful. The three stages are strategy, solution, and significance. This process is used in examples throughout the book. Here, we look at each stage of the process in turn.
Strategy is the beginning stage of solving a problem. The idea is to figure out exactly what the problem is and then develop a strategy for solving it. Some general advice for this stage is as follows:
- Examine the situation to determine which physical principles are involved . It often helps to draw a simple sketch at the outset. You often need to decide which direction is positive and note that on your sketch. When you have identified the physical principles, it is much easier to find and apply the equations representing those principles. Although finding the correct equation is essential, keep in mind that equations represent physical principles, laws of nature, and relationships among physical quantities. Without a conceptual understanding of a problem, a numerical solution is meaningless.
- Make a list of what is given or can be inferred from the problem as stated (identify the “knowns”) . Many problems are stated very succinctly and require some inspection to determine what is known. Drawing a sketch can be very useful at this point as well. Formally identifying the knowns is of particular importance in applying physics to real-world situations. For example, the word stopped means the velocity is zero at that instant. Also, we can often take initial time and position as zero by the appropriate choice of coordinate system.
- Identify exactly what needs to be determined in the problem (identify the unknowns) . In complex problems, especially, it is not always obvious what needs to be found or in what sequence. Making a list can help identify the unknowns.
- Determine which physical principles can help you solve the problem . Since physical principles tend to be expressed in the form of mathematical equations, a list of knowns and unknowns can help here. It is easiest if you can find equations that contain only one unknown—that is, all the other variables are known—so you can solve for the unknown easily. If the equation contains more than one unknown, then additional equations are needed to solve the problem. In some problems, several unknowns must be determined to get at the one needed most. In such problems it is especially important to keep physical principles in mind to avoid going astray in a sea of equations. You may have to use two (or more) different equations to get the final answer.
The solution stage is when you do the math. Substitute the knowns (along with their units) into the appropriate equation and obtain numerical solutions complete with units . That is, do the algebra, calculus, geometry, or arithmetic necessary to find the unknown from the knowns, being sure to carry the units through the calculations. This step is clearly important because it produces the numerical answer, along with its units. Notice, however, that this stage is only one-third of the overall problem-solving process.

Significance
After having done the math in the solution stage of problem solving, it is tempting to think you are done. But, always remember that physics is not math. Rather, in doing physics, we use mathematics as a tool to help us understand nature. So, after you obtain a numerical answer, you should always assess its significance:
- Check your units. If the units of the answer are incorrect, then an error has been made and you should go back over your previous steps to find it. One way to find the mistake is to check all the equations you derived for dimensional consistency. However, be warned that correct units do not guarantee the numerical part of the answer is also correct.
- Check the answer to see whether it is reasonable. Does it make sense? This step is extremely important: –the goal of physics is to describe nature accurately. To determine whether the answer is reasonable, check both its magnitude and its sign, in addition to its units. The magnitude should be consistent with a rough estimate of what it should be. It should also compare reasonably with magnitudes of other quantities of the same type. The sign usually tells you about direction and should be consistent with your prior expectations. Your judgment will improve as you solve more physics problems, and it will become possible for you to make finer judgments regarding whether nature is described adequately by the answer to a problem. This step brings the problem back to its conceptual meaning. If you can judge whether the answer is reasonable, you have a deeper understanding of physics than just being able to solve a problem mechanically.
- Check to see whether the answer tells you something interesting. What does it mean? This is the flip side of the question: Does it make sense? Ultimately, physics is about understanding nature, and we solve physics problems to learn a little something about how nature operates. Therefore, assuming the answer does make sense, you should always take a moment to see if it tells you something about the world that you find interesting. Even if the answer to this particular problem is not very interesting to you, what about the method you used to solve it? Could the method be adapted to answer a question that you do find interesting? In many ways, it is in answering questions such as these that science progresses.
As an Amazon Associate we earn from qualifying purchases.
This book may not be used in the training of large language models or otherwise be ingested into large language models or generative AI offerings without OpenStax's permission.
Want to cite, share, or modify this book? This book uses the Creative Commons Attribution License and you must attribute OpenStax.
Access for free at https://openstax.org/books/university-physics-volume-1/pages/1-introduction
- Authors: William Moebs, Samuel J. Ling, Jeff Sanny
- Publisher/website: OpenStax
- Book title: University Physics Volume 1
- Publication date: Sep 19, 2016
- Location: Houston, Texas
- Book URL: https://openstax.org/books/university-physics-volume-1/pages/1-introduction
- Section URL: https://openstax.org/books/university-physics-volume-1/pages/1-7-solving-problems-in-physics
© Jan 19, 2024 OpenStax. Textbook content produced by OpenStax is licensed under a Creative Commons Attribution License . The OpenStax name, OpenStax logo, OpenStax book covers, OpenStax CNX name, and OpenStax CNX logo are not subject to the Creative Commons license and may not be reproduced without the prior and express written consent of Rice University.
Mastering Physics Problem-Solving: A Comprehensive 6-Step Guide
Introduction.
Physics problems can often be daunting, but with a systematic approach, they become manageable challenges. In this guide, we will explore a detailed six-step process designed to enhance your problem-solving skills. Whether you are a student navigating your physics coursework or a physics enthusiast delving into complex scenarios, these steps will provide a solid foundation for tackling any physics problem effectively.
1. Commence with a Clear and Comprehensive Diagram
The importance of visualization in physics cannot be overstated. To kickstart your problem-solving journey, begin by drawing a clear and comprehensive diagram. This visual representation serves as a roadmap, aiding in the understanding of the problem’s intricacies. It enables you to decipher the given information and conceptualize the scenario, providing a tangible foundation for the subsequent steps.
Consider a scenario where you are tasked with understanding the motion of objects in a gravitational field. A well-drawn diagram could depict the initial and final positions, velocities, and any forces at play. This step ensures that you have a tangible representation of the problem, helping to organize your thoughts and set the stage for a systematic solution.
2. Systematically Transfer Data to the Diagram
With the diagram in place, the next step involves systematically transferring all pertinent data and information onto it. This process serves a dual purpose – it helps you internalize the details of the problem, and it minimizes the need to revisit the question repeatedly during the solution phase. Efficiently transferring information ensures that you have a clear reference point for the specifics of the given scenario.
For instance, if dealing with a dynamics problem involving multiple forces, annotate the magnitudes, directions, and points of application directly on the diagram. This step ensures that you have a consolidated source of information, reducing the chances of overlooking critical details during the subsequent stages of problem-solving.
3. Identify Relevant Concepts
Physics problems often encompass various concepts and principles. Identifying the relevant ones is crucial for crafting a targeted solution. As you examine the given problem, consider the fundamental physics principles at play. This step requires a solid understanding of the underlying theories and laws applicable to the specific scenario.
Continuing with the example of objects in a gravitational field, you would identify concepts such as Newton’s laws of motion and the principles of gravitational acceleration. Recognizing these fundamental ideas guides the subsequent steps, providing a conceptual framework for deriving and applying the necessary equations.
4. Establish Correct Equations
Once you have a conceptual framework in place, the next step involves establishing the correct equations. At this stage, resist the temptation to substitute numerical values. Instead, focus on the relationships between the physical quantities involved. Derive or identify the equations that encapsulate the principles relevant to the given scenario.
For our gravitational field example, this step might involve recognizing the kinematic equations related to the motion of objects under constant acceleration. Establishing these equations sets the stage for a more structured and conceptual solution, laying the groundwork for the subsequent numerical analysis.
5. Integrate Numerical Values into Simplified Equations
With the equations identified, it’s time to introduce numerical values. Before doing so, ensure that the units across all quantities are consistent. If necessary, convert units to the International System of Units (SI) for uniformity. This step is crucial for maintaining precision throughout the solution process.
Consider a scenario where time is initially given in minutes, but the chosen equation requires seconds. Converting units beforehand prevents errors and ensures that the subsequent calculations are accurate. This meticulous approach contributes to the overall accuracy and reliability of the solution.

6. Present the Final Answer with Precision
The final step in this comprehensive guide involves presenting the solution with precision. State the numerical answer with the appropriate number of significant figures or decimal places, accompanied by the correct unit. This attention to detail is essential for conveying the accuracy of your solution and aligning with the standards of scientific reporting.
In our gravitational field example, if the calculated displacement is expressed as 25.678 meters, the final answer should be presented with the appropriate precision – perhaps as 25.7 meters or 2.57 x 10^1 meters, depending on the context and significant figures involved.
Mastering physics problem-solving is a journey that involves a combination of visualization, systematic data organization, conceptual understanding, and precision in numerical analysis. By following this six-step guide, you can navigate through complex physics scenarios with confidence, developing a robust problem-solving skill set that is applicable across various physics disciplines. Embrace the challenge, cultivate a disciplined approach, and watch as your proficiency in solving physics problems reaches new heights.
Back To A Level Physics Topic List
Mini Physics
As the Administrator of Mini Physics, I possess a BSc. (Hons) in Physics. I am committed to ensuring the accuracy and quality of the content on this site. If you encounter any inaccuracies or have suggestions for enhancements, I encourage you to contact us . Your support and feedback are invaluable to us. If you appreciate the resources available on this site, kindly consider recommending Mini Physics to your friends. Together, we can foster a community passionate about Physics and continuous learning.
Leave a Comment Cancel reply
This site uses Akismet to reduce spam. Learn how your comment data is processed .
1 Units and Measurement
1.7 solving problems in physics, learning objectives.
By the end of this section, you will be able to:
- Describe the process for developing a problem-solving strategy.
- Explain how to find the numerical solution to a problem.
- Summarize the process for assessing the significance of the numerical solution to a problem.
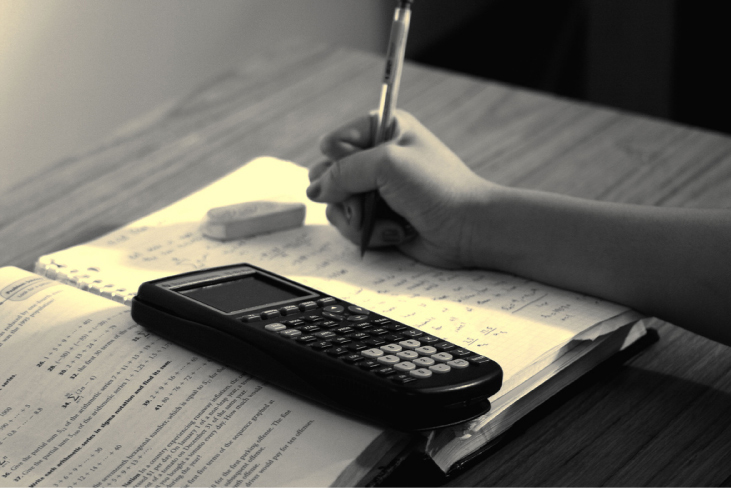
Figure 1.13 Problem-solving skills are essential to your success in physics. (credit: “scui3asteveo”/Flickr)
Problem-solving skills are clearly essential to success in a quantitative course in physics. More important, the ability to apply broad physical principles—usually represented by equations—to specific situations is a very powerful form of knowledge. It is much more powerful than memorizing a list of facts. Analytical skills and problem-solving abilities can be applied to new situations whereas a list of facts cannot be made long enough to contain every possible circumstance. Such analytical skills are useful both for solving problems in this text and for applying physics in everyday life.
As you are probably well aware, a certain amount of creativity and insight is required to solve problems. No rigid procedure works every time. Creativity and insight grow with experience. With practice, the basics of problem solving become almost automatic. One way to get practice is to work out the text’s examples for yourself as you read. Another is to work as many end-of-section problems as possible, starting with the easiest to build confidence and then progressing to the more difficult. After you become involved in physics, you will see it all around you, and you can begin to apply it to situations you encounter outside the classroom, just as is done in many of the applications in this text.
Although there is no simple step-by-step method that works for every problem, the following three-stage process facilitates problem solving and makes it more meaningful. The three stages are strategy, solution, and significance. This process is used in examples throughout the book. Here, we look at each stage of the process in turn.
Strategy is the beginning stage of solving a problem. The idea is to figure out exactly what the problem is and then develop a strategy for solving it. Some general advice for this stage is as follows:
- Examine the situation to determine which physical principles are involved . It often helps to draw a simple sketch at the outset. You often need to decide which direction is positive and note that on your sketch. When you have identified the physical principles, it is much easier to find and apply the equations representing those principles. Although finding the correct equation is essential, keep in mind that equations represent physical principles, laws of nature, and relationships among physical quantities. Without a conceptual understanding of a problem, a numerical solution is meaningless.
- Make a list of what is given or can be inferred from the problem as stated (identify the “knowns”) . Many problems are stated very succinctly and require some inspection to determine what is known. Drawing a sketch can be very useful at this point as well. Formally identifying the knowns is of particular importance in applying physics to real-world situations. For example, the word stopped means the velocity is zero at that instant. Also, we can often take initial time and position as zero by the appropriate choice of coordinate system.
- Identify exactly what needs to be determined in the problem (identify the unknowns) . In complex problems, especially, it is not always obvious what needs to be found or in what sequence. Making a list can help identify the unknowns.
- Determine which physical principles can help you solve the problem . Since physical principles tend to be expressed in the form of mathematical equations, a list of knowns and unknowns can help here. It is easiest if you can find equations that contain only one unknown—that is, all the other variables are known—so you can solve for the unknown easily. If the equation contains more than one unknown, then additional equations are needed to solve the problem. In some problems, several unknowns must be determined to get at the one needed most. In such problems it is especially important to keep physical principles in mind to avoid going astray in a sea of equations. You may have to use two (or more) different equations to get the final answer.
The solution stage is when you do the math. Substitute the knowns (along with their units) into the appropriate equation and obtain numerical solutions complete with units . That is, do the algebra, calculus, geometry, or arithmetic necessary to find the unknown from the knowns, being sure to carry the units through the calculations. This step is clearly important because it produces the numerical answer, along with its units. Notice, however, that this stage is only one-third of the overall problem-solving process.
Significance
After having done the math in the solution stage of problem solving, it is tempting to think you are done. But, always remember that physics is not math. Rather, in doing physics, we use mathematics as a tool to help us understand nature. So, after you obtain a numerical answer, you should always assess its significance:
- Check your units. If the units of the answer are incorrect, then an error has been made and you should go back over your previous steps to find it. One way to find the mistake is to check all the equations you derived for dimensional consistency. However, be warned that correct units do not guarantee the numerical part of the answer is also correct.
- Check the answer to see whether it is reasonable. Does it make sense? This step is extremely important: –the goal of physics is to describe nature accurately. To determine whether the answer is reasonable, check both its magnitude and its sign, in addition to its units. The magnitude should be consistent with a rough estimate of what it should be. It should also compare reasonably with magnitudes of other quantities of the same type. The sign usually tells you about direction and should be consistent with your prior expectations. Your judgment will improve as you solve more physics problems, and it will become possible for you to make finer judgments regarding whether nature is described adequately by the answer to a problem. This step brings the problem back to its conceptual meaning. If you can judge whether the answer is reasonable, you have a deeper understanding of physics than just being able to solve a problem mechanically.
- Check to see whether the answer tells you something interesting. What does it mean? This is the flip side of the question: Does it make sense? Ultimately, physics is about understanding nature, and we solve physics problems to learn a little something about how nature operates. Therefore, assuming the answer does make sense, you should always take a moment to see if it tells you something about the world that you find interesting. Even if the answer to this particular problem is not very interesting to you, what about the method you used to solve it? Could the method be adapted to answer a question that you do find interesting? In many ways, it is in answering questions such as these that science progresses.
The three stages of the process for solving physics problems used in this book are as follows:
- Strategy : Determine which physical principles are involved and develop a strategy for using them to solve the problem.
- Solution : Do the math necessary to obtain a numerical solution complete with units.
- Significance : Check the solution to make sure it makes sense (correct units, reasonable magnitude and sign) and assess its significance.
Conceptual Questions
What information do you need to choose which equation or equations to use to solve a problem?
What should you do after obtaining a numerical answer when solving a problem?
Check to make sure it makes sense and assess its significance.
Additional Problems
Consider the equation y = mt +b , where the dimension of y is length and the dimension of t is time, and m and b are constants. What are the dimensions and SI units of (a) m and (b) b ?
Consider the equation [latex] s={s}_{0}+{v}_{0}t+{a}_{0}{t}^{2}\text{/}2+{j}_{0}{t}^{3}\text{/}6+{S}_{0}{t}^{4}\text{/}24+c{t}^{5}\text{/}120, [/latex] where s is a length and t is a time. What are the dimensions and SI units of (a) [latex] {s}_{0}, [/latex] (b) [latex] {v}_{0}, [/latex] (c) [latex] {a}_{0}, [/latex] (d) [latex] {j}_{0}, [/latex] (e) [latex] {S}_{0}, [/latex] and (f) c ?
a. [latex] [{s}_{0}]=\text{L} [/latex] and units are meters (m); b. [latex] [{v}_{0}]={\text{LT}}^{-1} [/latex] and units are meters per second (m/s); c. [latex] [{a}_{0}]={\text{LT}}^{-2} [/latex] and units are meters per second squared (m/s 2 ); d. [latex] [{j}_{0}]={\text{LT}}^{-3} [/latex] and units are meters per second cubed (m/s 3 ); e. [latex] [{S}_{0}]={\text{LT}}^{-4} [/latex] and units are m/s 4 ; f. [latex] [c]={\text{LT}}^{-5} [/latex] and units are m/s 5 .
(a) A car speedometer has a 5% uncertainty. What is the range of possible speeds when it reads 90 km/h? (b) Convert this range to miles per hour. Note 1 km = 0.6214 mi.
A marathon runner completes a 42.188-km course in 2 h, 30 min, and 12 s. There is an uncertainty of 25 m in the distance traveled and an uncertainty of 1 s in the elapsed time. (a) Calculate the percent uncertainty in the distance. (b) Calculate the percent uncertainty in the elapsed time. (c) What is the average speed in meters per second? (d) What is the uncertainty in the average speed?
a. 0.059%; b. 0.01%; c. 4.681 m/s; d. 0.07%, 0.003 m/s
The sides of a small rectangular box are measured to be 1.80 ± 0.1 cm, 2.05 ± 0.02 cm, and 3.1 ± 0.1 cm long. Calculate its volume and uncertainty in cubic centimeters.
When nonmetric units were used in the United Kingdom, a unit of mass called the pound-mass (lbm) was used, where 1 lbm = 0.4539 kg. (a) If there is an uncertainty of 0.0001 kg in the pound-mass unit, what is its percent uncertainty? (b) Based on that percent uncertainty, what mass in pound-mass has an uncertainty of 1 kg when converted to kilograms?
a. 0.02%; b. 1×10 4 lbm
The length and width of a rectangular room are measured to be 3.955 ± 0.005 m and 3.050 ± 0.005 m. Calculate the area of the room and its uncertainty in square meters.
A car engine moves a piston with a circular cross-section of 7.500 ± 0.002 cm in diameter a distance of 3.250 ± 0.001 cm to compress the gas in the cylinder. (a) By what amount is the gas decreased in volume in cubic centimeters? (b) Find the uncertainty in this volume.
a. 143.6 cm 3 ; b. 0.2 cm 3 or 0.14%
Challenge Problems
The first atomic bomb was detonated on July 16, 1945, at the Trinity test site about 200 mi south of Los Alamos. In 1947, the U.S. government declassified a film reel of the explosion. From this film reel, British physicist G. I. Taylor was able to determine the rate at which the radius of the fireball from the blast grew. Using dimensional analysis, he was then able to deduce the amount of energy released in the explosion, which was a closely guarded secret at the time. Because of this, Taylor did not publish his results until 1950. This problem challenges you to recreate this famous calculation. (a) Using keen physical insight developed from years of experience, Taylor decided the radius r of the fireball should depend only on time since the explosion, t , the density of the air, [latex] \rho , [/latex] and the energy of the initial explosion, E . Thus, he made the educated guess that [latex] r=k{E}^{a}{\rho }^{b}{t}^{c} [/latex] for some dimensionless constant k and some unknown exponents a , b , and c . Given that [E] = ML 2 T –2 , determine the values of the exponents necessary to make this equation dimensionally consistent. ( Hint : Notice the equation implies that [latex] k=r{E}^{\text{−}a}{\rho }^{\text{−}b}{t}^{\text{−}c} [/latex] and that [latex] [k]=1. [/latex]) (b) By analyzing data from high-energy conventional explosives, Taylor found the formula he derived seemed to be valid as long as the constant k had the value 1.03. From the film reel, he was able to determine many values of r and the corresponding values of t . For example, he found that after 25.0 ms, the fireball had a radius of 130.0 m. Use these values, along with an average air density of 1.25 kg/m 3 , to calculate the initial energy release of the Trinity detonation in joules (J). ( Hint : To get energy in joules, you need to make sure all the numbers you substitute in are expressed in terms of SI base units.) (c) The energy released in large explosions is often cited in units of “tons of TNT” (abbreviated “t TNT”), where 1 t TNT is about 4.2 GJ. Convert your answer to (b) into kilotons of TNT (that is, kt TNT). Compare your answer with the quick-and-dirty estimate of 10 kt TNT made by physicist Enrico Fermi shortly after witnessing the explosion from what was thought to be a safe distance. (Reportedly, Fermi made his estimate by dropping some shredded bits of paper right before the remnants of the shock wave hit him and looked to see how far they were carried by it.)
The purpose of this problem is to show the entire concept of dimensional consistency can be summarized by the old saying “You can’t add apples and oranges.” If you have studied power series expansions in a calculus course, you know the standard mathematical functions such as trigonometric functions, logarithms, and exponential functions can be expressed as infinite sums of the form [latex] \sum _{n=0}^{\infty }{a}_{n}{x}^{n}={a}_{0}+{a}_{1}x+{a}_{2}{x}^{2}+{a}_{3}{x}^{3}+\cdots , [/latex] where the [latex] {a}_{n} [/latex] are dimensionless constants for all [latex] n=0,1,2,\cdots [/latex] and x is the argument of the function. (If you have not studied power series in calculus yet, just trust us.) Use this fact to explain why the requirement that all terms in an equation have the same dimensions is sufficient as a definition of dimensional consistency. That is, it actually implies the arguments of standard mathematical functions must be dimensionless, so it is not really necessary to make this latter condition a separate requirement of the definition of dimensional consistency as we have done in this section.
Since each term in the power series involves the argument raised to a different power, the only way that every term in the power series can have the same dimension is if the argument is dimensionless. To see this explicitly, suppose [x] = L a M b T c . Then, [x n ] = [x] n = L an M bn T cn . If we want [x] = [x n ], then an = a, bn = b, and cn = c for all n. The only way this can happen is if a = b = c = 0.
- OpenStax University Physics. Authored by : OpenStax CNX. Located at : https://cnx.org/contents/[email protected]:Gofkr9Oy@15 . License : CC BY: Attribution . License Terms : Download for free at http://cnx.org/contents/[email protected]
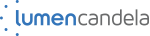
Privacy Policy

IMAGES
VIDEO
COMMENTS
An expert approaches problems differently than a novice. A novice may search for an equation to apply to the problem that matches the given variables, or the novice may try to solve a problem using the solution to a similar problem they have done before.
Although there is no simple step-by-step method that works for every problem, the following three-stage process facilitates problem solving and makes it more meaningful. The three stages are strategy, solution, and significance.
Although there is no simple step-by-step method that works for every problem, the following three-stage process facilitates problem solving and makes it more meaningful. The three stages are strategy, solution, and significance.
Mastering Physics Problem-Solving: A Comprehensive 6-Step Guide. Introduction. Physics problems can often be daunting, but with a systematic approach, they become manageable challenges. In this guide, we will explore a detailed six-step process designed to enhance your problem-solving skills.
1.7 Solving Problems in Physics | University Physics Volume 1. Learning Objectives. By the end of this section, you will be able to: Describe the process for developing a problem-solving strategy. Explain how to find the numerical solution to a problem. Summarize the process for assessing the significance of the numerical solution to a problem.
How To Solve Any Physics Problem: Learn five simple steps in five minutes! In this episode we cover the most effective problem-solving method I’ve encountered and call upon some fuzzy friends to help us remember the steps. Free Body Diagram: Use this figure to work through the example problem.