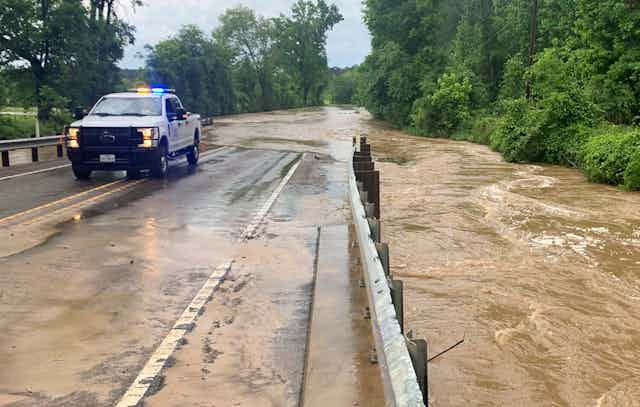

Houston’s flood problems offer lessons for cities trying to adapt to a changing climate
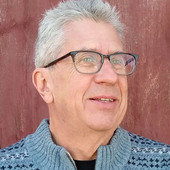
Professor Emeritus of Climate and Space Sciences and Engineering, University of Michigan
Disclosure statement
Richard Rood receives funding from the National Oceanographic and Atmospheric Administration and the National Science Foundation. He is a co-principal investigator at the Great Lakes Integrated Sciences and Assessment Center at the University of Michigan.
University of Michigan provides funding as a founding partner of The Conversation US.
View all partners
Scenes from the Houston area looked like the aftermath of a hurricane in early May 2024 after a series of powerful storms flooded highways and neighborhoods and sent rivers over their banks north of the city.
Hundreds of people had to be rescued from homes, rooftops and cars during storms, according to The Associated Press. Huntsville registered nearly 20 inches of rain from April 29 to May 4. Another storm system in mid-May blew out windows in Houston high rises and caused more flash flooding on urban streets and already saturated ground in the region.
Floods are complex events, and they are about more than just heavy rain. Each community has its own unique geography and climate that can exacerbate flooding. On top of those risks, extreme downpours are becoming more common as global temperatures rise.
I work with a center at the University of Michigan that helps communities turn climate knowledge into projects that can reduce the harm of future climate disasters. Flooding events like the Houston area experienced provide case studies that can help cities everywhere manage the increasing risk.
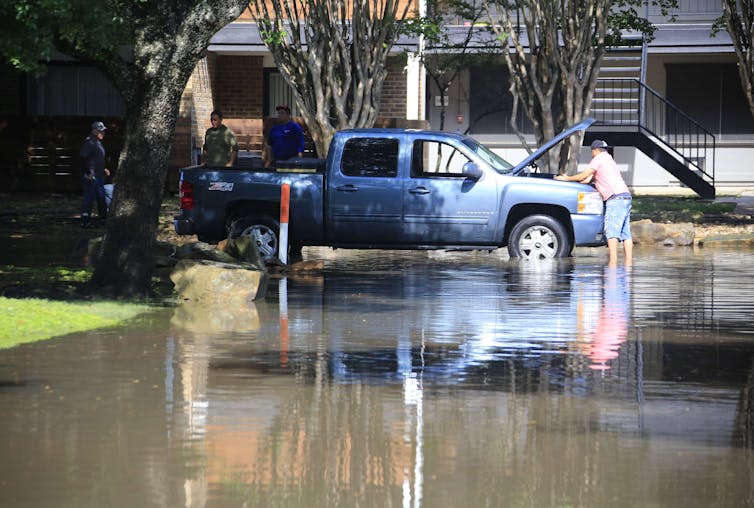
Flood risks are rising
The first thing recent floods tell us is that the climate is changing.
In the past, it might have made sense to consider a flood a rare and random event – communities could just build back. But the statistical distribution of weather events and natural disasters is shifting.
What might have been a 1-in-500-years event may become a 1-in-100-years event , on the way to becoming a 1-in-50-years event. When Hurricane Harvey hit Texas in 2017, it delivered Houston’s third 500-year flood in the span of three years.
Basic physics points to the rising risks: Global greenhouse gas emissions are increasing global average temperatures. Warming leads to increasing precipitation and more intense downpours, and increased flood potential, particularly when storms hit on already saturated ground.
Communities aren’t prepared
Recent floods are also revealing vulnerabilities in how communities are designed and managed.
Pavement is a major contributor to urban flooding, because water cannot be absorbed and it runs off quickly. The Houston area’s frequent flooding illustrates the risks. Its impervious surfaces expanded by 386 square miles (1,000 square kilometers) between 1997 and 2017, according to data collected by Rice University . More streets, parking lots and buildings meant more standing water with fewer places for rainwater to sink in.
If the infrastructure is well designed and maintained, flood damage can be greatly reduced. However, increasingly, researchers have found that the engineering specifications for drainage pipes and other infrastructure are no longer adequate to handle the increasing severity of storms and amounts of precipitation. This can lead to roads being washed out and communities being cut off . Failures in maintaining infrastructure, such as levees and storm drains, are a common contributor to flooding.
In the Houston area, reservoirs are also an essential part of flood management, and many were at capacity from persistent rain. This forced managers to release more water when the storms hit.
For a coastal metropolis such as the Houston-Galveston area, rapidly rising sea levels can also reduce the downstream capacity to manage water. These different factors compound to increase flooding risk and highlight the need to not only move water but to find safe places to store it.
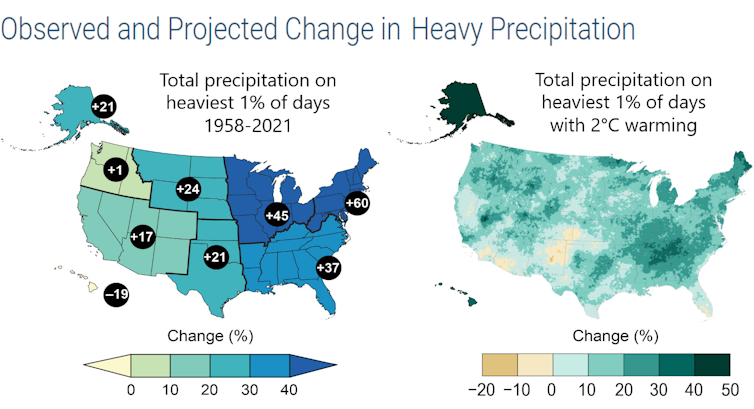
The increasing risks affect not only engineering standards, but zoning laws that govern where homes can be built and building codes that describe minimum standards for safety, as well as permitting and environmental regulations.
By addressing these issues now, communities can anticipate and avoid damage rather than only reacting when it’s too late.
Four lessons from case studies
The many effects associated with flooding show why a holistic approach to planning for climate change is necessary, and what communities can learn from one another. For example, case studies show that:
Floods can damage resources that are essential in flood recovery, such as roads, bridges and hospitals . Considering future risks when determining where and how to build these resources enhances the ability to recover from future disasters . Jackson, Mississippi’s water treatment plant was knocked offline by flooding in 2022, leaving people without safe running water. Houston’s Texas Medical Center famously prepared to manage future flooding by installing floodgates, elevating backup generators and taking other steps after heavy damage during Tropical Storm Allison in 2001.
Flood damage does not occur in isolation. Downpours can trigger mudslides , make sewers more vulnerable and turn manufacturing facilities into toxic contamination risks . These can become broad-scale dangers, extending far beyond individual communities.
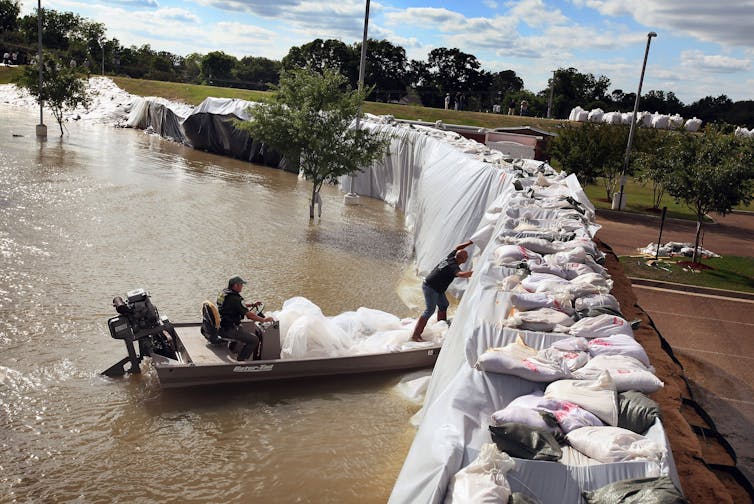
It is difficult for an individual or a community to take on even the technical aspects of flood preparation alone – there is too much interconnectedness. Protective measures like levees or channels might protect one neighborhood but worsen the flood risk downstream . Planners should identify the appropriate regional scale, such as the entire drainage basin of a creek or river, and form important relationships early in the planning process.
Natural disasters and the ways communities respond to them can also amplify disparities in wealth and resources. Social justice and ethical considerations need to be brought into planning at the beginning.
Learning to manage complexity
In communities that my colleagues and I have worked with , we have found an increasing awareness of the challenges of climate change and rising flood risks.
In most cases, local officials’ initial instinct has been to protect property and persist without changing where people live. However, that might only buy time for some areas before people will have little option but to move .
When they examine their vulnerabilities, many of these communities have started to recognize the interconnectedness of zoning, storm drains and parks that can absorb runoff, for example. They also begin to see the importance of engaging regional stakeholders to avoid fragmented efforts to adapt that could worsen conditions for neighboring areas.
This is an updated version of an article originally published Aug. 25, 2022 .
- Climate change
- Infrastructure
- Extreme weather
- Extreme rainfall
- Disaster mitigation
- Flash flooding

Compliance Lead

Lecturer / Senior Lecturer - Marketing

Assistant Editor - 1 year cadetship

Executive Dean, Faculty of Health

Lecturer/Senior Lecturer, Earth System Science (School of Science)
- 2023 Lancet Countdown U.S. Launch Event
- 2023 Lancet Countdown U.S. Brief
- PAST BRIEFS
2020 CASE STUDY 2
The 2019 floods in the central u.s..
Lessons for Improving Health, Health Equity, and Resiliency
In spring 2019, the Midwest region endured historic flooding that caused widespread damage to millions of acres of farmland, killing livestock, inundating cities, and destroying infrastructure. CS_52
The Missouri River and North Central Flood resulted in over $10.9 billion of economic loss in the region, making it the costliest inland flood event in U.S. history. CS_52 Yet, this is just the beginning, as climate change continues to accelerate extreme precipitation, increasing the likelihood of severe events previously thought of as “once in 100 year floods.” CS_53 , CS_54
This 2019 disaster exhibited the same health harms and healthcare system disruptions seen in previous flooding events, and vulnerable populations – notably tribal and Indigenous communities – were once again disproportionately impacted. Thus, there is an enormous need for policy interventions to minimize health harms, improve health equity, and ensure community resilience as the frequency of these weather events increases.
Before-and-after images of catastrophic flooding in Nebraska. Left image taken March 20, 2018. Right image taken March 16, 2019.

Source: NASA Goddard Space Flight Center, with permission
The role of climate change, widespread devastation, and compounding inequities
The Missouri River and North Central Flood were the result of a powerful storm that occurred near the end of the wettest 12-month period on record in the U.S. (May 2018 – May 2019). CS_55 , CS_56 The storm struck numerous states, specifically Nebraska (see Figure 1), Iowa, Missouri, South Dakota, North Dakota, Minnesota, Wisconsin, and Michigan. Two additional severe flooding events occurred in 2019 in states further south, involving the Mississippi and Arkansas Rivers.
This flood event exhibits two key phenomena that have been observed over the last 50 years as a result of climate change: annual rainfall rates and extreme precipitation have increased across the country. CS_57 The greatest increases have been seen in the Midwest and Northeast, and these trends are expected to continue over the next century. Future climate projections also indicate that winter precipitation will increase over this region, CS_57 further increasing the likelihood of more frequent and more severe floods. For example, by mid-century the intensity of extreme precipitation events could increase by 40% across southern Wisconsin. CS_58 While it is too early to have detection and attribution studies for these floods, climate change has been linked to previous extreme precipitation and flood events. CS_59 , CS_60
Hundreds of people were displaced from their homes and millions of acres of agricultural land were inundated with floodwaters, killing thousands of livestock and preventing crop planting. CS_52 , CS_61 , CS_62 Federal Emergency Management Agency (FEMA) disaster declarations were made throughout the region, allowing individuals to apply for financial and housing assistance, though remaining at the same housing site continues to place them at risk of future flood events.
In Nebraska alone, 104 cities, 81 counties and 5 tribal nations received state or federal disaster declarations. FEMA approved over 3,000 individual assistance applications in Nebraska, with more than $27 million approved in FEMA Individual and Household Program dollars. In addition to personal property, infrastructure was heavily affected, with multiple bridges, dams, levees, and roads sustaining major damage (see Figure 2). CS_52
Destruction of Spencer Dam during Missouri River and North Central Floods. CS_63

- Oglala Sioux Tribe, Cheyenne River Sioux Tribe of the Cheyenne River Reservation, Standing Rock Sioux Tribe (North Dakota and South Dakota), Yankton Sioux Tribe of South Dakota, Lower Brule Sioux Tribe of the Lower Brule Reservation, Crow Creek Sioux Tribe of Crow Creek Reservation, Sisseton-Wahpeton Oyate of the Lake Traverse Reservation, Rosebud Sioux Tribe of the Rosebud Sioux Indian Reservation, Santee Sioux Nation, Omaha Tribe of Nebraska, Winnebago Tribe of Nebraska, Ponca Tribe of Nebraska, Sac & Fox Nation of Missouri (Kansas and Nebraska), Iowa Tribe of Kansas and Nebraska, and Sac & Fox Tribe of the Mississippi in Iowa.
Source: Nebraska Department of Natural Resources, with permission.
As with other climate-related disasters, the 2019 floods had devastating effects on already vulnerable communities as numerous tribes and Indigenous peoples were impacted,° adding to centuries of historical trauma. CS_64 , CS_65 Accounts of flooding on the Pine Ridge Reservation in South Dakota demonstrate the challenges that resource-limited communities face in coping with extreme weather events. CS_64 Delayed response by outside emergency services left tribal volunteers struggling to help residents stranded across large distances without access to supplies, drinking water, or medical care.66 Lack of equipment and limited transportation hampered evacuations. CS_67
Health harms and healthcare disruptions
There were three recorded deaths from drowning, but hidden health impacts were widespread and extended well beyond the immediate risks and injuries from floodwaters. In the aftermath, individuals in flooded areas were exposed to hazards like chemicals, electrical shocks, and debris. CS_68 Water, an essential foundation for health, was contaminated as towns’ wells and other drinking water sources were compromised. This put people, especially children, at increased risk for health harms like gastrointestinal illnesses. CS_69 Stranded residents relied on shipments of water from emergency services and volunteer organizations and the kindness of strangers ( see Box 1 ).
BOX 1: “We just remember the trust and commitment to each other”
Linda Emanuel, a registered nurse and farmer living in the hard-hit rural area of North Bend in Nebraska, helped organize flood recovery efforts. She recalled wondering, “How are we going to handle this? How do we inform the people of all the hazards without scaring them?” In addition to her educational role, she administered a limited supply of tetanus shots, obtained and distributed hard-to-find water testing kits, and coordinated PPE usage. In the first days of the flooding, she hosted some 25 stranded individuals in her home. Reminiscing about how community members came together amidst the devastation, Emanuel remarked, “We just remember the trust and the commitment to each other and to our town. We are definitely a resilient city.” CS_70
Standing water remained in many small town for months, and a four-year old child at the Yankton Sioux reservation in South Dakota likely contracted Methicillin-resistant Staphylococcus aureus (MRSA) after playing in a pond. CS_71 The mold and allergens that developed in the aftermath of the floods exacerbated respiratory illness. CS_72 Flooding also backed up sewer systems into basements; clean up required personal protective equipment (PPE) to prevent the potential spread of infectious diseases. The significant financial burdens, notably the loss of property in the absence of adequate insurance, can contribute to serious mental and emotional distress in flood victims. CS_73 , CS_74
Infrastructure disruptions, like flooded roads, meant that many individuals in rural areas were unable to access essential services including healthcare. In an interview with the New York Times, Ella Red Cloud-Yellow Horse, 59, from Pine Ridge Indian Reservation, recounts her own struggle to get to the hospital for a chemotherapy appointment. CS_64 After being stranded by flooding for days, she had contracted pneumonia, but she couldn’t be reached by an ambulance or tractor because her driveway was blocked by huge amounts of mud. She was forced to trudge through muddy flood waters for over an hour to get to the highway.
She told the Times, “I couldn’t breathe, but I knew I needed to get to the hospital.” Her story is an increasingly common occurrence as critical infrastructure is damaged by climate change-intensified extreme events. These infrastructure challenges are also often superimposed on top of the challenges of poverty and disproportionate rates of chronic diseases ( see the Case Study ). Multiple hospitals sustained damage and several long-term care facilities were forced to evacuate, with some closing permanently, as a result of the rising floodwaters, CS_75 likely exacerbating existing diseases.
A path towards a healthier, equitable, and more resilient future
As human-caused climate change increases the likelihood of precipitation events that can cause severe flooding disasters, public health systems must serve as a first line of defense against the resulting health harms. As such, the broader public health system needs to develop the capacity and capability to understand and address the health hazards associated with climate-related disasters. Often funds and resources for these efforts are focused on coastal communities; however, inland states face many climate-related hazards that are regularly overlooked. Building on or expanding programs similar to CDC’s Climate-Ready States and Cities Initiative will help communities in inland states prepare for future climate threats. CS_76
Additionally, public health officials, health systems, and climate scientists should collaborate to create robust early warning systems to help individuals and communities prepare for flood events. Education regarding the health impacts of flooding should not be limited to the communities affected, but it should also include policymakers and other stakeholders who can implement systemic changes to decrease and mitigate the effects of floods. Local knowledge offered by community members regarding water systems, weather patterns, and infrastructure will be essential for effective and context-specific adaptation. By implementing these changes and executing more inclusive flood emergency plans, communities will be better situated to face the flood events that are projected to increase in the years to come.
Introduction – Figure 1: Nebraska Flooding The Role of Climate Change – Figure 2: Destruction of Spencer Dam Health Harms and Healthcare Disruptions – Box 1: Remember the Trust A Path Towards Equality
University of Minnesota Duluth
University digital conservancy, encroaching tides: how sea level rise and tidal flooding threaten u.s. east and gulf coast communities over the next 30 years, view/download file, persistent link to this item, journal title, journal issn, volume title, published date, description, collections, series/report number, funding information, isbn identifier, doi identifier, previously published citation, suggested citation.
Content distributed via the University Digital Conservancy may be subject to additional license and use restrictions applied by the depositor. By using these files, users agree to the Terms of Use . Materials in the UDC may contain content that is disturbing and/or harmful. For more information, please see our statement on harmful content in digital repositories .
- About the New York Fed
- Bank Leadership
- Diversity and Inclusion
- Communities We Serve
- Board of Directors
- Disclosures
- Ethics and Conflicts of Interest
- Annual Financial Statements
- News & Events
- Advisory Groups
- Vendor Information
- Holiday Schedule
At the New York Fed, our mission is to make the U.S. economy stronger and the financial system more stable for all segments of society. We do this by executing monetary policy, providing financial services, supervising banks and conducting research and providing expertise on issues that impact the nation and communities we serve.
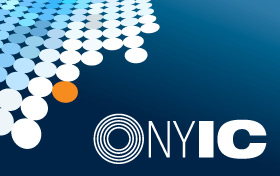
The New York Innovation Center bridges the worlds of finance, technology, and innovation and generates insights into high-value central bank-related opportunities.
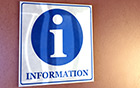
Do you have a request for information and records? Learn how to submit it.
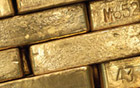
Learn about the history of the New York Fed and central banking in the United States through articles, speeches, photos and video.
- Markets & Policy Implementation
- Reference Rates
- Effective Federal Funds Rate
- Overnight Bank Funding Rate
- Secured Overnight Financing Rate
- SOFR Averages & Index
- Broad General Collateral Rate
- Tri-Party General Collateral Rate
- Desk Operations
- Treasury Securities
- Agency Mortgage-Backed Securities
- Reverse Repos
- Securities Lending
- Central Bank Liquidity Swaps
- System Open Market Account Holdings
- Primary Dealer Statistics
- Historical Transaction Data
- Monetary Policy Implementation
- Agency Commercial Mortgage-Backed Securities
- Agency Debt Securities
- Repos & Reverse Repos
- Discount Window
- Treasury Debt Auctions & Buybacks as Fiscal Agent
- INTERNATIONAL MARKET OPERATIONS
- Foreign Exchange
- Foreign Reserves Management
- Central Bank Swap Arrangements
- Statements & Operating Policies
- Survey of Primary Dealers
- Survey of Market Participants
- Annual Reports
- Primary Dealers
- Standing Repo Facility Counterparties
- Reverse Repo Counterparties
- Foreign Exchange Counterparties
- Foreign Reserves Management Counterparties
- Operational Readiness
- Central Bank & International Account Services
- Programs Archive
- Economic Research
- Consumer Expectations & Behavior
- Survey of Consumer Expectations
- Household Debt & Credit Report
- Home Price Changes
- Growth & Inflation
- Equitable Growth Indicators
- Multivariate Core Trend Inflation
- New York Fed DSGE Model
- New York Fed Staff Nowcast
- R-star: Natural Rate of Interest
- Labor Market
- Labor Market for Recent College Graduates
- Financial Stability
- Corporate Bond Market Distress Index
- Outlook-at-Risk
- Treasury Term Premia
- Yield Curve as a Leading Indicator
- Banking Research Data Sets
- Quarterly Trends for Consolidated U.S. Banking Organizations
- Empire State Manufacturing Survey
- Business Leaders Survey
- Supplemental Survey Report
- Regional Employment Trends
- Early Benchmarked Employment Data
- INTERNATIONAL ECONOMY
- Global Supply Chain Pressure Index
- Staff Economists
- Visiting Scholars
- Resident Scholars
- PUBLICATIONS
- Liberty Street Economics
- Staff Reports
- Economic Policy Review
- RESEARCH CENTERS
- Applied Macroeconomics & Econometrics Center (AMEC)
- Center for Microeconomic Data (CMD)
- Economic Indicators Calendar
- Financial Institution Supervision
- Regulations
- Reporting Forms
- Correspondence
- Bank Applications
- Community Reinvestment Act Exams
- Frauds and Scams
As part of our core mission, we supervise and regulate financial institutions in the Second District. Our primary objective is to maintain a safe and competitive U.S. and global banking system.
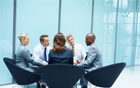
The Governance & Culture Reform hub is designed to foster discussion about corporate governance and the reform of culture and behavior in the financial services industry.
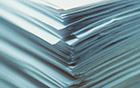
Need to file a report with the New York Fed? Here are all of the forms, instructions and other information related to regulatory and statistical reporting in one spot.
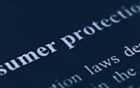
The New York Fed works to protect consumers as well as provides information and resources on how to avoid and report specific scams.
- Financial Services & Infrastructure
- Services For Financial Institutions
- Payment Services
- Payment System Oversight
- International Services, Seminars & Training
- Tri-Party Repo Infrastructure Reform
- Managing Foreign Exchange
- Money Market Funds
- Over-The-Counter Derivatives
The Federal Reserve Bank of New York works to promote sound and well-functioning financial systems and markets through its provision of industry and payment services, advancement of infrastructure reform in key markets and training and educational support to international institutions.
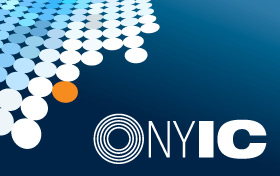
The New York Fed offers the Central Banking Seminar and several specialized courses for central bankers and financial supervisors.
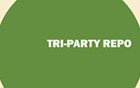
The New York Fed has been working with tri-party repo market participants to make changes to improve the resiliency of the market to financial stress.
- Community Development & Education
- Household Financial Well-being
- Fed Communities
- Fed Listens
- Fed Small Business
- Workforce Development
- Other Community Development Work
- High School Fed Challenge
- College Fed Challenge
- Teacher Professional Development
- Classroom Visits
- Museum & Learning Center Visits
- Educational Comic Books
- Economist Spotlight Series
- Lesson Plans and Resources
- Economic Education Calendar
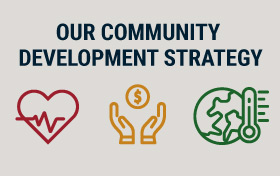
We are connecting emerging solutions with funding in three areas—health, household financial stability, and climate—to improve life for underserved communities. Learn more by reading our strategy.
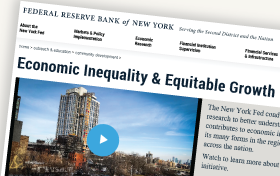
The Economic Inequality & Equitable Growth hub is a collection of research, analysis and convenings to help better understand economic inequality.
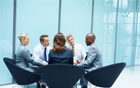
Case Study Finds New Yorkers Who Experienced Floods Felt Financially Unprepared
The Federal Reserve Bank of New York today released a white paper about the impact of flooding on low- and moderate-income households in New York City. “ Flooding Impacts on Household Finances: Insights from Focus Groups in New York City ,” draws from focus groups the New York Fed’s Community Development team conducted in 2023 with 31 New York City residents whose homes have flooded.
The report found that most renters reported having no renters’ insurance to cover the cost of lost clothes, furniture, or equipment. No focus group participants reported receiving payment from renters’ insurance after experiencing flooding. Additionally, no participants reported being offered or receiving public disaster assistance following a flood.
The New York Fed’s Community Development team conducted the focus groups to help improve estimates of typical financial impact of flooding, including property loss and repairs, lost labor hours, and health impacts. Separate New York Fed research has found that approximately one in ten low- to moderate-income people, immigrants, and racial and ethnic minorities in New York City live in a flood-prone census tract.
The white paper found that the most consequential flooding effects were on housing safety and stability. In addition to water damage and lost possessions, worsened mental and physical health are key consequences of flooding. The report found that recovery in the aftermath of flooding can be costly, isolating, and time consuming.
Among the other key findings:
- Economic barriers, including housing affordability, prevent some residents from moving to higher ground. Positive community connections also keep residents in their neighborhoods despite higher flood risk.
- Residents reported that they were highly dependent on friends, family, volunteers, and local nonprofit organizations for aid and assistance after flooding.
- Residents who lived alone or lacked strong social ties to their community reported a general sense of loneliness following a flood. They said they had trouble finding assistance and navigating an opaque relief structure on their own.
“The people we spoke with reported everything from depression to homelessness following a flood,” said Dyvonne Body, the report’s author. “The future financial supports they told us would protect others in flood-prone communities include adequate insurance, emergency grant assistance to replace lost income, and a grace period for debt repayment.”
The case study was developed as part of the New York Fed's Community Development efforts, which have three areas of focus: health , household financial well-being , and climate risk .

- Request a Speaker
- International Seminars & Training
- Governance & Culture Reform
- Data Visualization
- Economic Research Tracker
- Markets Data APIs
- Terms of Use
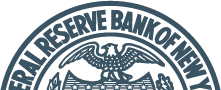
- Research article
- Open access
- Published: 07 May 2024
Floods and cause-specific mortality in the UK: a nested case-control study
- Danijela Gasevic 1 ,
- Zhengyu Yang 1 ,
- Guowei Zhou 2 ,
- Yan Zhang 2 ,
- Jiangning Song 3 ,
- Hong Liu 2 na1 ,
- Shanshan Li 2 na1 &
- Yuming Guo ORCID: orcid.org/0000-0002-1766-6592 2 na1
BMC Medicine volume 22 , Article number: 188 ( 2024 ) Cite this article
511 Accesses
18 Altmetric
Metrics details
Floods are the most frequent weather-related disaster, causing significant health impacts worldwide. Limited studies have examined the long-term consequences of flooding exposure.
Flood data were retrieved from the Dartmouth Flood Observatory and linked with health data from 499,487 UK Biobank participants. To calculate the annual cumulative flooding exposure, we multiplied the duration and severity of each flood event and then summed these values for each year. We conducted a nested case-control analysis to evaluate the long-term effect of flooding exposure on all-cause and cause-specific mortality. Each case was matched with eight controls. Flooding exposure was modelled using a distributed lag non-linear model to capture its nonlinear and lagged effects.
The risk of all-cause mortality increased by 6.7% (odds ratio (OR): 1.067, 95% confidence interval (CI): 1.063–1.071) for every unit increase in flood index after confounders had been controlled for. The mortality risk from neurological and mental diseases was negligible in the current year, but strongest in the lag years 3 and 4. By contrast, the risk of mortality from suicide was the strongest in the current year (OR: 1.018, 95% CI: 1.008–1.028), and attenuated to lag year 5. Participants with higher levels of education and household income had a higher estimated risk of death from most causes whereas the risk of suicide-related mortality was higher among participants who were obese, had lower household income, engaged in less physical activity, were non-moderate alcohol consumers, and those living in more deprived areas.
Conclusions
Long-term exposure to floods is associated with an increased risk of mortality. The health consequences of flooding exposure would vary across different periods after the event, with different profiles of vulnerable populations identified for different causes of death. These findings contribute to a better understanding of the long-term impacts of flooding exposure.
Peer Review reports
Floods are the most frequent type of weather-related disaster, accounting for about 47% of all weather-related disasters from 1995 to 2015 [ 1 , 2 ]. Between 1995 and 2015, more than 2.3 billion people were affected by flood disasters, with over 157 thousand people dying directly as a result of floods [ 3 ]. In recent years, many intense urban flooding events have been recorded in the UK, resulting in loss of lives, damages to personal property and public health infrastructure, and disruption to vital services such as water, communications, energy, and public transport [ 4 , 5 , 6 , 7 , 8 , 9 ]. Approximately 1.9 million people across the UK are at risk of floods, and this number will double as early as the 2050s [ 10 ].
In addition to immediate fatalities due to drowning and acute trauma [ 11 ], floods can also cause short- (lasting days or weeks) or medium-health impacts (several weeks or months), including the spread of water- and vector-borne diseases, such as cholera, typhoid, or malaria; injuries during evacuations and disaster clean-up; and exposure to chemical hazards [ 1 , 12 ]. Non-communicable diseases (e.g. cardiovascular disease, neoplasms, chronic respiratory diseases, and diabetes) which need prolonged treatment and care can be exacerbated after floods due to a disruption in care, treatment, medication, supplies, equipment, and overcrowding in shelters [ 13 , 14 , 15 , 16 , 17 , 18 ]. Mental health issues may arise from stressors caused by floods (e.g. property damage, financial loss, loss of a loved one) and have long-lasting health effects on mortality and morbidity. These long-term health consequences may arise from several pathways, including impairment of the immune system, sleep disturbances, substance abuse, and inadequate self-care [ 19 , 20 , 21 , 22 ].
Despite the severe impacts of floods, there currently is limited epidemiological evidence on the long-term mortality impacts of exposure to floods. To address these gaps in knowledge, we utilized the UK Biobank project, a population-based study with a large sample size, to explore the long-term effects of flooding on mortality. We aimed to estimate the risk of all-cause and seven cause-specific mortality associated with floods and to explore the lag patterns in mortality risk. We also conducted subgroup analyses to identify populations who are potentially more vulnerable to flood-related death.
Study design and study population
We conducted a nested case-control study within a cohort of participants registered with the UK Biobank study. About 0.5 million residents aged between 37 and 73 years were enrolled in the UK Biobank from 2006 to 2010, from 21 assessment centres across England, Wales, and Scotland. The cohort was followed up until the date of death or the study end date (December 31, 2020). We excluded participants lacking longitude and latitude data of residence ( n = 11), participants with missing data on age, sex, and ethnicity ( n = 2775), and those who died in the year of recruitment ( n = 141). A total of 499,487 participants were included (Fig 1 ). All participants in the UK Biobank study provided informed consent. The utilization of the data presented in this paper has been approved by the UK Biobank access committee under UK Biobank application number 55257.
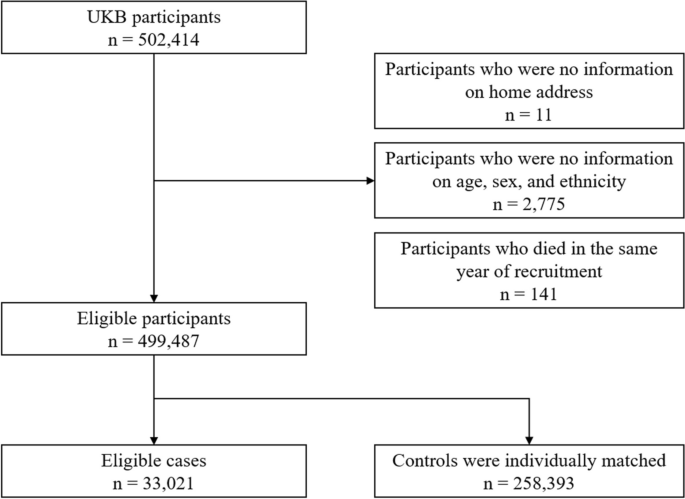
A flow diagram to show participants whose data were used to estimate the association between flooding exposure and mortality
Case-control selection
With the nested case-control design, we matched controls to cases with replacement at the time of the outcome event and assessed exposure retrospectively, from the date of death or end of follow-up. This ensures identical exposure lengths across participants. Using a risk-set sampling method, each case was matched with eight controls randomly selected from study participants who met the matching criteria for age (within 5 years), sex (male and female), and ethnicity (White, Black, Asian or Asian British, mixed, Chinese, and others). The index date for cases corresponded to the date of death; while for controls, it was the date of death of the matched case participant. For twelve case-control sets, eligible controls were less than eight but at least one (Fig 1 ).
Participants were eligible for inclusion as cases for the study if they died during the study period. We defined all-cause mortality and seven cause-specific mortality categories using the International Classification of Diseases, edition 10 (ICD-10), classification as follows: neoplasms, C00–D48; cardiovascular disease, I00–I99; respiratory diseases, J09–J98; digestive disease, K20–K93; neurodegenerative disease, F01–03, G122, G20, G21, G23, G30, G31; mental and behavioural disorders: F00–F90; and suicide: X60–X84, Y10–Y34, Y87.
Flooding exposure
We collected flood data during 2000–2020 from the Dartmouth Flood Observatory (DFO), which is a global catalogue of all flood events with detailed information on start date, end date, centroids, impacted geographic areas, and severities. All documented flood events were sourced from news, government, and instrumental sources and have been validated by satellite observations [ 23 , 24 ]. Participants whose home addresses fall within flood-affected areas were considered as having been exposed to a flood event. To assess the long-term effect of floods, we calculated a cumulative exposure during the study period for each participant. Building on previous research [ 25 , 26 ], we derived the annual cumulative exposure by multiplying the duration and severity of each flood event and summing these values for each year. Our preliminary analyses suggested a weak negative association between flood severity and duration (Pearson coefficient: − 0.03). The severity of each flood event documented in the DFO was classified based on a pre-defined scale, detailed in Additional file 1 : Table S1. For each participant, annual cumulative flooding exposure was calculated using equation ( 1 ):
where \(\mathrm{Flood}\;{\mathrm{index}}_{i,\text{year}=m}\) stands for the cumulative flooding exposure in year \(m\) for participants \(i\) . \({{\text{Duration}}}_{ij}\) and \({{\text{Severity}}}_{ij}\) represent the duration (day) and the severity of the \(j\) th flood event that participant \(i\) experienced in year \(m\) , respectively. If there were no flood events within a given year, a flood index of 0 was recorded.
Meteorological data
We extracted hourly temperature and relative humidity data from the European Centre for Medium-Range Weather Forecasts Reanalysis v5 (ERA-5) reanalysis data set with a spatial resolution of 0.1°×0.1°. We mapped meteorological data to the participant’s geocoded residential address at baseline. Daily meteorological data were calculated by averaging hourly data within each day. Daily temperature and relative humidity were then aggregated into yearly averages.
Baseline data collected by the UK Biobank include demographics, lifestyle factors, socioeconomic status, and anthropometric measurements. We included additional covariates informed by existing literature, beyond those used for matching cases and controls [ 27 , 28 , 29 ]: body mass index (BMI), physical activity, healthy diet score, cigarette smoking, alcohol consumption, educational attainment, average total annual household income before tax, Townsend deprivation index (TDI), overall health rating, and assessment centres. BMI was calculated from objectively measured weight and height as weight over height squared and expressed as kg/m 2 . Physical activity was derived from the International Physical Activity Questionnaire-Short Form (IPAQ-SF) [ 30 ]. Participants were categorized at ‘high’ (≥ 1500 metabolic equivalent (MET)-minutes/week), ‘moderate’ (≥ 600 MET-minutes/week), or ‘low’ levels of physical activity following standardized IPAQ-SF scoring guidance [ 30 ]. Diet score was calculated based on the following dietary factors: vegetable intake ≥ 3 servings/day; fruit intake ≥ 3 servings /day; whole grains ≥ 3 servings/day; refined grains ≤ 1.5 servings/day; fish intake ≥ 2 servings/day; unprocessed red meat intake ≤ 2 servings/week; and processed meat intake ≤ 2 servings/week. Each point was given for each favourable dietary factor, and the suboptimal diet was defined as a diet score < 4. Smoking status was coded into three categories: current, former, and never. Low-risk alcohol consumption was defined as moderate drinking (no more than one drink/day for women and two drinks/day for men; one drink is measured as 8 g ethanol in the UK) on a relatively regular frequency [ 31 ]. Educational attainment was coded in two categories: ‘high’ (college or university degree) or ‘low’ (A/AS levels or equivalent, O levels/GCSEs or equivalent, or none of the above). Annual household income was classified into two groups (< £31,000 and ≥ £31,000). TDI was utilized to define area deprivation level, with participants being classified as either high (TDI above the median) or low [ 32 ]. Self-reported health was categorized as poor, fair, good, and excellent [ 27 ].
Statistical analysis
We performed conditional logistic regression analysis to estimate the risk of mortality associated with per unit increase in flood index. Year-specific flood index was modelled using a distributed lag non-linear model featuring a non-linear exposure-response association and the additional lag-response association, respectively [ 33 , 34 , 35 , 36 ]. The lag-response association refers to how the risk changes over time and provides an estimation of the combined immediate and delayed effects that accumulate throughout the lag period. We first modelled the exposure-response curve with a natural cubic spline with three degrees of freedom. However, the nonlinear analysis indicated an approximately linear relationship (Additional file 1 : Fig. S1). Further, both the Akaike Information Criterion (AIC) and the Bayesian Information Criterion (BIC) favoured the linear model (Additional file 1 : Table S2). Therefore, we applied a linear exposure-response relationship in the formal analysis. The lag-response curve was modelled with a natural cubic spline with three degrees of freedom plus an intercept. The exposure window comprised the 0 to 5 years before the index date. A maximum lag of 5 years was used because the flood-related mortality risk declined to zero by the lag year 5.
Estimates of risk were obtained from the crude model that only included flood (model 1); the multivariate model that additionally controlled for socioeconomic status (education attainment, household income, and deprivation) (model 2); and the full model that additionally adjusted for BMI, physical activity, smoking, alcohol consumption, suboptimal diet, overall health rating, mean temperature, mean relative humidity, and assessment centre which serves as an indicator of the recruitment location for each participant (model 3). All variance inflator factors were less than 1.5, indicating no multicollinearity. Temperature and relative humidity terms were defined as the average annual mean temperature and relative humidity over 6 years (lag 0–5 years) preceding the index date, respectively. Given that the crude model (model 1) did not include any covariates, all participants were retained in the analysis. For models 2 and 3, we excluded participants with any missing data. In sensitivity analyses, we employed multiple imputation to address missing covariate data and assess the robustness of our findings.
We further identified subgroups vulnerable to floods through stratification analyses by age group (≤64 and >65 years), sex, weight status defined according to BMI (≤ 24.9, 25–29.9, ≥ 30), education attainment, household income, physical activity, suboptimal diet, alcohol consumption status, smoking status, and area deprivation level. Results are presented as odds ratios (ORs) and their 95% confidence intervals (95% CIs) per unit increase in flood index. The significance of the difference in results between subgroups was tested using a random-effect meta-regression model.
Sensitivity analysis
We carried out the following sensitivity analyses: (1) Multiple imputation by chained equations was used for the missing values. Five imputed data sets were created, and their results were combined using Rubin’s rules [ 37 ]. (2) Alternative degrees of freedom were used for the lag-response association of flood. (3) Alternative degrees of freedom were used for the non-linear exposure-response relationship of mean temperature and relative humidity. (4) Alternative matching ratios (1:4 and 1:6) were used. (5) Excluding data after 2020 to control for the effect of the COVID-19 pandemic. (6) To capture the variation in flooding impacts within the year preceding mortality, we performed additional analyses with monthly flood index.
Table 1 shows the baseline characteristics of the 33,021 death cases and the 258,393 matched controls. The mean age (± standard deviation (SD)) of participants at study entry was 61.3 (± 6.4) years; 170,549 (58.5%) were male; 281,175 (96.5%) were white. Participants who died were more likely to have a higher BMI and lower household income; were less likely to be university graduates; more likely to smoke; and consumed less fruit and vegetables and more red and processed meat. They were also more likely to rate their overall health as poor and fair. Baseline characteristics of cases and controls with any missing values in covariates are shown in Additional file 1 : Table S3.
The distributions of the flood index and meteorological factors are shown in Table 2 . The annual average flood index across all participants during the study period ranged from 0.0 to 38.3, with a median value of 1.8 (25 th to 75 th percentiles: 0.5 to 3.6). Cases exposed to higher levels of flooding than controls during the 6 years before the end of follow-up (Additional file 1 : Fig. S2). The median annual mean temperature was 10.0 °C (25 th to 75 th percentiles: 9.3°C to 10.7°C) (Table 2 ). The flood index was negatively correlated with mean temperature (Pearson r = − 0.04) but positively correlated with relative humidity (Pearson r = 0.08).
Figure 2 illustrates the estimated cumulative OR of all-cause and cause-specific mortality associated with per unit increase in flood index over lag years 0–5. Per unit increase in flood index was associated with a 9.2% increased risk of all-cause mortality (OR: 1.092, 95% CI: 1.090–1.093) in the crude model. The results remained similar after further adjustment for socio-economic status (OR: 1.090, 95% CI: 1.088–1.091), whereas adjustment for lifestyle factors decreased the strength of the association (OR for fully adjusted model: 1.067, 95% CI: 1.063–1.071). Similar effects were observed for cause-specific mortality after fully adjusting the models, whereby a greater flood index was associated with a greater risk of death from neurodegenerative diseases (OR: 1.068, 95% CI: 1.050–1.087), neoplasm (OR: 1.063, 95% CI: 1.058–1.068), respiratory diseases (OR: 1.062, 95% CI: 1.045–1.080), suicide (OR: 1.052, 95% CI: 1.018–1.088), cardiovascular diseases (OR: 1.051, 95% CI: 1.042–1.059), mental diseases (OR: 1.047, 95% CI: 1.008–1.087), and digestive diseases (OR: 1.031, 95% CI: 1.011–1.052) (Fig. 2, Additional file 1 : Table S4).
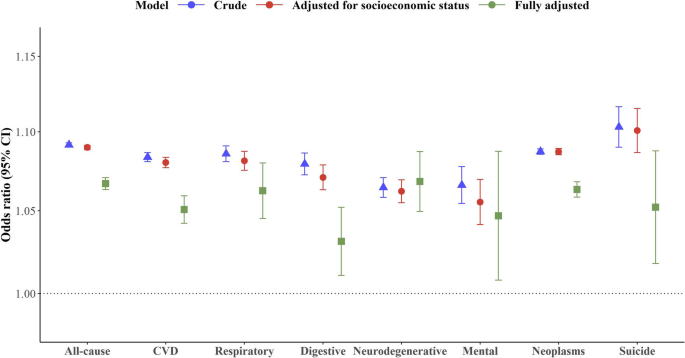
Cumulative odds ratio of all-cause and cause-specific mortality associated with per unit increase in flood index over lag years 0–5. Estimates of risk were obtained from the crude model that only included flood (crude); the multivariate model that additionally controlled for socioeconomic status (education attainment, household income, and deprivation); and the full model that additionally adjusted for BMI, physical activity, smoking, alcohol consumption, suboptimal diet, overall health rating, mean temperature, mean relative humidity, and assessment centre which serves as an indicator of the recruitment location for each participant (fully adjusted). The error bars represent 95% confidence intervals
Figure 3 shows the lag structure in the effects of flooding exposure on all-cause and cause-specific mortality. For all-cause mortality, the magnitude of associations increased from the current year (OR: 1.012, 95% CI: 1.011–1.013) to the lag year 3 (OR: 1.016, 95% CI: 1.015–1.017), and subsequently diminished to zero by lag year 5. For neurodegenerative mortality and mortality due to mental-ill health, the mortality risk was negligible in the current year, but strongest in the lag years 3 and 4. By contrast, the risk of mortality from suicide was the strongest in the current year (OR: 1.018, 95% CI: 1.008–1.028), and attenuated to lag year 5.
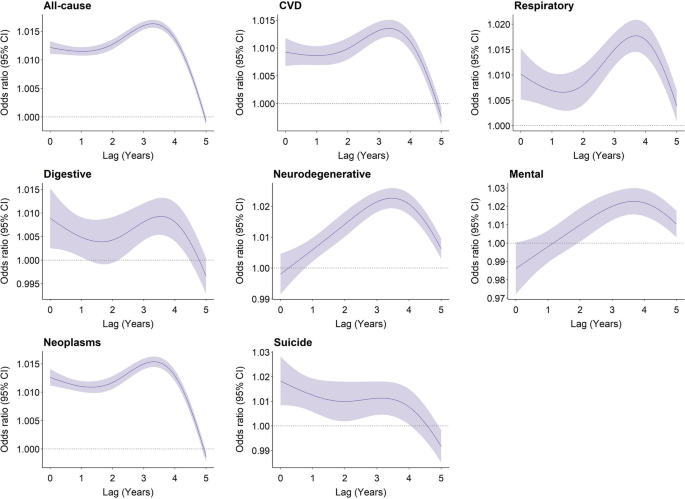
Overall lag structure in effects of flooding exposure on cause-specific mortality. Shaded areas represent 95% confidence intervals for the odds ratio
Subgroup analyses revealed that participants with higher levels of education and household income had a higher estimated risk of death from most causes in association with flooding exposure. Participants aged below 64 and female had a higher estimated risk of death from all-cause mortality, respiratory diseases, and neoplasm, but a lower estimated risk of death from digestive and mental diseases, respectively. The risk of suicide-related mortality in association with flooding exposure was higher among participants who were obese, had lower household income, engaged in less physical activity, were non-moderate alcohol consumers, and had high deprivation levels (Table 3 ).
Our sensitivity analysis suggested that using multiple imputed data did not change study findings (Additional file 1 : Fig. S3). Our results were not dependent on modelling assumptions and remained unaffected by the COVID-19 pandemic (Additional file 1 : Fig. S4–7). Matching ratios of 1:4 and 1:6 revealed a modest increase in the odds ratio for all-cause mortality and neoplasms, while the odds ratio for other causes of death remained unchanged ( Additional file 1 : Fig. S8 ). For all-cause mortality, with per unit increase in monthly flood index, odds ratios over 0–12 months preceding the mortality ranged from 1.005 (95% CI: 1.005–1.006) to 1.018 (95% CI: 1.017–1.018) (Additional file 1 : Table S5).
In this nested case-control study, we observed a significantly increasing risk of mortality associated with floods. The exposure-response curve was linear, with no discernible thresholds. The lag pattern varied across different causes of death. Flooding exposure has a long-lasting impact on neurodegenerative and mental diseases, whereas it has an immediate impact on suicide. Subgroup analyses revealed specific groups of vulnerable populations for flood-related death, which varied according to the cause of death.
Every unit increase in flood index was associated with a 6.7% increase in all-cause mortality risk over the following 6 years. This finding was similar to cause-specific mortality. Although few epidemiological studies have assessed the long-term effect of floods on mortality, our findings are consistent with previous findings of short-term flooding exposure showing increased risk of cholera at lag 0–20 weeks [ 38 ], diarrhoea at lag 0–28 weeks [ 38 ], respiratory infection at lag 3 months [ 39 ], typhoid fever at lag 1 week [ 40 ], malaria at lag 1 year [ 12 ], malnutrition at lag 1 year [ 41 ], and mental disorders at lag 6 months [ 19 ]. One study assessing the effects of flooding on mortality in England and Wales during 1994–2005 suggested a deficit of deaths in the post-flood period [ 42 ]. The inconsistency might result from the underestimation of death number, which can occur when deaths are registered at different places after displacement and a short observation period (one year after flooding exposure) during which the occurrence of death has not been observed. Milojevic et al. reported a slight but non-significant increase in mortality rates following the floods in Bangladesh in the flooded areas compared to non-flooded areas [ 43 ]. The accuracy of their results might be subject to recall bias in exposure assessment, given that exposure to flooding was ascertained from an interview survey four years after the flood event.
The long-term health deterioration resulting from floods could be attributed to mental health disorders driven by financial losses and community or social disruption, especially for those who live in resource-poor countries and communities (e.g. floodplains or non-resistant buildings, lack of warning systems and awareness of flooding hazard) [ 1 , 44 ]. For example, previous studies reported a significant and continued increase in the prevalence of post-traumatic stress disorder (PTSD), stress, anxiety, depression, and even suicide ideation following flooding exposure [ 20 ], which contribute to worse health outcomes. Additionally, among people who were exposed to floods, those who had chronic medical conditions are at higher risk of health deterioration due to potential disruptions in medication and healthcare services. Previous studies have noted that older adults and those receiving long-term care services showed decreased treatment adherence (e.g. interruption of medication and access to physicians) months and even years after the flooding [ 45 , 46 ], which serve to exacerbate or prolong symptoms of existing conditions.
Flooding exposure has a long-lasting impact on neurodegenerative and mental diseases, reaching its peak at 3–4 years post-exposure whereas the highest risk of mortality due to suicide occurs in the year of exposure. This suggests that varying health issues should be given consideration, depending on the stage following flooding exposure. A study in Queensland has noted that direct exposure to flood resulted in an increase in alcohol and tobacco usage half a year after flooding [ 46 ] and use of substances has been associated with an increased risk of suicide attempts in previous studies [ 47 , 48 , 49 , 50 ]. However, social support and compensation coverage have been demonstrated to have had a positive impact on health [ 20 ], which helps reduce the risk of subsequent suicide attempts. By contrast, cognitive decline is more likely to occur 2 years later after natural disasters [ 51 , 52 ], resulting from the new onset of depression and disruption of social contacts (e.g. loss of interactions with neighbours) [ 51 ].
Our findings align with previous studies highlighting the vulnerability of cancer patients to disruptions in healthcare services following natural disasters. While limited evidence suggests an increased risk of disease exacerbation among cancer patients post-disaster, our primary concern is the potential for delays in receiving essential cancer care [ 14 , 18 ]. Natural disasters like floods can severely disrupt healthcare systems, leading to damage to oncology centres, loss of medical records, pharmaceutical shortages, displacement of healthcare workers, and disruptions in pathology specimen handling, all of which can compromise cancer patient care [ 13 , 53 , 54 , 55 , 56 ]. The relocation of cancer patients to temporary shelters can be particularly challenging and distressing, especially for those with clinical instability [ 53 ]. Additionally, initial recovery efforts following natural disasters often prioritize immediate needs such as providing shelter, food, water, and addressing injuries from environmental hazards, infectious diseases, or other acute conditions [ 57 ]. This prioritization of immediate needs may inadvertently overlook the continuity of care required for non-acute medical issues like cancer. Given the individualized and continuous nature of cancer treatment, neoplasms are particularly susceptible to the disruptions caused by natural disasters. Our study demonstrates the association between floods and elevated mortality risks among cancer patients, reinforcing the urgent need to prioritize the needs of cancer patients before, during, and after disasters [ 13 , 58 ].
Profiles of vulnerable populations to flood-related mortality varied across causes of death. Of all factors considered, socio-economic status, which is determined by individual levels of education and income, has been identified as a significant modifier of flood-related mortality impacts. Individuals with higher socioeconomic status tend to have an increased risk of flood-related mortality from chronic diseases (e.g. cardiovascular diseases, respiratory diseases, and neurodegenerative diseases) but decreased risk of flood-related mortality from suicide. Although there is very limited evidence that can elucidate this finding, some insights can be gathered from the following studies. It is reported that people in high socioeconomic groups are more likely to be affected by work-life conflict-induced mental illness due to their higher occupational aspirations but a greater discrepancy between aspirations and reality [ 59 , 60 ]. Flooding exposure may further amplify the disparity between an individual’s aspirations and their actual circumstances, resulting in a negative impact on their mental health. Long-lasting psychological illness has been associated with worse chronic medical conditions [ 61 ].
In our study, we observed that participants with higher BMI and lower physical activity levels exhibited a significantly higher risk of flood-related mortality from suicide, but a comparatively lower risk of all-cause mortality. These associations can be attributed to different factors. On the one hand, individuals in low socio-economic groups, those engaged in minimal physical activity, and non-moderate alcohol drinkers, are at higher risk of developing suicide ideation in a short time following psychological trauma associated with flooding exposure [ 49 , 62 ]. On the other hand, individuals with higher levels of physical activity may be prone to engage in risk-taking behaviours during flooding events, potentially leading to increased mortality rates [ 63 ]. These behaviours could involve actions such as entering floodwaters to cross a river or stream, safeguarding property and families (e.g. through activities like sandbagging homes and clearing drains), and participating in rescuing operations [ 63 ]. Surprisingly, current smokers demonstrated a decreased risk of flood-related mortality from all-cause deaths and neoplasms. We acknowledge that residual confounding, raising from unmeasured factors at follow-up, might contribute to these associations. Nevertheless, it is important to note that our study represents the first report of a higher mortality risk after long-term exposure to floods, highlighting the need for further investigations to validate this finding and explore potential underlying reasons.
Based on our research, flooding exposure is responsible for advancing a substantial number of deaths, with the impact persisting for up to 6 years. Our findings suggest that preventive interventions should be implemented peri- and post-flooding periods to reduce avoidable deaths due to flooding exposure. Following a flooding event, there is an increased risk of suicide within the first year. Therefore, timely provision of coping support and stress management is crucial to avert psychological illness, particularly among individuals in low socio-economic groups, those engaged in less physical activity, and non-moderate alcohol drinkers. In long-term rehabilitation, more resources should be allocated towards addressing the chronic medical conditions of populations that have been exposed to flooding, especially neurological well-being. It is also crucial to pay attention to the high-income population, although further research is needed to elucidate the underlying mechanisms behind their greater mortality risks associated with flooding exposure.
The limitations merit consideration. Our participants were residents in the UK who were more likely to live in less socioeconomically deprived areas, therefore, our results may not be generalizable to a whole population, especially people in low- and middle-income countries. Like most of cohort studies, covariates were collected at enrolment in the biobank. Due to the limited information on behavioural changes after the baseline examinations, we are unable to exclude the effect of behavioural changes on the risk estimates. However, most of the covariates (e.g. socio-economic status) were considered as effect modifiers rather than confounders, therefore, any changes in these factors should not have a substantial impact on our estimates. While the flood index accounts for cumulative exposure, it does not yet capture the potential differential impacts of distinct flood phases (warning, event, post-event) on mortality. Further research with a short-term design would be helpful to investigate the impacts of distinct flood phases. The destructive power of floods can differ based on factors like terrain, altitude, water management, drainage, urbanization, and building design. Therefore, a single severity label for an entire flood event may not fully capture the nuances of varying local experiences. Further research is needed to refine our exposure assessment as more detailed data becomes available. Lastly, it is likely for people to move after flooding exposure, with or without moving back into their homes. We assumed that participants did not move, which may have underestimated the effect of floods if an individual moved from an area with a high risk of flooding to an area with a lower risk of flooding. However, exposure to flooding can still have a long-term impact on them due to potential property damage and financial loss, even if people relocate to areas with a low risk of flooding in the aftermath of the event.
In conclusion, this study provides robust epidemiological evidence for associations of long-term exposure to flooding with increased risk of mortality. The health consequences of flooding exposure can vary across different periods after the event. These findings contribute to a better understanding of the long-term impacts of flooding exposure and can help improve public health practices to reduce the disease burden associated with floods.
Availability of data and materials
Data used in this study are available through registration on the UK Biobank.
Abbreviations
Akaike Information Criterion
Bayesian Information Criterion
Body mass index
Confidence interval
Coronavirus disease 2019
Dartmouth Flood Observatory
European Centre for Medium-Range Weather Forecasts Reanalysis v5
International Classification of Diseases, edition 10
International Physical Activity Questionnaire-Short Form
Metabolic equivalent minutes per week
Post-traumatic stress disorder
Standard deviation
Townsend deprivation index
Lee J, Perera D, Glickman T, Taing LN. Water-related disasters and their health impacts: a global review. Prog Disaster Sci. 2020;8:100123.
Article Google Scholar
Wahlstrom M, Guha-Sapir D. The human cost of weather-related disasters 1995–2015. Geneva: UNISDR. 2015.
Wahlstrom M, Guha-Sapir D. The human cost of natural disasters: a global perspective. Geneva: UNISDR. 2015.
Rubinato M, Nichols A, Peng Y, Zhang JM, Lashford C, Cai YP, et al. Urban and river flooding: Comparison of flood risk management approaches in the UK and China and an assessment of future knowledge needs. Water Sci Eng. 2019;12(4):274–83.
Pitt M. Learning Lessons From the 2007 Floods. An Independent Review by Sir Michael Pitt. Cabinet Office, London. 2008.
Pregnolato M, Ford A, Wilkinson SM, Dawson RJ. The impact of flooding on road transport: A depth-disruption function. Transp Res D Transp Environ. 2017;55:67–81.
Thorne C. Geographies of UK flooding in 2013/4. Geogr J. 2014;180(4):297–309.
Marsh T, Kirby C, Muchan K, Barker L, Henderson E, Hannaford J. The winter floods of 2015/2016 in the UK-a review: UK: NERC/Centre for Ecology and Hydrology; 2016.
Wallemacq P, Below R, McClean D. Economic losses, poverty and disasters: 1998-2017: Geneva: United Nations Office for Disaster Risk Reduction; 2018.
Sayers P, Horritt M, Carr S, Kay A, Mauz J, Lamb R, et al. Third UK Climate Change Risk Assessment (CCRA3): Future flood risk. UK: Research undertaken by Sayers and Partners for the Committee on Climate Change; 2020.
Jonkman SN, Kelman I. An analysis of the causes and circumstances of flood disaster deaths. Disasters. 2005;29(1):75–97.
Article PubMed Google Scholar
Boyce R, Reyes R, Matte M, Ntaro M, Mulogo E, Metlay JP, et al. Severe Flooding and Malaria Transmission in the Western Ugandan Highlands: Implications for Disease Control in an Era of Global Climate Change. J Infect Dis. 2016;214(9):1403–10.
Article PubMed PubMed Central Google Scholar
Man RX, Lack DA, Wyatt CE, Murray V. The effect of natural disasters on cancer care: a systematic review. Lancet Oncol. 2018;19(9):e482–99.
Ryan B, Franklin RC, Burkle FM Jr, Aitken P, Smith E, Watt K, et al. Identifying and Describing the Impact of Cyclone, Storm and Flood Related Disasters on Treatment Management, Care and Exacerbations of Non-communicable Diseases and the Implications for Public Health. PLoS Curr. 2015;7:21–50.
Google Scholar
McKinney N, Houser C, Meyer-Arendt K. Direct and indirect mortality in Florida during the 2004 hurricane season. Int J Biometeorol. 2011;55(4):533–46.
Rath B, Young EA, Harris A, Perrin K, Bronfin DR, Ratarh R, et al. Adverse Respiratory Symptoms and Environmental Exposures Among Children and Adolescents Following Hurricane Katrina. Public Health Rep. 2011;126(6):853–60.
Buajaroen H. Management of health care services for flood victims: The case of the shelter at Nakhon Pathom Rajabhat University Central Thailand. Australas Emerg Nurs. 2013;16(3):116–22.
Loehn B, Pou AM, Nuss DW, Tenney J, McWhorter A, DiLeo M, et al. Factors affecting access to head and neck cancer care after a natural disaster: a post-Hurricane Katrina survey. Head Neck. 2011;33(1):37–44.
Alderman K, Turner LR, Tong S. Assessment of the health impacts of the 2011 summer floods in Brisbane. Disaster Med Public Health Prep. 2013;7(4):380–6.
Zhong S, Yang L, Toloo S, Wang Z, Tong S, Sun X, et al. The long-term physical and psychological health impacts of flooding: A systematic mapping. Sci Total Environ. 2018;626:165–94.
Article CAS PubMed Google Scholar
Trief PM, Ouimette P, Wade M, Shanahan P, Weinstock RS. Post-traumatic stress disorder and diabetes: co-morbidity and outcomes in a male veterans sample. J Behav Med. 2006;29(5):411–8.
Sharpe I, Davison CM. Climate change, climate-related disasters and mental disorder in low- and middle-income countries: a scoping review. BMJ Open. 2021;11(10):e051908.
Carozza DA, Boudreault M. A global flood risk modeling framework built with climate models and machine learning. J Adv Model Earth Syst. 2021;13(4):e2020MS002221.
Yang Z, Huang W, McKenzie JE, Xu R, Yu P, Ye T, et al. Mortality risks associated with floods in 761 communities worldwide: time series study. BMJ. 2023;383:e075081.
Steenland K, Seals R, Klein M, Jinot J, Kahn HD. Risk estimation with epidemiologic data when response attenuates at high-exposure levels. Environ Health Perspect. 2011;119(6):831–7.
White E, Hunt JR, Casso D. Exposure measurement in cohort studies: the challenges of prospective data collection. Epidemiol Rev. 1998;20(1):43–56.
Ganna A, Ingelsson E. 5 year mortality predictors in 498,103 UK Biobank participants: a prospective population-based study. Lancet. 2015;386(9993):533–40.
Wang M, Zhou T, Song Q, Ma H, Hu Y, Heianza Y, et al. Ambient air pollution, healthy diet and vegetable intakes, and mortality: a prospective UK Biobank study. Int J Epidemiol. 2022;51(4):1243–53.
Wang M, Zhou T, Song Y, Li X, Ma H, Hu Y, et al. Joint exposure to various ambient air pollutants and incident heart failure: a prospective analysis in UK Biobank. European heart journal. 2021;42(16):1582–91.
Article CAS PubMed PubMed Central Google Scholar
IPAQ Research Committee. Guidelines for data processing and analysis of the International Physical Activity Questionnaire (IPAQ)-short and long forms. https://www.physio-pedia.com/images/c/c7/Quidelines_for_interpreting_the_IPAQ.pdf (accessed January 20, 2024).
Zhang YB, Chen C, Pan XF, Guo J, Li Y, Franco OH, et al. Associations of healthy lifestyle and socioeconomic status with mortality and incident cardiovascular disease: two prospective cohort studies. BMJ. 2021;373:n604.
Chen H, Cao Y, Ma Y, Xu W, Zong G, Yuan C. Age- and sex-specific modifiable risk factor profiles of dementia: evidence from the UK Biobank. Eur J Epidemiol. 2023;38(1):83–93.
Jung CR, Chung WT, Chen WT, Lee RY, Hwang BF. Long-term exposure to traffic-related air pollution and systemic lupus erythematosus in Taiwan: A cohort study. Sci Total Environ. 2019;668:342–9.
Gasparrini A. Modeling exposure-lag-response associations with distributed lag non-linear models. Stat Med. 2014;33(5):881–99.
Li Y-Z, Huang S-H, Shi S, Chen W-X, Wei Y-F, Zou B-J, et al. Association of long-term particulate matter exposure with all-cause mortality among patients with ovarian cancer: A prospective cohort. Sci Total Environ. 2023;884:163748.
Kriit HK, Andersson EM, Carlsen HK, Andersson N, Ljungman PL, Pershagen G, et al. Using distributed lag non-linear models to estimate exposure lag-response associations between long-term air pollution exposure and incidence of cardiovascular disease. Int J Env Res Pub He. 2022;19(5):2630.
Article CAS Google Scholar
Rubin DB. Multiple imputation for nonresponse in surveys: Canada: Wiley; 2004.
Hashizume M, Wagatsuma Y, Faruque ASG, Hayashi T, Hunter PR, Armstrong B, et al. Factors determining vulnerability to diarrhoea during and after severe floods in Bangladesh. J Water Health. 2008;6(3):323–32.
Saulnier DD, Hanson C, Ir P, Alvesson HM, von Schreeb J. The Effect of Seasonal Floods on Health: Analysis of Six Years of National Health Data and Flood Maps. Int J Env Res Pub He. 2018;15(4):665–77.
Liu ZD, Lao JH, Zhang Y, Liu YY, Zhang J, Wang H, et al. Association between floods and typhoid fever in Yongzhou, China: Effects and vulnerable groups. Environ Res. 2018;167:718–24.
Rodriguez-Llanes JM, Ranjan-Dash S, Mukhopadhyay A, Guha-Sapir D. Flood-Exposure Is Associated with Higher Prevalence of Child Undernutrition in Rural Eastern India. Int J Env Res Pub He. 2016;13(2):210.
Milojevic A, Armstrong B, Kovats S, Butler B, Hayes E, Leonardi G, et al. Long-term effects of flooding on mortality in England and Wales, 1994–2005: controlled interrupted time-series analysis. Environ Health. 2011;10(1):11–9.
Milojevic A, Armstrong B, Hashizume M, McAllister K, Faruque A, Yunus M, et al. Health Effects of Flooding in Rural Bangladesh. Epidemiology. 2012;23(1):107–15.
Ahern M, Kovats RS, Wilkinson P, Few R, Matthies F. Global health impacts of floods: Epidemiologic evidence. Epidemiol Rev. 2005;27:36–46.
Tomio J, Sato H, Mizumura H. Interruption of Medication among Outpatients with Chronic Conditions after a Flood. Prehospital Disaster. 2010;25(1):42–50.
Turner LR, Alderman K, Huang CR, Tong SL. Impact of the 2011 Queensland floods on the use of tobacco, alcohol and medication. Aust Nz J Publ Heal. 2013;37(4):396.
Orri M, Seguin JR, Castellanos-Ryan N, Tremblay RE, Cote SM, Turecki G, et al. A genetically informed study on the association of cannabis, alcohol, and tobacco smoking with suicide attempt. Mol Psychiatr. 2021;26(9):5061–70.
Berlin I, Hakes JK, Hu MC, Covey LS. Tobacco Use and Suicide Attempt: Longitudinal Analysis with Retrospective Reports. Plos One. 2015;10(4):e0122607.
Darvishi N, Farhadi M, Haghtalab T, Poorolajal J. Alcohol-Related Risk of Suicidal Ideation, Suicide Attempt, and Completed Suicide: A Meta-Analysis. Plos One. 2015;10(5):e0126870.
Gobbi G, Atkin T, Zytynski T. Association of Cannabis Use in Adolescence and Risk of Depression, Anxiety, and Suicidality in Young Adulthood: A Systematic Review and Meta-analysis. Jama Psychiat. 2019;76(4):426–34.
Hikichi H, Aida J, Kondo K, Tsuboya T, Matsuyama Y, Subramanian SV, et al. Increased risk of dementia in the aftermath of the 2011 Great East Japan Earthquake and Tsunami. P Natl Acad Sci USA. 2016;113(45):E6911–8.
Akanuma K, Nakamura K, Meguro K, Chiba M, Gutierrez Ubeda SR, Kumai K, et al. Disturbed social recognition and impaired risk judgement in older residents with mild cognitive impairment after the Great East Japan Earthquake of 2011: the Tome Project. Psychogeriatrics. 2016;16(6):349–54.
Morikawa N, Yanagisawa S, Iwasashi H, Tabata T, Abe T, Nakamura R, et al. Cancer patients in the hospital damaged by the Japan earthquake and tsunami. J Clin Oncol. 2012;30(15):e19508-e.
Cancer Ullman K, Disasters Care During Natural. J Natl Cancer I. 2011;103(24):1819–20.
Arrieta MI, Foreman RD, Crook ED, Icenogle ML. Providing Continuity of Care for Chronic Diseases in the Aftermath of Katrina: From Field Experience to Policy Recommendations. Disaster Med Public. 2009;3(3):174–82.
Rosenthal E. How the Cancer Community Fared During Hurricane Sandy's Mid-Atlantic Sweep. Oncology Times. 2012;34(23):8-10.
Prasad AS, Francescutti LH. Natural disasters. International encyclopedia of public health. 2017;5:215-22.
Aitsi-Selmi A, Murray V. Protecting the Health and Well-being of Populations from Disasters: Health and Health Care in The Sendai Framework for Disaster Risk Reduction 2015–2030. Prehospital Disaster. 2016;31(1):74–8.
Cochran DB, Wang EW, Stevenson SJ, Johnson LE, Crews C. Adolescent Occupational Aspirations: Test of Gottfredson’s Theory of Circumscription and Compromise. Career Dev Q. 2011;59(5):412–27.
Kim YM, Cho SI. Socioeconomic status, work-life conflict, and mental health. Am J Ind Med. 2020;63(8):703–12.
Patten SB, Williams JVA, Lavorato DH, Modgill G, Jette N, Eliasziw M. Major depression as a risk factor for chronic disease incidence: longitudinal analyses in a general population cohort. Gen Hosp Psychiat. 2008;30(5):407–13.
Vancampfort D, Hallgren M, Firth J, Rosenbaum S, Schuch FB, Mugisha J, et al. Physical activity and suicidal ideation: A systematic review and meta-analysis. J Affect Disorders. 2018;225:438–48.
Hamilton K, Demant D, Peden AE, Hagger MS. A systematic review of human behaviour in and around floodwater. Int J Disaster Risk Reduction. 2020;47:101561.
Download references
Acknowledgements
We gratefully thank all the participants in the UK Biobank, and everyone involved in planning and conducting the study.
Australian Research Council grant DP210102076
Australian National Health and Medical Research Council grant GNT2000581)
China Scholarship Council grant 202006010044 (YW)
China Scholarship Council grant 202006010043 (BW)
China Scholarship Council grant 201906210065 (PY)
National Health and Medical Research Council grant GNT2009866 (SL)
National Health and. Medical Research Council grant GNT1163693 (YG)
National Health and Medical Research Council grant GNT2008813 (YG)
Author information
Hong Liu, Shanshan Li, and Yuming Guo are co-senior authors.
Authors and Affiliations
School of Public Health and Preventive Medicine, Monash University, Level 2, 553 St Kilda Road, Melbourne, VIC, 3004, Australia
Yao Wu, Danijela Gasevic, Bo Wen, Zhengyu Yang & Pei Yu
Department of Dermatology, Xiangya Hospital, Central South University, Changsha, 410008, Hunan, China
Guowei Zhou, Yan Zhang, Hong Liu, Shanshan Li & Yuming Guo
Department of Biochemistry and Molecular Biology, Monash Biomedicine Discovery Institute, Monash University, Melbourne, VIC, 3800, Australia
Jiangning Song
You can also search for this author in PubMed Google Scholar
Contributions
Conceptualization: YG, JS, HL, SL, YW. Methodology: YW, DG. Data collection: BW, ZY, GZ, YZ, ZY
Visualization: YW, BW. Supervision: YG, HL, SL. Writing—original draft: YW. Writing—review and editing: DG, BW, ZY, PY. All authors read and approved the final manuscript.
Authors’ Twitter handles
Yuming Guo: @YumingGuo007
Yao Wu: @Yaoyaowu13
Corresponding author
Correspondence to Yuming Guo .
Ethics declarations
Ethics approval and consent to participate.
UK Biobank has ethical approval from the North West Multi-Centre Research Ethics Committee (reference 16/NW/0274).
Consent for publication
Consent for publication was obtained from all included participants.
Competing interests
The authors declare that they have no competing interests.
Additional information
Publisher’s note.
Springer Nature remains neutral with regard to jurisdictional claims in published maps and institutional affiliations.
Supplementary Information
Additional file 1:.
Table S1. Definitions of different severities of flood events. Table S2. The Akaike Information Criterion (AIC) and the Bayesian Information Criterion (BIC) of nonlinear and linear models. Table S3. Baseline characteristics of cases and matched controls enrolled in UK Biobank, including missing values. Table S4. Cumulative odds ratios of cause-specific mortality associated with per unit increase in flood index over lag years 0–5. Table S5. Cumulative odds ratios of all-cause mortality associated with per unit increase in monthly flood index over lag month 0–12. Figure S1. Nonlinear curves of the associations between flood index and all-cause and cause-specific mortality. Figure S2. Cumulative flood index of cases and controls during the six years before the date of death or the end of the follow-up. Figure S3. Cumulative odds ratios of all-cause and cause-specific mortality associated with per unit increase in flood index over lag years 0–5 using complete data after multiple imputation. Figure S4. Cumulative odds ratios of all-cause and cause-specific mortality associated with per unit increase in flood index over lag years 0–5 using different degrees of freedom for lag-response association of flood index. Figure S5. Cumulative odds ratios of all-cause and cause-specific mortality associated with per unit increase in flood index over lag years 0–5 using different degrees of freedom for mean temperature. Figure S6. Cumulative odds ratios of all-cause and cause-specific mortality associated with per unit increase in flood index over lag years 0–5 using different degrees of freedom for relative humidity. Figure S7. Cumulative odds ratios of all-cause and cause-specific mortality associated with per unit increase in flood index over lag years 0–5 after excluding deaths after 2020. Figure S8. Cumulative odds ratios of all-cause and cause-specific mortality associated with per unit increase in flood index over lag years 0–5 using different matching ratios.
Rights and permissions
Open Access This article is licensed under a Creative Commons Attribution 4.0 International License, which permits use, sharing, adaptation, distribution and reproduction in any medium or format, as long as you give appropriate credit to the original author(s) and the source, provide a link to the Creative Commons licence, and indicate if changes were made. The images or other third party material in this article are included in the article's Creative Commons licence, unless indicated otherwise in a credit line to the material. If material is not included in the article's Creative Commons licence and your intended use is not permitted by statutory regulation or exceeds the permitted use, you will need to obtain permission directly from the copyright holder. To view a copy of this licence, visit http://creativecommons.org/licenses/by/4.0/ . The Creative Commons Public Domain Dedication waiver ( http://creativecommons.org/publicdomain/zero/1.0/ ) applies to the data made available in this article, unless otherwise stated in a credit line to the data.
Reprints and permissions
About this article
Cite this article.
Wu, Y., Gasevic, D., Wen, B. et al. Floods and cause-specific mortality in the UK: a nested case-control study. BMC Med 22 , 188 (2024). https://doi.org/10.1186/s12916-024-03412-0
Download citation
Received : 27 November 2023
Accepted : 29 April 2024
Published : 07 May 2024
DOI : https://doi.org/10.1186/s12916-024-03412-0
Share this article
Anyone you share the following link with will be able to read this content:
Sorry, a shareable link is not currently available for this article.
Provided by the Springer Nature SharedIt content-sharing initiative
- Natural disaster
BMC Medicine
ISSN: 1741-7015
- Submission enquiries: [email protected]
- General enquiries: [email protected]
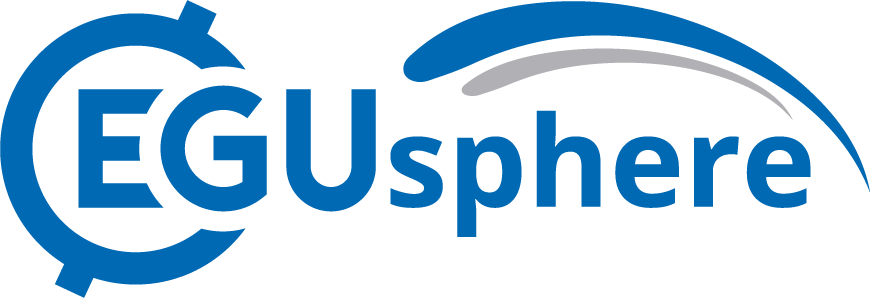
Preprint
- Preprint egusphere-2024-1030
Long Short-Term Memory Networks for Real-time Flood Forecast Correction: A Case Study for an Underperforming Hydrologic Model
Abstract. Flood forecasting systems play a key role in mitigating socio-economic damages caused by flooding events. The majority of these systems rely on process-based hydrologic models (PBHM), which are used to predict future river runoff. To enhance the forecast accuracy of these models, many operational flood forecasting systems implement error correction techniques, which is particularly important if the underlying hydrologic model is underperforming. Especially, AutoRegressive Integrated Moving Average (ARIMA) type models are frequently employed for this purpose. Despite their high popularity, numerous studies have pointed out potential shortcomings of these models, such as a decline in forecast accuracy with increasing lead time. To overcome the limitations presented by conventional ARIMA models, we propose a novel forecast correction technique based on a hindcast-forecast Long Short-Term Memory (LSTM) network. We showcase the effectiveness of the proposed approach by rigorously comparing its capabilities to those of an ARIMA model, utilizing one underperforming PBHM as a case study. Additionally, we test whether the LSTM benefits from the PBHM's results or if a similar accuracy can be reached by employing a standalone LSTM. Our investigations show that the proposed LSTM model significantly improves the PBHM's forecasts. Compared to ARIMA, the LSTM achieves a higher forecast accuracy for longer lead times. In terms of flood event runoff, the LSTM performs mostly on par with ARIMA in predicting the magnitude of the events. However, the LSTM majorly outperforms ARIMA in accurately predicting the timing of the peak runoff. Furthermore, our results provide no reliable evidence of whether the LSTM is able to extract information from the PBHM's results, given the widely equal performance of the proposed and standalone LSTM models.
- Preprint (PDF, 16342 KB)
- Preprint (16342 KB)
- Metadata XML

Status : open (until 09 Jul 2024)
Report abuse
Please provide a reason why you see this comment as being abusive. You might include your name and email but you can also stay anonymous.
Please provide a reason why you see this comment as being abusive.
Please confirm reCaptcha.

Model code and software
Model code for "Long Short-Term Memory Networks for Real-time Flood Forecast Correction: A Case Study for an Underperforming Hydrologic Model" Sebastian Gegenleithner, Manuel Pirker, Clemens Dorfmann, Roman Kern, and Josef Schneider https://github.com/tug17/ForecastModel
Interactive computing environment
Plots for "Long Short-Term Memory Networks for Real-time Flood Forecast Correction: A Case Study for an Underperforming Hydrologic Model" Sebastian Gegenleithner, Manuel Pirker, Clemens Dorfmann, Roman Kern, and Josef Schneider https://github.com/tug17/ForecastModel
Viewed (geographical distribution)
Sebastian gegenleithner, manuel pirker, clemens dorfmann, josef schneider.
Advertisement
Flood risk and adaptation in Indian coastal cities: recent scenarios
- Review Article
- Open access
- Published: 04 December 2018
- Volume 9 , article number 5 , ( 2019 )
Cite this article
You have full access to this open access article
- Ravinder Dhiman 1 na1 ,
- Renjith VishnuRadhan 2 na1 ,
- T. I. Eldho 2 &
- Arun Inamdar 3
25k Accesses
82 Citations
34 Altmetric
Explore all metrics
Coastal cities contrive to spread their transformative influence both into the hinterland, along the coastline, and into the coastal waters themselves. These effects will be intensified in urban agglomerations as the concentration of population and allied activities are more pronounced there compared to the inland regions. Indian coastal cities are no exception, and it is high time to delineate these hazard-prone regions and implement proper mitigation and adaptation strategies at city scale. This review article provides an assessment regarding quantification, management and climate change impacts of flood risks in Surat, Mumbai, Chennai and Kolkata, which are the most populated coastal cities in India. The flood impacts considered in the existing or prevailing analyses are associated with adverse effects on population, land use of cities, transportation and economy caused by different types of riverine and urban flooding, though coastal flooding, tsunami and storm surge effects are less studied. Mumbai and Kolkata are relatively progressive in the assessment of flood risks and adaptation. The present article also suggests strategies to evaluate the relative progress in the assessment of past and future risks and adaptation. We also discuss the mitigation and adaptation strategies considering the historical importance of these cities. We propose that the strategies should be implemented considering public opinion and should be initialized at the grass root level. Though it is technically difficult to re-plan the city structures in the current scenario, it is possible to adapt to and mitigate the effects of natural hazards through suitable planning and management with the integrated cooperation and involvement of citizens and government as well.
Similar content being viewed by others
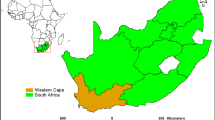
Flooding trends and their impacts on coastal communities of Western Cape Province, South Africa
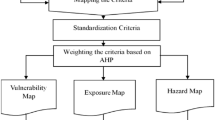
Coastal Flood Induced Salinity Intrusion Risk Assessment Using a Spatial Multi-criteria Approach in the South-Western Bangladesh
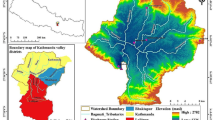
Characterizing urban flooding in the Kathmandu Valley, Nepal: the influence of urbanization and river encroachment
Avoid common mistakes on your manuscript.
Introduction
Urban centres are dynamic interfaces and regions of intense economic activities. The growing significance of urban areas is recognized by including Goal 11 (sustainable cities and communities) in the United Nations Sustainable Developmental Goals (SGDs). Cities are knowledge and innovation hubs, that can drive the urban system towards better governance of health and the climate within cities, and are centres which catalyse societal transformations (de Oliveira and Doll 2016 ). Most of the urban regions are located in coastal areas (Small and Nicholls 2003 ). As urban areas grow, correspondingly the population and its allied pressures also increase in the coastline. In addition to natural changes, these coastal environments face multifaceted hazards due to anthropogenic fossil fuel combustion, fertilizer use, sewage input, industrial activities, tourism, overfishing, aquaculture farms and marine traffic (VishnuRadhan et al. 2014 ). The coastal cities are important drivers of socio-economic development as well as sources of environmental challenges (von Glasow et al. 2013 ), and nearly half of the world population now lives within 200 km of the coast, and it is expected to reach 75% by 2025 (UNESCO 2009 ; Creel 2003 ). Currently, India is the second most populous nation and has large coastline compared to most of the other nations around the globe; the pressure in its coastal zones is beyond imagination. India ranks 18th in terms of coastline length, and the total coastline is 7517 km including the Andaman and Nicobar Islands in the Bay of Bengal and the Lakshadweep Islands in the Arabian Sea (IYB 2007 ). The majority of the population of this agriculture-dependant country in the Indian Ocean rim is depended on the Indian summer monsoon for their survival and daily livelihood. As the monsoon is one of the most unpredictable climate phenomena in the globe (Jaiswal et al. 2018 ; Mahmood et al. 2018 ; Shahi et al. 2018 ), it can substantially affect the population not only in terms of their basic livelihood, but also by inducing geohazards such as flash floods and inundation. In the case of coastal cities, the effect will be exacerbated as these are densely populated and are prone to sea-level rise, storm surges and tropical cyclones, of which recent studies portraits are intense under climate change scenarios (Mendelsohn et al. 2012 ; Rahmstorf 2017 ; Vitousek et al. 2017 ).
The city scale has ever been more recognized for risks management and adaptation in recent years (Bai et al. 2018 ; Ürge-Vorsatz et al. 2018 ; Rodriguez et al. 2018 ; Solecki et al. 2018 ). Analysis within the city boundary is more relevant for local administrations such that decisions for adaptation can be facilitated at an applicable level of governance. There are a number of significant impacts on cities which are directly related to climate change such as surface sealing by concrete (urban heat islands) and urban floods (Lindley et al. 2006 ). The developing countries are having greater vulnerability than developed countries at city scale, because of rapid increase in population as compared to the capacity of infrastructure, resulting in the deficit of their adaptation measures towards climate variability and future exposure to flood risks (Hunt and Watkiss 2011 ). The definition of the coastal megacity is debatable in the literature because of the inconsistency in the threshold of megacity which is 1, 8 and 10 million people (Cross 2001 ), but the United Nations has defined the megacity as having population more than 10 million (UN 2008 ). We have considered coastal megacities following Nicholls and Small ( 2002 ). Kolkata is located 145 km away from the coastline, but is considered as a coastal megacity because of the direct effect of marine processes and deltaic settings (Sekovski et al. 2012 ). Though Surat is not a megacity, the city is growing in terms of population and infrastructure and can be a potential future megacity.
This review article presents evidence-oriented flood risks and adaptation strategies in select coastal cities in India based on published literature, in addition to reviewing the methodology of information utilization. We also delineate the potential impacts of flood-induced risks in the select coastal cities: Surat, Mumbai, Kolkata and Chennai. According to the previous studies (Revi 2008 ; Bhat et al. 2013 ; Ranger et al. 2011 ; Joerin et al. 2012 ; Nicholls et al. 2008 ; Dasgupta et al. 2013 ), the most important risks posed by floods in Indian coastal cities are primarily due to (1) the riverine flooding and extreme precipitation, (2) the effect of cyclonic storms, tsunamis and tidal inundation, (3) failure in urban planning and unsustainable land-use practices. Flood risks are usually coupled with cyclones, storm surges, tides and tsunami across different geographical locations having a difference in impact categories, including riverine flooding, urban flooding, population growth, transportation, energy demand and climate variability. Füssel and Klein ( 2006 ) have mentioned the importance of qualitative approaches over quantitative estimation during the assessment of large future risks to minimize the uncertainty. The literature mentioned in this review is supposed to be indicative only of the true scope and extent of flood risks and climate change impacts along the major Indian coastal cities.
This study integrates the existing scientific studies about floods (impacts, methods and adaptation) in major Indian coastal cities precisely to make it easy for other researchers who want to pursue advancements in the similar domain. This work considers the assessment of different methodologies also, particularly techniques adapted in different studies, which results in lack of consistency. Consequently, several studies have highlighted different methods, including the action of climate models, socio-economic scenario, index-based approach, GIS and the monetary valuation of market/non-market impacts. Comparability among different approaches may assist more easy transfer of useful discoveries and observations between cities. The existing coastal city studies show that sectoral stakeholder engagement is the key to maximize the benefits of adaptation activities. We are highlighting the need of future experiments relevant to overall objectives and necessary actions of flood impacts coupled with climate change scenario. The goal of this review article is to evaluate the causes, potential risks and measures to control them at city scale under the pressure experienced along the dynamic coastline of India and is intended towards the assessment of flood impact considering all possible scenarios. Moreover, there are no review articles exclusively dedicated in addressing the flood impacts and adaptation considering the Indian coastal urban regions.
In this study, four maximum populated coastal cities along the Indian coastline, two at the west (Surat and Mumbai) coast and two at the east (Chennai and Kolkata) coast, are chosen. The selection of cities from both the west and east coasts is due to the fact that the climatic/oceanographic conditions along the two coasts are different (Fig. 1 ). These cities are vulnerable to floods by multiple causes such as rivers in the city itself (adjacent or central), faulty urban design and planning, the dynamic and vulnerable coastline, flash floods, storm surges, cyclones and tsunamis. Table 1 indicates the causative factors leading to the occurrence of floods in these coastal cities.
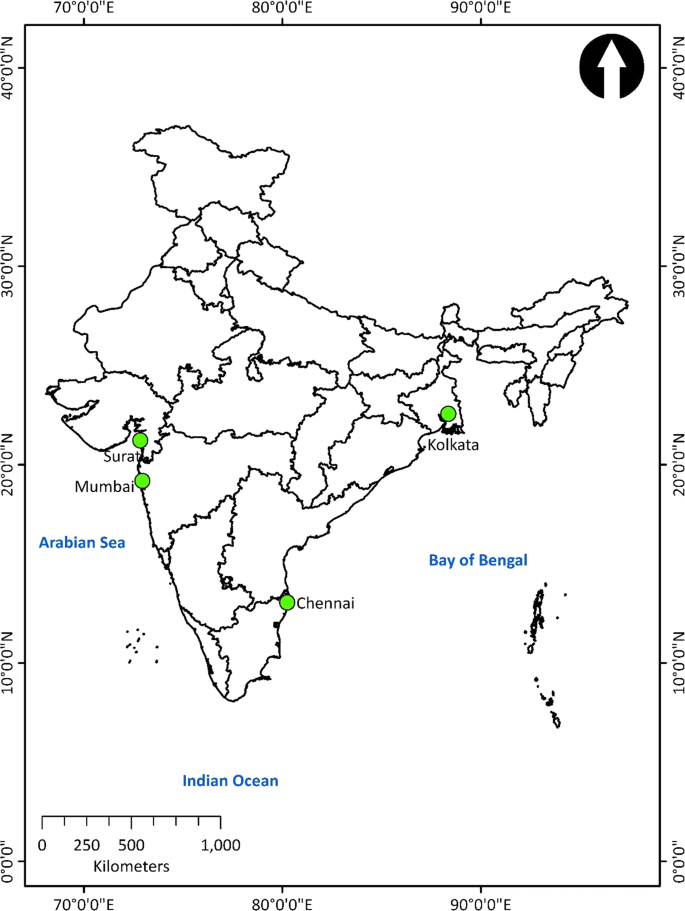
Locations map of study areas
Surat is a historical coastal city located on the bank of the Tapi River, Gujarat state. The Surat port was an active hub of trade and commerce in the past, and the city reached its utmost prosperity during the sixteenth and seventeenth century. Surat was also the main port of the Mughal Empire (Gupta 1987 ). The British East India Company established one of its first Indian factories at Surat in 1612, and this English factory has been called the cornerstone of the British Empire in India (Rawlinson 1920 ). The colonial past of the city resulted in providing well-documented past flooding events in the city. Imperial Gazetteers and written historical accounts documented details of the past floods prior to the instrumental records (Kale et al. 1996 ). The construction of an inner wall in the city was commenced by the British in the year 1664 as a flood protection structure with gates that were closed in the event of a flood, and the construction of the entire wall was completed in the year 1707. The city area expanded from 8.12 km 2 (prior to 1961) to 326.5 km 2 in 2009 (SMC 2011 ). The city can be divided geomorphologically into a coastal zone and an alluvial area. The coastal zone is composed of marshy shoreline with an extensive tidal flat stretch intercepted by estuaries. The alluvial area is marked by alluvial deposits from the Tapi River (Patel et al. 2017 ). Flooding occurs in the city when there is a sudden release of water from the Ukai dam (constructed in 1972) which is located 100 km away from the city. Floods can also be caused by seawater intrusion through different creeks which are also known as Khadi floods, less devastating than the Ukai floods though it significantly affects the vulnerable households along these creeks (Bahinipati et al. 2015 ). The city population has grown tenfold since 1951, and the municipal area has increased from 8.1 km 2 in 1951 to 327 km 2 recently (Bhat et al. 2013 ). The population was 5.9 million in 2016 and is projected to be 8.6 million in 2030 (UNDESA 2016 ), which shows more vulnerability in the near future.
Mumbai, the most populous Indian city, located along the western coast of India, started trading activity during 1675. It is the largest port in western India and simultaneously known as the commercial and financial capital of India (Pacione 2006 ). The megacity currently ranks as the 5th largest city (in terms of the population) in the globe (UNDESA 2016 ) and is originally composed of seven islets. Anthropogenic reclamation primarily caused the islets to merge and form the current Mumbai city. It is encircled by the Arabian Sea to the west and harbours the Thane Creek inlet to the east. Mithi River is located in the centre of the city having origin from Vihar and Powai Lake. Weather is typical humid and coastal sultry, and annual rainfall ranges from 15 to 20 cm (Vaz 2014 ). The population of the city during the first census of India in 1872 was around 65,000 (Da Cunha and Jose 1902 ). Since then, the city has developed extensively over the years, and correspondingly the population increased to around 21.3 million in 2016 (UNDESA 2016 ), which is projected to pass 27 million in 2030. This has created enormous pressure on the land and resulted in substantial land-use changes in the region. Burgeoning population and encroachment have resulted in the unavailability of land for residential and commercial purposes, leaving the city with the only vertical growth option. This space crisis may have prompted the state government to raise the floor space index (FSI) in 2018, which will further develop the city vertically. The city experiences frequent spells of flood during monsoon time. The primary reason for the flooding stems from the fact that the shape of the present Mumbai city was moulded by the reclamation of water-logged areas between the original seven separate islands. The reclaimed space between the islands lies below the high water level. Whenever the rainfall persists for a longer time (7–10 h) and it coincides with the time of high tides, water cannot recede into the ocean and compounds the flooding (Gupta 2009 ). Apart from this, other important reasons for flooding are mainly anthropogenic. These include inappropriate levels of outfalls, the increase in the run-off coefficient due to the urban landscape, the loss of holding ponds due to land development and encroachments on drains and obstructions caused by utility lines being crossed (MCGM 2014 ). The yearly flooding in Mumbai incurs huge economic losses due to the economical–social disorientation and associated shutdown, ultimately affecting the nation’s economy. small- and medium-sized enterprises (SMEs) often bear the cost of flooding as the industrial estates located near chronic flooding spots experience recurrent flooding at least two or three times every year (Schaer et al. 2018 ). Figure 2 shows the estimated total losses for a 1-in-100-year flood event in Mumbai under five scenarios.
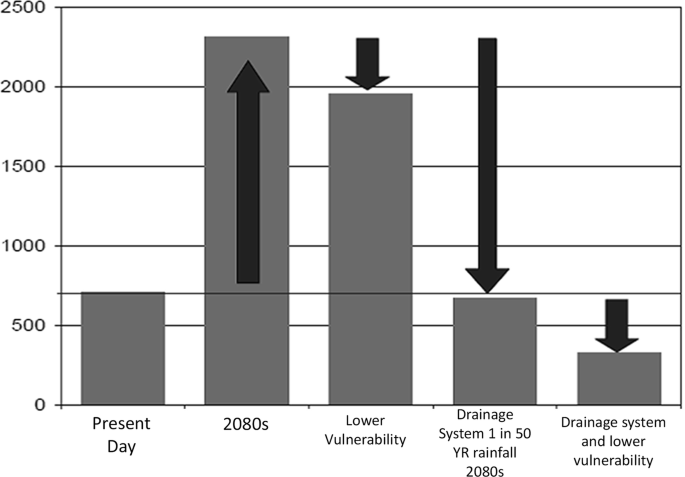
Estimated total losses for a 1-in-100-year flood events in Mumbai under five scenarios (following Hallegatte 2010 )
Chennai, formerly known as Madras, was formed in 1600 and is the capital city of Tamil Nadu state. The city is located at the southern east coast of India, bounded by the Bay of Bengal in the east. The region has persistent as well as eventful archaeological and colonial history. The City is drained by three major rivers, namely Adyar (to the south), Cooum (through the centre) and Kosasthalaiyar (to the north). The city faces a number of risks which include climate-related as well as human-induced reasons (Gupta and Nair 2010 ). Chennai receives an average approximately 1300 mm of rainfall per year, but the majority of this (~ 800 mm) falls during the north-east (NE) monsoon months (October–December). The population of the city was 777,481 (1941 Census of India) before the independence of the country, which drastically increased thereafter to a current population of 10.16 million in 2016 (UNDESA 2016 ). This number is projected to continue increasing to 13.29 million in 2030. The rise in population leads to intensive developmental activities and land-use changes. The region has plain terrain, thus lacking a natural gradient for free run-off. In order to wade off a potential flood, the city requires natural buffering mechanisms such as wetlands, marshes and open lakes. These buffering factors are significantly affected by the construction and developmental activities allied to the city growth as well as encroachments. For example, the alarming decreases in the area of the Pallikaranai marshland from 5000 ha in 1970 to 988 ha in 2008 (Stephen 2016 ). Recent devastation by the flood waters in the region was wrought mainly by the shrinkage of wetlands and marshes. Major impacts of flooding on the booming IT industry in the region and sustainability of housing sector can be expected in the years to come (Suriya et al. 2012 ).
Kolkata, previously known as Calcutta, is the capital of the Indian state of West Bengal. Calcutta was the last of the port cities to be founded by the English, and the suburbanization had been started by the British during the last decades of the eighteenth century (Kosambi and Brush 1988 ). The modern city of Kolkata was developed on land recovered from the wetlands following the Dutch model of cities built along the Rhine River despite geographical challenge similar to those in Kolkata (Rumbach 2014 ). The city is often mentioned as the city in the swamps (Murphey 1964 ) and has been found to be one of the world’s most flood-prone coastal cities (Balica et al. 2012 ). Though situated away from the Bay of Bengal, the city is considered as a coastal city because of the deltaic setting. This megacity has a tropical climate and is subjected to extreme precipitation during monsoons with an annual rainfall of around 160 cm. It is situated on the bank of the Hooghly River and has an area of 1851 km 2 . The region is prone to flooding primarily due to the flat terrain and the drainage outfalls which are tide-dominated. Flooding occurs during excess rainfall on the catchment as well as during high-tidal conditions. The drainage sewer system in the city was designed during the British era and is not capable of handling the current run-off scenario. The sewer system also suffers from siltation and a consequent reduction in conveyance capacities irrespective of the efforts by the Kolkata Municipal Corporation (KMC) in renovating the drainage pipelines (Sen 2013 ). The population increase (14.98 million in 2016) (UNDESA 2016 ), rapid urbanization and the corresponding land-use changes have substantially reduced the flood-resilient efficacy of the region in recent years.
Research methods and comparative assessment
This section briefly provides insights into major flood events in the study regions and the different methodologies adopted in various studies for elucidating flood-induced risk in the above-mentioned coastal cities (Table 2 ).
Flooding is common in the region which depends on climate, seasonality and other local factors. Five major flood events were reported since 1979, and the flood event of 2006 was devastating. Major flood events are reportedly caused due to monsoon-induced rainfall events (the peak flood is observed during August) (Agnihotri and Patel 2008 ) as well as by the emergency opening of the Ukai dam. The various factors which contribute to the flooding of Tapi River are the increase in population and allied factors such as built-up area increase, silting up of riverbed and embankment construction. Though the Ukai dam in the region was constructed with an additional aim to prevent floods, major floods during years 1998, 2004 and 2006 occurred due to the emergency opening of the dam when its capacity is overthrown (Joshi et al. 2012 ). The Khadi floods also pose a flooding threat (e.g. 2004, 2005 and 2007) in addition to the flooding of the Tapi River. Surat was selected as one of the pilot cities in the Asian cities climate resilience network in 2008. The major flood events also increase the risk of vector-borne disease in the region (Bhat et al. 2013 ). Jariwala and Samtani ( 2012 ) detailed the natural and anthropogenic cause of the flood in the southern part of Surat city, where the storm water is discharged through Mindhola River and its tributaries. Some of the anthropogenic factors listed are land reclamation on the bank of creeks, construction of bridges and suspended solids/non-degradable substances in city effluents. Natural reasons include precipitation pattern, catchment topography, bed gradients and the cross-sectional area available for flow. The peak flood is estimated from hydrological and catchment data using rational formula, and accordingly tributary cross sections were designed to contain the estimated peak flood. Jariwala and Samtani ( 2012 ) proposed the modification in the channel section of the most vulnerable stretches (Mithi and Kankara), so that it can contain peak flood waters. Joshi et al. ( 2012 ) had done urban flood mapping using geospatial techniques at Surat city. They used the Geo-Coded Indian Remote Sensing (IRS-1D) satellite image of April 2005 and Topo-Sheets at 1:50,000 scales collected from office of Survey of India and generated flood inundation map for the West Zone area of Surat City for different flood water levels. It was found that the inundation will start at water level above 4.0 m in the low-lying area in the West Zone area of Surat. The study also showed that the 95.38% area will be inundated when water level will rise at 12.0-m level.
A two-dimensional hydrodynamic model was used to simulate flooding (Ramirez and Rajasekar 2015 ). The methodology includes three stages: (1) simulation of Ukai dam release discharge reaching the city, (2) simulation of the combined effect of the tides and the dam released water, (3) delineating the vulnerable infrastructure and population. A loss and damage assessment of Surat City due to floods was carried out (Bahinipati et al. 2015 ). This study exclusively considered the textile industry which was affected during the 2006 floods. The storm drainage from the Surat city passes through various tributaries (creeks) and ultimately drains into the Mindhola River. Following the floods during 2006, flood hazards mapping of the urban floodplain of the Tapi catchment area was carried out using GIS and remote sensing techniques (Singh and Sharma 2009 ). Ukai dam release scenarios combined with tidal effects were used to run a two-dimensional flood model (CAESAR-Lisflood) using a fine spatial resolution (30 m) topography (Ramirez et al. 2016 ). Simulations were carried out for extreme conditions, and probabilistic flood risk maps were prepared for the city. The flood impact assessment and the extent of inundation in the low-lying area are carried out (Patel et al. 2017 ) using a 1D/2D coupled hydrodynamic model (HEC-RAS 5) considering two hundred and ninety-nine cross sections, five major bridges and two hydraulic structures along the river course. The model set-up considered the conditions (tidal level at the river mouth and the Ukai Dam release) during the 2006 flood as boundary conditions. The results indicated that ~ 75–77% area of the city was inundated during the flood. Areas within the city which are prone to flooding and submergence were identified using Digital Elevation Model and Geographical Information System (Patel and Dholakia 2010 ). Modelling studies incorporating climate factors are almost absent in the region. As the city is predicted to be prone to flooding induced by climate change impacts, there is a need for studies addressing this issue.
Mumbai city
The Mumbai region is prone to severe floods annually, and the flood of 2005 (Gupta 2007 ) was the worst in near history. The region is flood prone primarily due to its geography, which was shaped both by natural and by anthropogenic activities. The heavy summer monsoon rainfall together with the anthropogenic geographical alterations aggravates the flood risk. The land is heavily reclaimed and most of it is below the high-tide line, which can cause sea water entering to the complex drainage system of the city, resulting in mass inundation, as occurred during the 2005 floods. Urbanization is the most important driver of flood risk in the city. The inadequate drainage system and an ineffective spatial planning increase the flood risk in the region. There had been a surge in the number of studies regarding flood in the region following 2005 flood event. Major flood events in Mumbai are studied and compiled by Hallegatte et al. ( 2010 ). They used risk quantification following a standard catastrophe risk modelling framework combining estimates of hazard, exposure and vulnerability, which can give an estimate of economic damage and exposure of population to flood risks in various probabilities. Additional damages from the floods are also included in the framework. Simulation using climate model and further downscaling indicate high potential sensitivity of flood risk to climate change (Ranger et al. 2011 ). An urban flood model, Storm Water Management Model (SWMM), was used in the study. The land use/land cover (LULC) of the region is derived by analysing observations from the IRS LISS III satellite. Chatterjee ( 2010 ) discussed the response of the informal settlers in 2005 flood events in Mumbai. The study showed that informal settlers have to employ a combination of structural means and complex networks of assistance to recover from flood-induced risks. Ramesh et al. ( 2008 ) used extreme value analysis (EVA) to find out the hourly maximum rainfall, which is helpful in the estimation of flood levels during maximum rainfall conditions. The changes in the urban pattern (1973 and 2004) of the city were mapped using Landsat MSS and ETM+ data (Pavri 2010 ). A land-use map, using an unsupervised classification, and an elevation model using Shuttle Radar Topography Mission (SRTM) data were prepared, to identify the zones vulnerable to floods. A FEM-based flood simulation model was developed to simulate rainfall events consisting of flooding as well as non-flooding events (Shahapure et al. 2011 ). The model was integrated with the GIS and remote sensing database and could simulate the flooding event and the required detention pond. A Participatory Spatial Risks Mapping was carried out in the Dharavi region which encouraged the community participation which includes town watching and hazard mapping (Samaddar et al. 2011 ). The method was proved to be a very promising and empowering tool for local communities in tackling flood issues. An artificial neural network (ANN)-based flood management system was developed (Subramanian 2012 ), which helps users in data processing and can serve as an effective real-time flood tackling decision management system in the region due to its flexible and interactive nature. A methodology for rapid inundation mapping was developed for a data-sparse condition considering two scenarios (Gupta and Nikam 2013 ). The scenarios include continuous rainfall at 50 mm/h (the return period of 2 in 1 year) and 100 mm/h (the return period of 1 in 1 year), each for 1 h. This helped in delineating areas prone to flood water inundation due to these intensities. The flood simulation in the coastal urban region was carried out using an integrated flood assessment model in which the spatial data sets were organized by a web-based GIS framework (Kulkarni et al. 2014 ). The model could simulate the flood event during the extreme rainfall event of 2005. The spatial and temporal variation of LULC change for the coastal urban catchment area of the Mithi River was evaluated using toposheets and remote sensing data (Zope et al. 2015 ). Flood hydrographs were formulated for various conditions of LULC change and floodplain maps as well as flood hazard maps were derived. The models HEC-HMS and HEC-RAS were integrated with HEC-GeoHMS and HEC-GeoRAS for the preparation of maps. The study contends that major flooding can occur even in marginal flow conditions in the catchment when there is tidal influence. Another LULC change analysis was carried out for the Oshiwara River catchment in the region (Zope et al. 2016 ) to understand the impact of LULC change and urbanization on floods. The study found out an increase in the flood inundation area in the region.
Kolkata city
Kolkata city is prone to annual flooding during monsoon time and during extreme events such as cyclones. The city drainage network is too old, and the existing network is not sufficient for the entire city. The parts of the city were once wet lands, and the slope of the region as well as the wet land nature is posing threats to the drainage. A high-intensity rainfall event combined with high-tidal condition will always result in water-logging condition. Heavy siltation and inadequate maintenance of the sewer system have reduced the hydraulic capacity, which is also increasing the flood risks. The region is undergoing land subsidence (Chatterjee 2010 ), and this can be a boosting mechanism for future floods in the region. Presently, the population in the region has adapted to flood risks as it is not very severe and long lasting. Present adaptation strategies can change the wake of severe flood induced by climate change scenarios. There are three factors responsible for flood risks in the area: (1) natural factors, (2) developmental factor and (3) climate change factors. The natural factors include the low relief and topography, natural subsidence together with cyclones, storm surges and heavy rainfall. Unplanned urbanization, the low capacity drainage system, siltation in sewerage channels and human-induced subsidence are included in developmental factors (Dasgupta et al. 2013 ). The Kolkata Municipal Corporation (KMC) has recently introduced (September 2018) a comprehensive city-level Flood Forecasting and Early Warning System (FFEWS) making Kolkata as the first city in India having this kind of facility. This will empower the city administration and citizens in efficiently manage flood risks and damage.
An integrated management of storm water management in the region was discussed by Bose ( 2008 ). Remote Analysis of Vulnerability by Non-linearization Algorithm (RAVANA) was used to identify the extent of flood inundation in the Kolkata city (Chakraborty 2010 ). An array of automatic rain gauges and digital water-level sensors were used to monitor the rainfall distribution and water levels in the drainage channels in real time (Sen 2013 ). A framework for a real-time flood inundation forecasting model was proposed using a sewer flow–overland flow combined model, the results of which can be disseminated to the public through the internet. The flood vulnerability of the city was assessed considering intense precipitation events for return periods of 30, 50 and 100 years using rainfall data, emission scenarios and the sea-level rise for 2050 (Dasgupta et al. 2013 ). The outcome was a geographically overlaid, location-specific inundation depth and duration forecast. The study also projected the relevance of de-silting the sewers in reducing the vulnerability. The vulnerability of informal settlements in Kolkata to the flood risks indicates the dependence towards various socio-economic and infrastructure variables (Rumbach and Shirgaokar 2017 ). The study proposed that the disaster reduction policies should focus on lowest income groups. An open-source, coupled flood model having a surface model which uses a simplified numerical scheme and SWMM drainage network model was developed to study historical flooding in Kolkata (Courty 2018 ). The developed model has been proved to be a useful tool in flood risk mapping and drainage network design.
Chennai city
Chennai coastal region is prone to Tsunamis (e.g. in 2004) and cyclones; both the factors can cause coastal flooding. The flood in the region is manifested mainly by anthropogenic reasons (Drescher et al. 2007 ). Chennai lacks a natural gradient for free run-off; thus, an effective drainage system is very essential. The present drainage system is not sufficient for the growing city. The Cooum and Adyar rivers in the Chennai city also play a major role in the floods. The river banks are also heavily encroached. Chennai witnessed several catastrophic floods in recent years associated with heavy rainfall events and cyclonic depressions, which often lead to flooding of major rivers and clogging of the drainage system. A major flood event occurred in December 2015 and was reported as one of the most disastrous floods in the history of the region. The flood event affected 2 million people and caused the loss of properties worth US$ 80,000 million (Ramasamy et al. 2018 ). A surge in studies addressing the causes, effects and impacts of floods in the region was observed following the devastating flood of 2015.
Geocommunication and geovisualization were used as interactive participatory mapping tools to understand the risk due to extreme precipitation in the coastal areas of Chennai (Glaser et al. 2008 ), and the results were publically accessible through an information portal. Strategies to reduce flood risks were detailed in Gupta and Nair ( 2010 , 2011 ), which include directions such as the protection of lakes and open water bodies from encroachment and eviction of existing encroachments by governmental agencies. Flood avoidance, flood tolerance and flood resilience strategies should be combined into an integrated mode to build up flood resistance in the city. A basin model was developed by using a hydrological model based on remote sensing (HEC-GeoHMS), and the run-off due to precipitation was simulated using the HEC-HMS model in the Thirusoolam sub-watershed in Chennai (Suriya and Mudgal 2012 ). Flood hazard and flood zone mapping were also carried out, and the study showed the impacts of urbanization on hydrological processes. Different available methods to tackle the damage caused by the flood and the methodology to assess the economic loss in the Velachery region, Chennai, were discussed by Suriya et al. ( 2012 ). The methods include problem identification tasks composed of focus group discussion, stakeholder meeting, the questionnaire survey, succeeded by the data set preparation and flood damage assessment. Soft systems methodology (Rich Picture Diagrams and CATWOE analysis) was used for an integrated flood management and assessment in Adyar watershed in Chennai (Suriya and Mudgal 2012 ). A vulnerability assessment study was carried out in the region using satellite data and GIS techniques. Shoreline change rates were derived, and the Chennai coastal region was classified as a highly vulnerable zone (Mariappan and Devi 2012 ). Pairwise ranking (ranking and prioritizing a decision criteria) and force field analysis (visual identification and analysis of the forces affecting the flood situation), which are participatory action research tools used, were also used. Hydrological (HEC-HMS) and Hydraulic (HEC-RAS) modelling was used in feasibility analysis to control the flooding (Gokilashree 2015 ). The study proposed a new drainage channel in south-west Chennai, which will reduce the inundation area by 70%. Some flood mitigation and prevention strategies such as the revival of Buckingham Canal and renovating the surface water bodies in the region were proposed (Selvaraj et al. 2016 ), in addition to the call for better strategies considering future extreme scenarios. Jameson and Baud 2016 showed the influence of a variety of knowledge about the flooding in creating a more efficient flood management system in Chennai. Meteosat IR rainfall data, measured using a multispectral rainfall signature-based rainfall estimation technique, were used to monitor the 2015 flood (Mishra 2016 ), and the 2015 flood scenario was analysed using an integrated geographical information system (GIS) and multicriteria decision analysis (MCDA) method (Seenirajan et al. 2017 ). Ahmed and Kranthi ( 2018 ) found that around 18% of the Chennai Metropolitan Area was affected by the 2015 flood event, using satellite data from Landsat-8 OLI, Sentinel-1 and CartoDEM-3 R1. Arabindoo ( 2016 ) discussed the argument among environmentalists and the administration where the former targeted the haphazard urbanization as a strong reason for the floods in the city and the later considered it as an unprecedented climate anomaly in the history of the city. Sharma et al. ( 2017 ) showed the potential of global rainfall products (Tropical Rainfall Measuring Mission (TRMM)) and global forecast precipitation products (Global Ensemble Forecast System (GEFS) in successful flood mapping of the region. Methods such as map and site analysis, focus group interview and questionnaire survey were employed by Muneerudeen ( 2017 ) to compose urban and landscape design strategies for flood resilience.
Climate change impacts and flood risks
There is a growing scientific consensus that the climate is changing (Hartter et al. 2018 ), and the impacts on the coastal zone can be in the form of sea-level rise, the increase in the frequency of extreme wind events, the change of the annual frequency of winds, the increase in storm surge events frequency, higher waves, changes in wave direction and changes in coastal currents (Karambas 2015 ). These impacts can result in urban flooding events of varying intensities ultimately affecting the prevailing city metabolism. The impacts of the changing climate are exacerbated in urban areas due to the immense anthropogenic activities centred around these regions. All the cities addressed in this study have been through recent flood problems related to the changing climate. There are less studies addressing the climate change impacts on the flood events in these urban areas, and there is a knowledge gap regarding these realms.
For Surat city, various climatic factors, such as precipitation, temperature and sea level, were identified in the region which increase the flood risks and can induce coastal inundation. Climate models also indicate higher future precipitation in the region and an increase in number of rainy days. The models also predict the increase in minimum and maximum temperature of the region with a monthly average maximum temperature increase by 0.5 °C (Bhat et al. 2013 ). A Climate Change Impact Assessment using HEC-RAS model was carried out to picturize the inundation scenario in Surat (Parikh et al. 2017 ). A hypothetical situation was considered (a 50% increase in water inflow in the Tapi River) as a possible impact of climate change. The results indicated that there will be more inundated areas in the region as a result of climate change impacts.
For the city of Mumbai, previous studies indicate that the region is likely to be highly vulnerable to climate change and that the flood-prone and reclaimed land is occupied by the majority of Mumbai population (Patankar et al. 2010 ). The city is vulnerable and exposed to climate change-induced hazards stemming from sea-level rise, heavy precipitation, storm surge and tropical cyclone risks (Hallegatte et al. 2010 ). An assessment of climate change impacts in the region observed that by 2080 the likelihood of floods similar to the 2005 flood is more than double (Ranger et al. 2011 ). The climate change-induced floods can also inflict current and future economic losses in the region (Kumar et al. 2008 ), and the cost was found to be enormous. The single-value water surface method was used to find out the probable flooding for the various sea-level rise scenarios, and it is observed that the rate of coastal inundation is directly correlated with the sea-level rise (Singh and Kambekar 2017 ). Climate change-induced sea-level rise scenarios were used to study (Pramanik 2017 ) the impacts caused by these scenarios on the Mumbai coastal region and concluded that the sea-level rise can potentially reduce the drainage gradients in the city and can induce flooding conditions.
For Kolkata city, climate change factors responsible for flooding in the region comprises the sea-level rise, extreme precipitation and storm surge. Increased flooding in the region is caused by increased precipitation together with high tide and extreme storm surge. Dasgupta et al. ( 2013 ) studied the hydrological and hydraulic impacts of extreme precipitation due to climate change by 2050. The study employed three models to capture the flooding: (1) Soil and Water Assessment Tool (SWAT) (hydrological model), (2) Hydrologic Engineering Centre-River Analysis System (HEC-RAS) (hydraulic model) and (3) Storm Water Management Model (SWMM) (urban storm model), and historical rainfall data from continuous recording rain gauges for 25 years. The study urges the need for considering the climate change effects in all the future adaptation strategies for the Kolkata city. The heavy rainfall and storm surge combined with the high-tide result is heavy flooding and is a major problem for the river-side dwellers of the city (Ghosh 2015 ), which will be exacerbated in this era of climate change, and a world bank study modelled the impact of climate change on increased flooding in the region (World Bank 2011 ).
For Chennai city, there is a knowledge gap in the climate change assessment of the Chennai city since there are no major assessment studies till date. It is high time to address climate change impacts on flood risks and associated variability in the wake of present regional as well as global climate scenarios. van Oldenborgh et al. ( 2016 ) ruled out the possibility of the effect of global warming during the extreme rainfall event (caused by substantial flooding and inundation) of December 2015. This was attributed to the counteracting effect of aerosols and greenhouse gases. Some of the recent case studies about Chennai floods of December 2015 investigated the impact of climate change based on extreme rainfall data and the relationship with the global phenomenon like El-Nino and La-Nina, but the observations are contrary because of different data sets and methodologies for analysis (Krishnamurthy et al. 2018 ; Boyaj et al. 2018 ).
Flood risk and impacts on the cultural sites and monuments
The flood risks and impacts on the heritage sites and monuments in the coastal cities are a less addressed issue globally. A large number of cultural world heritage sites are concentrated near the coasts (Marzeion and Levermann 2008 ). Currently, there are lot of instances regarding disasters affecting the cultural heritage and monuments, but this realization is still not widespread (Taboroff 2003 ). The cultural heritage and monuments in historical urban areas have not received attention or support they warrant (Jigyasu 2016 ). The integration of cultural heritage and development in urban areas can result in a substantial increase in the sustainability and diversity of proposed development. This can also provide opportunities for employment and community participation ensuing social cohesion and peace (ICOMOS 2015 ). A holistic approach should be undertaken for the flood risk assessment in regions with cultural heritage structures, which must integrate physical, economic, social, and cultural vulnerabilities, along with perceived risk (Vojinovic et al. 2016 ). Cultural sites, historical infrastructure, monuments and objects of art can be affected by a wide variety of flood-associated impacts (Drdácký 2010 ). These include horizontal static pressure of the rising water, upward hydrostatic pressure, floating and displacement of objects due to the low-velocity stream movement, dynamic high-velocity stream action due to high-pressure water emerging through tiny openings, dynamic impacts of floating materials, the dynamic wave impacts, changes in soil properties and condition, saturation of objects and materials with water as a result of complete immersion in water, chemical and biological pollutants affecting historical objects and art works, barrier formation by the floating objects due to the combined uplift forces and flow, and post-flood effects such as change in internal climate resulting from high humidity, and appearance of cracks, the loss of cohesion, and volumetric changes of structures and materials due to the post-flood drying. There are several occasions of destruction of the archaeological sites and monuments due to flood effects even in the countries which devised substantially well and practical adaptation strategies to cope up with the flood damage inflicted to cultural sites and monuments. All the four cities addressed in this work are historical cities and have a significant place in global history. Currently, there is no authenticated database showing the extent of damage to the cultural sites and monuments in these cities due to past floods. Due to the absence of any ample legal framework, the vulnerability of the historical urban areas has been exacerbated during the disaster as well as the emergency period and post-disaster recovery phases (Jigyasu 2016 ). The recently released National Disaster Management Guidelines for Cultural Heritage Sites and Precincts (NDMA 2017 ) contain sufficient details pertaining to the directions for various departments, ministries and other stakeholders involved in the management of Cultural Heritage Sites and Precincts. These guidelines, if followed properly and systematically, can be very useful for the historical urban areas in India where there is recurring floods and associated impacts.
Adaptation framework for managing flood risks
Adaptation to extreme events such as coastal floods is very crucial in the present era due to the multi-facet nature of the causes and effects, which will be exacerbated due to the increasing population and climate change. The ever-changing and cross-boundary nature of flood risk (Jongman 2018 ) render adaptation strategies as very crucial in this era of fluctuating social and environmental conditions. Flood adaptation strategies are often intended to minimize impacts on various sectors such as built environment, human health, water quality and transport infrastructures (Wilby and Keenan 2012 ). The efficient and effective adaptation strategies should have the services of structures and facilities to defend flood, use solutions which are nature-based, have early warning systems, include financing schemes to tackle and reduce the economic loss due to the flood risk, practice risk-informed land planning (Jongman 2018 ). The regions located mainly in the tropics will experience the largest increase in the flooding frequency in the near future (Vitousek et al. 2017 ). Human adaptation to the environment is one of the most important processes of survival (Rossnerova et al. 2017 ) in the era of extreme floods. Adaptation measures in a developing country like India can bring in immediate community benefits (Mathew et al. 2012 ) which can result in the reduction in future flood impacts. Though there is a considerable improvement in the flood adaptation measures in India, the social and economic costs due to floods continue to increase yearly. Adaptation measures can play a pivotal role in managing flood risks in the near future in the four coastal cities addressed in this study, considering the huge population and infrastructure which are vulnerable to extreme flood conditions.
Agnihotri and Patel ( 2008 ) elucidated flood reduction plans for the Surat city by suggesting some preventive and curative measures by highlighting the need for flood water detention ponds and diversion of flood waters to other rivers. They used Kennedy’s theory for the design of the interlinking canals. A channel modification strategy for the Tapi River using HEC-RAS and Arc GIS software HECGeo was put forwarded (Agnihotri and Patel 2011 ) as a curative flood control measure, useful in the preparation of mitigation and adaptations plans. Bhat et al. ( 2013 ) addressed various strategies to aid Surat city to cope up with and adapt to flood risks. Flood adaptation uses built environment such as by raising the plinth height and urging to use the ground floor for parking purposes only. Low-income groups adopt their own methods to store valuables in the case of extreme events, for example keeping things in plastic pouches. Warnings using the help of information technology are also included in adaptation strategies. The megaphone warning mostly helps the low-income group during a possible flood event. There are plans to implement GIS-assisted warning system by geotagging residential complexes and collecting the information of vulnerable people (elderly, infirm, babies, pregnant women) during pre-monsoon time. Using qualitative interviews and public planning documents, Patel ( 2014 ) addressed adaptation strategies in the Surat city. The major flood event of 2006 and its after-effects paved the way for increased public consciousness of flood risk and need for adaptation strategies, leading to major investments by the public and private sector players in adaptation strategies and methods. Micro-level approaches for prevention and response protocol at ward level in addition to city level were also implemented specially for flood risk. Over the years, Surat city has acquired successful adaptation and resilience strategies to deal with extreme events. The ever-changing environmental conditions are a challenge to the city and thus warrant further studies regarding adaptation strategies considering future scenarios.
In Mumbai, more than half of the population live in squatter settlements, rendering this low-income group more vulnerable to the perils of the recurring floods. There was always an inertia in response by the authorities towards extreme events in the city (Johnson et al. 2015 ), which was well reflected in the 2005 Mumbai flood. The devastating flood leads to the constitution of a fact finding committee to find out the causes for the disaster and to take measures. In order to reduce future risks, the government also initiated the Mithi river bed restoration plan. The river is choked with plastic wastes and is devoid of a floodplain because of the encroachment and the illegal settlements. Due to this, the slightest unforeseen rainfall can cause flooding in the region (Vengurlekar and Patkar 2016 ). A public participatory workshop method called Yonmenkaigi System Method (YSM) was implemented in the Mumbai region, which imbibe the concerns and dimensions of the problems based on the perspective of the community (Samaddar et al. 2015 ). The method will enable the community to assess their strengths and weaknesses rather than depending on other supports, such as from civic bodies, for tackling and adapting to the flood-related problems. The adaptation strategies in the Mumbai region should identify the physical, economic and social vulnerability of the areas with poor and low-income households, small businesses and informal settlements, and adaptation should be incorporated as a main component into larger developmental goals (Patankar and Patwardhan 2016 ). Surjan and Shaw ( 2009 ) analysed the role of participatory mechanism called ALM (Advanced Locality Management) in flood risk reduction. ALM has enabled people to care the environment beyond their own household and work for the entire locality. The local government also encouraged this community effort since it reduces flood risks as well as the burden on overstressed municipal activities, which further helps in reducing water-borne diseases and epidemics in the region. These types of people or community-centred activities with the support of government can play a major role in the reduction in disaster hazard risks. Based on the data collected from informal settlements in Mumbai, Chatterjee ( 2010 ) showed different adaptation strategies taken by the population occupying these settlements. These include structural adjustments, support networks after the flood event and networks of loss redistribution. Considering the informal settlements, Subramanyam and MacAlister ( 2016 ) elaborated the prevailing gap in the perception of solutions to flooding risks between the community and local government. Micro-Small and Medium Enterprises (MSMEs), which are some of the most affected establishments during flood events, in the flood-affected areas implemented their own flood protection measures which include various structural and non-structural measures (Schaer and Pantakar 2018 ).
Kolkata’s seasonal flooding has prompted the local population to adapt a number of management strategies. However, challenges are still continuing due to the adaptation deficit in (1) sewer network, (2) drainage infrastructure and (3) financial resources. Some recommended adaptive measures are de-siltation of the existing main sewer, construction of new main sewers, extension of the drainage facility, improvement in the storm water drainage system and renovation of the outfall canal system (Dasgupta et al. 2013 ). A substantial transition in risk governance is observed in the region in recent times, and the flood risks are managed more efficiently and in a responsive mode (Parasuram et al. 2016 ). For example, pumps are deployed immediately after heavy rainfall events to drain the flood water.
In Chennai, urbanization coupled with climate variability turned out as a prominent reason for flood-induced risks, which was well reflected in the recent 2015 flood events. An integrated approach is essential to avert the vagaries of extreme flood events. This approach should combine watershed and land-use management with planning of development, engineering precautions, flood preparedness and emergency management in affected areas (Lavanya 2012 ). Local adaptation strategies are essential to bring about flood resilience in the region (Tajuddin 2018 ), by constructing socio-ecological resilience at the neighbourhood level. Geotagging and text mining techniques were used to understand the resilience of infrastructure using 2015 flood data (Chong et al. 2018 ), and social media is found to be very useful in evaluating the infrastructure resiliency (Yadav and Rahman 2016 ; Gunessee et al. 2017 ) and flood adaptation (Nair et al. 2017 ). Locality-specific, culturally relevant green social work which was implemented in the region proved to be a better adaptation strategy to tackle the flood risks (Samuel et al. 2018 ).
Concluding remarks
This study briefly reviewed the flood risk and adaptation assessment studies in major Indian coastal cities. Four coastal cities (Surat, Mumbai, Chennai and Kolkata) were selected based on the flood risks, anthropogenic activities and vulnerability from flood hazards. This review study also identified some knowledge gaps in flood risk assessment and allied studies in Indian coastal cities. Among the four cities, Mumbai followed by Surat is most vulnerable in terms of flood risks associated with anthropogenic activities. Another factor which exacerbates flood risk in all these cities is the lack of proper drainage and sewerage system. Community-based adaptation strategies in grassroots level supported by local governments show some successes in adaptation for tackling flood risk scenarios in urban areas. Economic minorities are mostly affected by flood risk and associated problems in these cities. Future planning and management of flood risks in these cities should also include economic minorities for the sustainability of city environments. Early warning systems combined with information technology should be effectively implemented in flood risk management and adaptation strategies to make the strategies more effective and practical. Though this review has a drawback that only four cities were considered, the study can be representative of other Indian coastal cities which have similar trends in environmental and demographic settings.
Agnihotri PG, Patel JN (2008) Study of flood at Surat City and its remedial measures the 3rd IASME. In: WSEAS international conference on water resources, hydraulics and hydrology, vol 3, pp 23–25
Agnihotri PG, Patel JN (2011) Improving carrying capacity of River Tapi (Surat, India) by channel modification. Int J Adv Eng Technol 2:231–238
Google Scholar
Ahmed CF, Kranthi N (2018) Flood vulnerability assessment using geospatial techniques: Chennai, India. Indian J Sci Technol 11(6):1–13
Arabindoo P (2016) Unprecedented natures? An anatomy of the Chennai floods. City 20(6):800–821
Bahinipati CS, Rajasekar U, Acharya A, Patel M (2015) Flood-induced economic loss and damage to the textile industry in Surat City, India. Asian Cities climate resilience working paper series 26
Bai X, Dawson RJ, Ürge-Vorsatz D et al (2018) Six research priorities for cities and climate change. Nature 555:23–25. https://doi.org/10.1038/d41586-018-02409-z
Article Google Scholar
Balica SF, Wright NG, van der Meulen F (2012) A flood vulnerability index for coastal cities and its use in assessing climate change impacts. Nat Hazards 64:73–105
Bhat GK, Karanth A, Dashora L, Rajasekar U (2013) Addressing flooding in the city of Surat beyond its boundaries. Environ Urban 25:1–13
Bose S (2008) Adaptive and integrated management of wastewater and storm water drainage in Kolkata—case study of a Mega City. In: Pahl Wostl C, Kabat P, Moltgen J (eds) Adaptive and integrated water management: coping with complexity and uncertainty. Springer, Heidelberg, New York, Berlin, pp 341–355
Boyaj A, Ashok K, Ghosh S, Devanand A, Dandu G (2018) The Chennai extreme rainfall event in 2015: the Bay of Bengal connection. Clim Dynam 50(7–8):2867–2879
Chakraborty T (2010) Flood inundation study of Kolkata Metro City with remote analysis of vulnerability by non-linearization algorithm (RAVANA). Doctoral dissertation, Jadavpur University
Chatterjee M (2010) Slum dwellers response to flooding events in the megacities of India. Mitig Adapt Strateg Glob Change 15:337–353
Chong WK, Naganathan H, Liu H, Ariaratnam S, Kim J (2018) Understanding infrastructure resiliency in Chennai, India using Twitter’s Geotags and texts: a preliminary study. Engineering 4(2):218–223
Courty L (2018) Integrated modelling of overland flows and drainage networks in an urban environment. Thesis
Creel L (2003) Ripple effects: population and coastal regions. Population Reference Bureau, Washington, DC
Cross JA (2001) Megacities and small towns: different perspectives on hazard vulnerability. Gob Environ Change 3:63–80
Da Cunha, Jose G (1902) The origin of Bombay (Vol. 20). Society’s library
Dasgupta S, Gosain AK, Rao S, Roy S, Sarraf M (2013) A megacity in a changing climate: the case of Kolkata. Clim Change 116:747–766
de Oliveira JAP, Doll CN (2016) Governance and networks for health co-benefits of climate change mitigation: lessons from two Indian cities. Environ Int 97:146–154
Drdácký MF (2010) Flood damage to historic buildings and structures. J Perform Constr Fac 24(5):439–445
Drescher A, Glaser R, Pfeiffer C, Vencatesan J, Schliermann-Kraus E, Glaser S, Dostal P (2007) Risk assessment of extreme precipitation in the coastal areas of Chennai as an element of catastrophe prevention. In: Forum DKKV/CEDIM: disaster reduction in climate change
Füssel HM, Klein RJ (2006) Climate change vulnerability assessments: an evolution of conceptual thinking. Clim Change 75:301–329
Ghosh A (2015) Kolkata and climate change. Clim Change Policy Pap IV WWF 4:1–20
Glaser S, Glaser R, Drescher A, Pfeiffer C, Schliermann-Kraus E, Lechner M, Vencatesan J (2008) Geo-communication for risk assessment and catastrophe prevention of flood events in the coastal areas of Chennai. In: Sànchez-Marrè M, Béjar J, Comas J, Rizzoli A (eds) iEMSs 2008: International Congress on Environmental Modelling and Software Integrating Sciences and Information Technology for Environmental Assessment and Decision Making 4th Biennial Meeting of iEMSs. International Environmental Modelling and Software Society
Gokilashree R (2015) A feasibility analysis on new drainage channel to control flooding in Southwest Chennai using hydraulic model. J Water Resour Eng Manag 2(1):1–7
Gunessee S, Subramanian N, Roscoe S, Ramanathan J (2017) The social preferences of local citizens and spontaneous volunteerism during disaster relief operations. Int J Prod Res 16:1–6
Gupta V (1987) Tourism in India. Gyan Publishing House, New Delhi
Gupta K (2007) Urban flood resilience planning and management and lessons for the future: a case study of Mumbai, India. Urban Water J 4(3):183–194
Gupta AK (2009) Urban floods: case study of Mumbai. Disaster Dev 3(2):99
Gupta AK, Nair SS (2010) Flood risk and context of land-uses: Chennai city case. J Geogr Reg Plan 3:365–372
Gupta AK, Nair SS (2011) Urban floods in Bangalore and Chennai: risk management challenges and lessons for sustainable urban ecology. Curr Sci 10:1638–1645
Gupta K, Nikam V (2013) A methodology for rapid inundation mapping for a megacity with sparse data: case of Mumbai, India. IAHS-AISH publication 385-391
Hallegatte S et al (2010) Flood risks, climate change impacts and adaptation benefits in Mumbai: an initial assessment of socio-economic consequences of present and climate change induced flood risks and of possible adaptation options. OECD environment working papers, no. 27. OECD Publishing
Hartter J, Hamilton LC, Boag AE, Stevens FR, Ducey MJ, Christoffersen ND, Oester PT, Palace MW (2018) Does it matter if people think climate change is human caused? Clim Serv 10:53–62
Hunt A, Watkiss P (2011) Climate change impacts and adaptation in cities: a review of the literature. Clim Change 104:13–49
ICOMOS (2015) Cultural heritage, the UN sustainable development goals, and the new urban agenda. In: ICOMOS concept note for the United Nations post-2015 agenda and the third United Nations conference on housing and sustainable urban development (HABITAT III)
IYB (2007) India year book. Government of India, New Delhi
Jaiswal N, Kishtawal CM, Bhomia S (2018) Similarity-based multi-model ensemble approach for 1–15-day advance prediction of monsoon rainfall over India. Theor Appl Climatol 132:639–645. https://doi.org/10.1007/s00704-017-2109-6
Jameson S, Baud IS (2016) Varieties of knowledge for assembling an urban flood management governance configuration in Chennai, India. Habitat Int 54:112–123
Jariwala RC, Samtani BK (2012) The estimation of flood and its control by section modification in Mithi and Kankara tributaries at Surat. Int J Eng Res Appl 2:862–867
Jigyasu R (2016) Reducing disaster risks to urban cultural heritage: global challenges and opportunities. J Herit Manag 1(1):59–67
Joerin J, Shaw R, Takeuchi Y, Krishnamurthy R (2012) Assessing community resilience to climate-related disasters in Chennai, India. Int J Disaster Risk Reduct 1:44–54
Johnson C, Toly N, Schroeder H (eds) (2015) The urban climate challenge: rethinking the role of cities in the global climate regime. Routledge, London
Jongman B (2018) Effective adaptation to rising flood risk. Nat Commun 9(1):1986
Joshi PM, Sherasia NK, Patel DP (2012) Urban flood mapping by geospatial technique a case study of Surat City. IOSR J. Eng 2:43–51
Kale VS, Ely LL, Enzel Y, Baker VR (1996) Palaeo and historical flood hydrology, Indian Peninsula. Geol Soc Lond Spec Publ 115:155–163. https://doi.org/10.1144/GSL.SP.1996.115.01.12
Karambas TV (2015) Modelling of climate change impacts on coastal flooding/erosion, ports and coastal defence structures. Desalin Water Treat 54(8):2130–2137
Kosambi M, Brush JE (1988) Three colonial port cities in India. Geograph Rev 78(1):32–47
Krishnamurthy L, Vecchi GA, Yang X, van der Wiel K, Balaji V, Kapnick SB, Underwood S (2018) Causes and probability of occurrence of extreme precipitation events like Chennai 2015. J Clim 31(10):3831–3848
Kulkarni AT, Mohanty J, Eldho TI, Rao EP, Mohan BK (2014) A web GIS based integrated flood assessment modeling tool for coastal urban watersheds. Comput Geosci 64:7–14
Kumar R, Jawale P, Tandon S (2008) Economic impact of climate change on Mumbai, India. Reg Health Forum 12:38–42
Lavanya AK (2012) Urban flood management—a case study of Chennai City. Archit Res 2:115–121
Lindley SJ, Handley JF, Theuray N, Peet E, McEvoy D (2006) Adaptation strategies for climate change in the urban environment: assessing climate change related risk in UK urban areas. J Risk Res 9:543–568
Mahmood S, Davie J, Jermey P et al (2018) Indian monsoon data assimilation and analysis regional reanalysis: configuration and performance. Atmos Sci Lett 19:3. https://doi.org/10.1002/asl.808
Mariappan VN, Devi RS (2012) Chennai coast vulnerability assessment using optical satellite data and GIS techniques. Int J Remote Sens 1:175–182
Marzeion B, Levermann A (2008) Loss of cultural world heritage and currently inhabited places to sea-level rise. Environ Res Lett 9(3):034001
Mathew S, Trück S, Henderson-Sellers A (2012) Kochi, India case study of climate adaptation to floods: ranking local government investment options. Glob Environ Change 22(1):308–319
MCGM (2014) Greater Mumbai city development plan 2005–2025. Municipal Corporation of Greater Mumbai, Mumbai
Mendelsohn R, Emanuel K, Chonabayashi S, Bakkensen L (2012) The impact of climate change on global tropical cyclone damage. Nat Clim Change 2(3):205
Mishra AK (2016) Monitoring Tamil Nadu flood of 2015 using satellite remote sensing. Nat Hazards 82(2):1431–1434
Muneerudeen A (2017) Urban and landscape design strategies for flood resilience in Chennai city. Master’s thesis
Murphey R (1964) The city in the swamp: aspects of the site and early growth of Calcutta. Geogr J 130:241–256
Nair MR, Ramya GR, Sivakumar PB (2017) Usage and analysis of Twitter during 2015 Chennai flood towards disaster management. Procedia Comput Sci 115:350–358
NDMA (2017) National disaster management guidelines for cultural heritage sites and precincts, September 2017. New Delhi A publication of the National Disaster Management Authority, Government of India
Nicholls RJ, Small C (2002) Improved estimates of coastal population and exposure to hazards released. Eos 83:301–305
Nicholls RJ et al (2008) Ranking port cities with high exposure and vulnerability to climate extremes: exposure estimates. OECD environment working papers, no. 1. OECD Publishing
Pacione M (2006) City profile Mumbai. Cities 23:229–238
Parasuram P, Narayanan P, Pelling M, Solecki W, Ramachandran P, Ramachandran R (2016) Climate change adaptation pathways in Kolkata. J Extreme Events 3(03):1–22
Parikh K, Parikh J, Kumar M (2017) Vulnerability of Surat, Gujarat to flooding from Tapi River: a climate change impact assessment. Vayu Mandal 43(2):120–129
Patankar A, Patwardhan A (2016) Estimating the uninsured losses due to extreme weather events and implications for informal sector vulnerability: a case study of Mumbai, India. Nat Hazards 80(1):285–310
Patankar A, Patwardhan A, Andharia J, Lakhani V (2010) Mumbai city report. In: International workshop on climate change vulnerability assessment and urban development planning for Asian coastal cities
Patel T (2014) Funding for adaptation to climate change: the case of Surat. Doctoral dissertation, Massachusetts Institute of Technology
Patel DP, Dholakia MB (2010) Identifying probable submergence area of Surat City using digital elevation model and geographical information system. World Appl Sci J 9(4):461–466
Patel DP, Ramirez JA, Srivastava PK et al (2017) Assessment of flood inundation mapping of Surat city by coupled 1D/2D hydrodynamic modeling: a case application of the new HEC-RAS 5. Nat Hazards 89:93–130. https://doi.org/10.1007/s11069-017-2956-6
Pavri F (2010) Urban expansion and sea-level rise related flood vulnerability for Mumbai (Bombay), India using remotely sensed data. In: Showalter P, Lu Y (eds) Geospatial techniques in urban hazard and disaster analysis. Springer, Dordrecht, pp 31–49
Pramanik MK (2017) Impacts of predicted sea level rise on land use/land cover categories of the adjacent coastal areas of Mumbai megacity, India. Environ Dev Sustain 19(4):1343–1366
Rahmstorf S (2017) Rising hazard of storm-surge flooding. Proc Natl Acad Sci 114(45):11806–11808
Ramasamy SM, Vijay A, Dhinesh S (2018) Geo-anthropogenic aberrations and Chennai floods: 2015, India. Nat Hazards 92(1):443–477
Ramesh C, Vivekanandan N, Surwade KB, Bapat AD, Govindan S, Mathew FT (2008) Extreme value analysis of rainfall in Mumbai region to aid estimation of severe flood. ISH J Hydraul Eng 14(2):102–117
Ramirez J, Rajasekar U (2015) Modelling flood impact in Surat, India. In: TIFAC-IDRiM conference, New Delhi, India
Ramirez J, Rajasekar U, Coulthard T, Keiler M (2016) Probabilistic mapping of urban flood risk: application to extreme events in Surat, India. In: EGU general assembly conference abstracts, vol 18, p 2093
Ranger N, Hallegatte S, Bhattacharya S, Bachu M, Priya S, Dhore K, Corfee-Morlot J (2011) An assessment of the potential impact of climate change on flood risk in Mumbai. Clim Change 104:139–167
Rawlinson HG (1920) British beginnings in Western India, 1579–1657: an account of the early days of the British factory of Surat. Clarendon Press, Oxford
Revi A (2008) Climate change risk: an adaptation and mitigation agenda for Indian cities. Environ Urban 20:207–229
Rodriguez JA, Fernandez FJ, Arboleya P (2018) Study of the architecture of a smart city. Proceedings 2(23):1485
Rossnerova A, Pokorna M, Svecova V, Sram RJ, Topinka J, Zölzer F, Rossner P (2017) Adaptation of the human population to the environment: current knowledge, clues from Czech cytogenetic and “omics” biomonitoring studies and possible mechanisms. Mutat Res Rev Mutat 773:188–203
Rumbach A (2014) Do new towns increase disaster risk? Evidence from Kolkata, India. Habitat Int 43:117–124
Rumbach A, Shirgaokar M (2017) Predictors of household exposure to monsoon rain hazards in informal settlements. Nat Hazards 85(2):709–728
Samaddar S, Chatterjee R, Misra BA, Tatano H (2011) Participatory risk mapping for identifying spatial risks in flood prone slum areas, Mumbai. Annu Disaster Prev Res Inst Kyoto Univ 54B
Samaddar S, Choi J, Misra BA, Tatano H (2015) Insights on social learning and collaborative action plan development for disaster risk reduction: practicing Yonmenkaigi system method (YSM) in flood-prone Mumbai. Nat Hazards 75(2):1531–1554
Samuel M, Annadurai P, Sankarakrishnan S (2018) The 2015 Chennai floods: green social work, an emerging model for practice in India. The Routledge handbook of green social work, pp 281–292
Schaer C, Pantakar A (2018) Promoting private sector engagement in climate change adaptation and flood resilience—a case study of innovative approaches applied by MSMEs in Mumbai, India. In: Alves F, Leal Filho W, Azeiteiro U (eds) Theory and practice of climate adaptation. Springer, pp 175–191
Schaer C, Bee S, Kuruppu N (2018) Developing the business case for adaptation in agriculture: case studies from the adaptation mitigation readiness project. Private-sector action in adaptation: perspectives on the role of micro, small and medium size enterprises, p 51
Seenirajan M, Natarajan M, Thangaraj R, Bagyaraj M (2017) Study and analysis of Chennai flood 2015 using GIS and multicriteria technique. J Geogr Inf Syst 9(02):126
Sekovski I, Newton A, Dennison WC (2012) Megacities in the coastal zone: using a driver-pressure-state-impact-response framework to address complex environmental problems. Estuar Coast Shelf Sci 96:48–59
Selvaraj K, Pandiyan J, Yoganandan V, Agoramoorthy G (2016) India contemplates climate change concerns after floods ravaged the coastal city of Chennai. Ocean Coast Manage 129:10–14
Sen D (2013) Real-time rainfall monitoring and flood inundation forecasting for the city of Kolkata. ISH J Hydraul Eng 19(2):137–144
Shahapure SS, Eldho TI, Rao EP (2011) Flood simulation in an urban catchment of Navi Mumbai City with detention pond and tidal effects using FEM, GIS, and remote sensing. J Waterw Port Coast Ocean Eng 137:286–299
Shahi NK, Rai S, Mishra N (2018) Recent predictors of Indian summer monsoon based on Indian and Pacific Ocean SST. Meteorol Atmos Phys. https://doi.org/10.1007/s00703-018-0585-6
Sharma VK, Mishra N, Shukla AK, Yadav A, Rao GS, Bhanumurthy V (2017) Satellite data planning for flood mapping activities based on high rainfall events generated using TRMM, GEFS and disaster news. Ann GIS 23(2):131–140
Singh PD, Kambekar AR (2017) Assessing impact of sea level rise along the coastline of Mumbai City using geographic information system. In: Seta F, Biswas A, Khare A, Sen J (eds) Understanding built environment. Springer, pp 87–96
Singh AK, Sharma AK (2009) GIS and a remote sensing-based approach for urban flood-plain mapping for the Tapi catchment, vol 331. IAHS Publication, Wallingford, p 389
Small C, Nicholls RJ (2003) A global analysis of human settlement in coastal zones. J Coast Res 19:584–599
SMC (2011) Surat City resilience strategy. Surat Municipal Corporation, Surat
Solecki W, Rosenzweig C, Dhakal S et al (2018) City transformations in a 1.5 °C warmer world. Nat Clim Change 8:177–181
Stephen A (2016) Natural disasters: Are they really natural? In: Proceedings of ICSSR sponsored national seminar on infrastructural development and environment: issues and challenges
Subramanian R (2012) Implementation of neural networks in flood forecasting. Int J Sci Res Publ 2(10):1–3
Subramanyam N, MacAlister C (2016) Governance of flooding risks in informal settlements in satellite cities in the Mumbai region. South Asian Water Stud 6(1):12–27
Suriya S, Mudgal BV (2012) Impact of urbanization on flooding: the Thirusoolam sub watershed—a case study. J Hydrol 412:210–219
Suriya S, Mudgal BV, Nelliyat P (2012) Flood damage assessment of an urban area in Chennai, India, part I: methodology. Nat Hazards 62:149–167
Surjan A, Shaw R (2009) Enhancing disaster resilience through local environment management: case of Mumbai, India. Disaster Prev Manage 18:418–433
Taboroff J (2003) Natural disasters and urban cultural heritage: a reassessment. In: Kreimer A, Arnold M, Carlin A (eds) Building safer cities: the future of disaster risk. The World Bank, Disaster Risk Management, Series no. 3, 2003, pp. 233–240
Tajuddin N (2018) Leveraging socio-cultural networks: local adaptation strategies to bring about flood resilience in Chennai metropolitan area, India. Thesis TU Delft Architecture and the Built Environment
UN (2008) Urban agglomerations 2007. United Nations Department of Economic and Social Affairs, Population Division, New York
UNDESA (2016) The world’s cities in 2016. United Nations, Department of Economic and Social Affairs, Population Division, New York, p 392
UNESCO (2009) World water assessment programme (United Nations). Water in a changing world. UNESCO, Paris
Ürge-Vorsatz D, Rosenzweig C, Dawson RJ et al (2018) Locking in positive climate responses in cities. Nat Clim Change 8:174–177
Van Oldenborgh GJ, Otto FE, Haustein K, AchutaRao K (2016) The heavy precipitation event of December 2015 in Chennai, India. Bull Am Meteorol Soc 97(12):87–91
Vaz E (2014) Managing urban coastal areas through landscape metrics: an assessment of Mumbai’s mangrove system. Ocean Coast Manage 98:27–37
Vengurlekar M, Patkar S (2016) Mithi river regeneration. International Journal of Research in Advent Technology, Special Issue RDACE, March 2016, 90–96, Special Issue National Conference VishwaCon’16, 19 March 2016
VishnuRadhan R, Vethamony P, Zainudin Z, Kumar KV (2014) Waste assimilative capacity of coastal waters along Mumbai Mega City, West Coast of India using MIKE-21 and WASP simulation models. CLEAN 42:295–305
Vitousek S, Barnard PL, Fletcher CH et al (2017) Doubling of coastal flooding frequency within decades due to sea-level rise. Sci Rep 7:1399
Vojinovic Z, Hammond M, Golub D, Hirunsalee S, Weesakul S, Meesuk V, Medina N, Sanchez A, Kumara S, Abbott M (2016) Holistic approach to flood risk assessment in areas with cultural heritage: a practical application in Ayutthaya, Thailand. Nat Hazards 81(1):589–616
Von Glasow R, Jickells TD, Baklanov A, Carmichael GR, Church TM, Gallardo L, Zhu T (2013) Megacities and large urban agglomerations in the coastal zone: interactions between atmosphere, land, and marine ecosystems. Ambio 42:13–28
Wilby RL, Keenan R (2012) Adapting to flood risk under climate change. Prog Phys Geogr 36(3):348–378
World Bank (2011) Vulnerability of Kolkata metropolitan area to increased precipitation in a changing climate environment, climate change and water resources department South Asia region. World Bank, Washington, DC
Yadav M, Rahman Z (2016) The social role of social media: the case of Chennai rains-2015. Soc Netw Anal Min 6(1):101
Zope PE, Eldho TI, Jothiprakash V (2015) Impacts of urbanization on flooding of a coastal urban catchment: a case study of Mumbai City, India. Nat Hazards 75(1):887–908
Zope PE, Eldho TI, Jothiprakash V (2016) Impacts of land use–land cover change and urbanization on flooding: a case study of Oshiwara River Basin in Mumbai, India. CATENA 145:142–154
Download references
Acknowledgements
RD acknowledges the Ministry of Human Resource Development (MHRD) of Govt. of India for providing funding under Teaching Assistantship category. RVR acknowledges SERB-National Post-Doctoral fellowship (PDF/2017/002093) from Science and Engineering Research Board, Department of Science and Technology, Government of India. The authors thank Dr. Rohit Jigyasu, President, ICOMOS India, and members of National Scientific Committee, Risk Preparedness, ICOMOS India, for inputs.
Author information
Ravinder Dhiman and Renjith VishnuRadhan have contributed equally to this work.
Authors and Affiliations
Centre for Urban Science and Engineering, Indian Institute of Technology Bombay, Mumbai, 400076, India
Ravinder Dhiman
Department of Civil Engineering, Indian Institute of Technology Bombay, Powai, Mumbai, Maharashtra, 400076, India
Renjith VishnuRadhan & T. I. Eldho
Centre of Studies in Resources Engineering, Indian Institute of Technology Bombay, Mumbai, 400076, India
Arun Inamdar
You can also search for this author in PubMed Google Scholar
Corresponding author
Correspondence to Renjith VishnuRadhan .
Additional information
Publisher’s note.
Springer Nature remains neutral with regard to jurisdictional claims in published maps and institutional affiliations.
Rights and permissions
Open Access This article is distributed under the terms of the Creative Commons Attribution 4.0 International License ( http://creativecommons.org/licenses/by/4.0/ ), which permits unrestricted use, distribution, and reproduction in any medium, provided you give appropriate credit to the original author(s) and the source, provide a link to the Creative Commons license, and indicate if changes were made.
Reprints and permissions
About this article
Dhiman, R., VishnuRadhan, R., Eldho, T.I. et al. Flood risk and adaptation in Indian coastal cities: recent scenarios. Appl Water Sci 9 , 5 (2019). https://doi.org/10.1007/s13201-018-0881-9
Download citation
Received : 03 July 2016
Accepted : 22 November 2018
Published : 04 December 2018
DOI : https://doi.org/10.1007/s13201-018-0881-9
Share this article
Anyone you share the following link with will be able to read this content:
Sorry, a shareable link is not currently available for this article.
Provided by the Springer Nature SharedIt content-sharing initiative
- Flood management
- Urban planning
- Coastal megacities
- Find a journal
- Publish with us
- Track your research
Expert analysis of record-breaking February floods
The devastating flooding across the UK in February 2020 was remarkable in terms of the combination of duration, scale and severity, according to a preliminary analysis by the UK Centre for Ecology & Hydrology. Three successive storms – Ciara on February 8 and 9, Dennis on February 15 and 16, and Jorge from February 28 to March 1 – resulted in thousands of homes and businesses being flooded as well as widespread disruption to transport and other services.
Our briefing note provides an in-depth, initial hydrological summary of the record-breaking February floods. Highlighted findings include:
- It was the wettest February on a UK scale since records began in 1910, receiving more than twice the average rainfall, and the fourth wettest of any month in that timeframe. Around 180mm of rain fell in two days in parts of Cumbria during Storm Ciara
- New February peak flow records were established on many rivers, contributing to the highest combined flow from all rivers in England for any month on record
- As a result of storms Ciara and Dennis, more than 3,300 properties were flooded in England. In the Welsh county of Rhonda alone, 1,000 properties were flooded during Storm Dennis.
Persistent heavy rainfall resulting from Storm Ciara on February 8/9 triggered exceptionally high peak river flows, most notably across northern England in many of the same areas that suffered from flooding in November 2019 and winter 2015/2016. A week later, Storm Dennis generated similarly extreme high river flows, predominantly affecting Wales and central England.
February was a truly remarkable month in hydrological terms, with record-breaking rainfall and river flows - Dr Simon Parry
The duration and severity of the high river flows resulted in extensive property flooding, most notably from the rivers Calder, Aire, Teme, Wye, Severn and Taff. However, the total number of properties affected across the UK was significantly lower than in previous notable flood events in winter 2015/16, summer 2012 and summer 2007. The Environment Agency says flood defences protected 128,000 homes across England in February 2020. Dr Simon Parry, one of the UKCEH hydrologists who put together the preliminary analysis of the flooding, says: “February was a truly remarkable month in hydrological terms, with record-breaking rainfall and river flows caused by storms Clara, Dennis and Jorge, leading to significant flooding across much of the UK. “The significant rainfall in the previous nine months may also have contributed to the extensive flooding, because soils were saturated and could not retain as much water.”
He points out that it is challenging to collate information on the impacts of extreme events in a very short period of time, and says more comprehensive assessments will follow in the coming months.
The Environment Agency , Natural Resources Wales , Scottish Environment Protection Agency and Northern Ireland Department for Infrastructure - Rivers have been continuing to manage the impacts of the flooding, and Dr Parry thanked these authorities for providing river flow data and feedback under difficult circumstances. Our preliminary analysis is available at https://nrfa.ceh.ac.uk/sites/default/files/Briefing_Note_V6.pdf
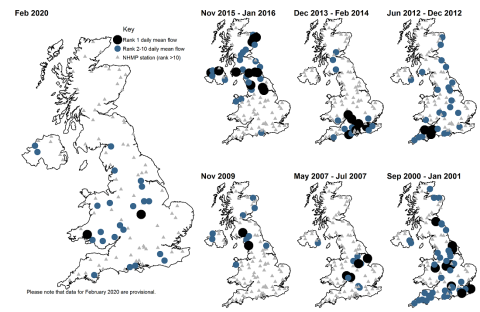
Maps showing record mean river flows for recent notable flooding events
- 0 Shopping Cart
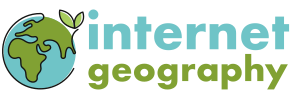
UK Floods Case Study November 2019
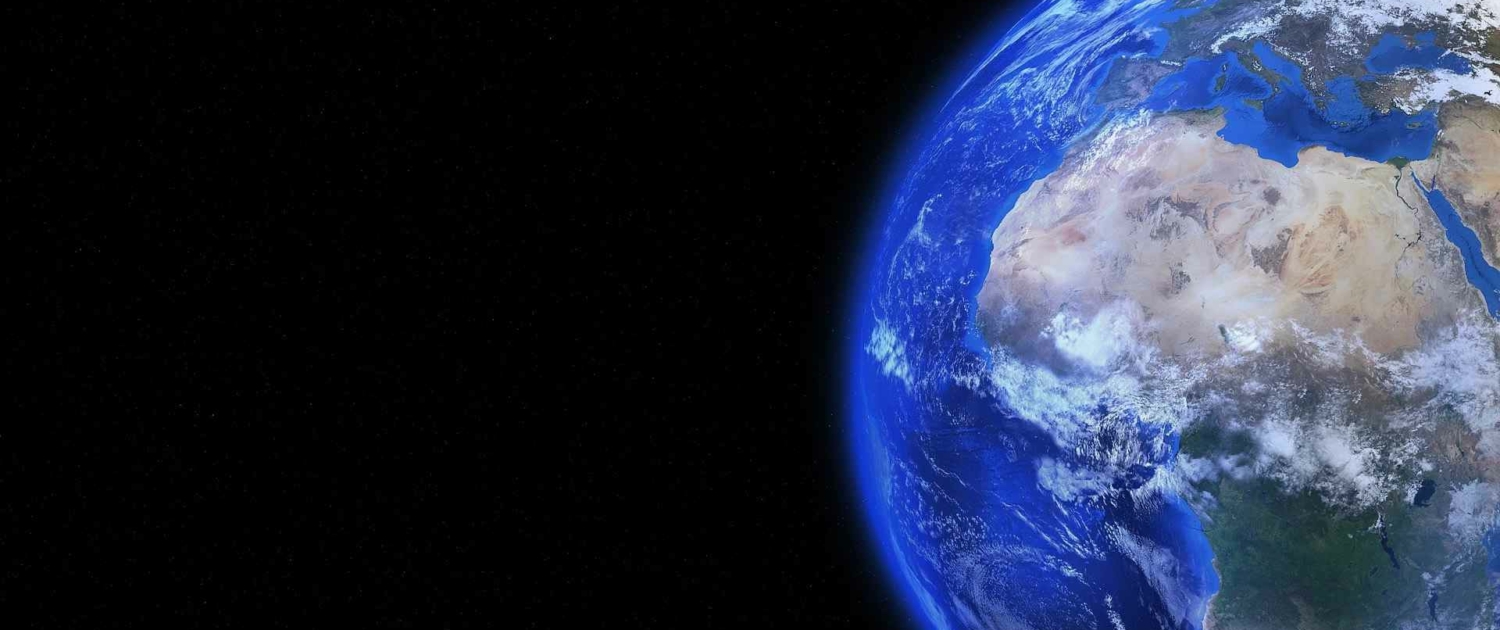
The UK experienced an extreme weather event in November 2019 when exceptionally heavy rainfall caused flooding in parts of the UK. Heavy downpours across large parts of northern England led to surface water and river flooding in parts of Yorkshire, Nottinghamshire, Greater Manchester, Derbyshire and Lincolnshire.
According to the Met Office, on Thursday 7th November 2019 over half of the average rainfall for the whole of November fell in parts of the Midlands and Yorkshire.
If you have images and/or videos of flooding or an eye witness account that you would be happy to share on an interactive flood impact map we are developing please send them [email protected]
What caused flooding in the UK in November 2019?
A large area of prolonged rainfall fell on parts of the UK in November 2019. Some areas experienced the whole of Novembers average rainfall over a period of 24 hours. Sheffield experienced 84mm of rainfall. The rainfall was caused by an area low pressure stalling over the UK.
Further reading/watching:
BBC Weather Overview
What were the effects the extreme weather in November 2019?
About 500 homes have been flooded in Doncaster with more than 1,000 properties evacuated in areas hit by the floods.
South Yorkshire Fire and Rescue said it had declared a major incident on the evening of Friday 8th November and firefighters rescued more than 40 people from the Fishlake area, near Doncaster. Residents of Fishlake said it was the first time the village had flooded in 100 years.
Empty coffins were seen floating inside the workshop of a flooded funeral parlour in the village.
Some villagers had to spend the night at a nearby pub, where staff said they had seen people crying because of the devastation.
The village church is collecting food to distribute to residents and roast dinners were delivered on Sunday to those who had remained in their homes.
Reseidents have complained that the River Don has not been dredged recently.
According to the BBC, Adrian Gill, a flood manager at the Environment Agency, said did not currently dredge the River Don “because we don’t think that’s the right thing to do” but the situation could be reviewed in the future.
Water sports enthusiast and teacher Mark Ibbotson, from Doncaster, said he, along with his 13-year-old son Logan, had rescued more than 30 people – including two babies – from a number of streets using his inflatable boat in Bentley where homes have been hit by flooding.
One of the most severely hit areas has been Bentley in Doncaster, where flooding affected many homes 12 years ago.
One resident told BBC Radio Sheffield: “The worry is our insurance policies are expensive as it is because of the 2007 floods, so now we’re all worried whether we’re going to get reinsured.”
Extensive flooding affected Rotherham , where residents were told to stay at home and not leave unless asked to do so by emergency services. Some have been taken to safety by boats.
Dozens of people were forced to spend the night in the Meadowhall shopping centre .
In Derbyshire, the River Derwent at Chatsworth reached its highest recorded level and council workers put up sandbags around Matlock and Matlock Bath, where the river was “dangerously high” .
A number of properties in Derby city centre were flooded, however, a full evacuation was not ordered as the River Derwent didn’t burst its banks to the extent emergency services believed it would.
The A52 – the main road route into Derby – was closed westbound between the city and the M1 along with a handful of smaller roads in the county.
Residents from 12 homes in Mansfield, Nottinghamshire, were unable to return home after a mudslide on Thursday led to 35 properties being evacuated .
In Nottinghamshire, residents living in mobile homes close to the River Trent in Newark were urged to move to higher ground.
On Friday, the floods claimed the life of a woman who was swept into the River Derwent at Rowsley in Derbyshire. Her body was found about two miles away in Darley Dale. She was named earlier as Derbyshire’s former High Sherriff Annie Hall .
Trains were cancelled in Yorkshire and parts of the East Midlands as rail routes were flooded.
BBC reporter Richard Cadey said some roads around Fishlake had been closed and the village was “effectively cut off because of flooding”. He said people on the ground had told him 90% of the homes there had been flooded.
The River Don, which flows through Sheffield, Rotherham and Doncaster, hit its highest recorded level at just over 6.3m (21ft), higher than it was in 2007 when it also flooded.
Sources:
England flooding: River warnings and rail delays continue
Flooding in pictures/videos
Torrential downpours flood parts of northern England – BBC
Flooding in Yorkshire – In Pictures – The Guardian
England flooding: A tour of a flooded house in Fishlake
River Derwent Flooding – Drone Video
Helicopter captures footage of flooded South Yorkshire
What were the responses to the UK floods in November 2019?
More than 100 flood warnings were put in place across England. The Environment Agency (EA) urged people to take them seriously.
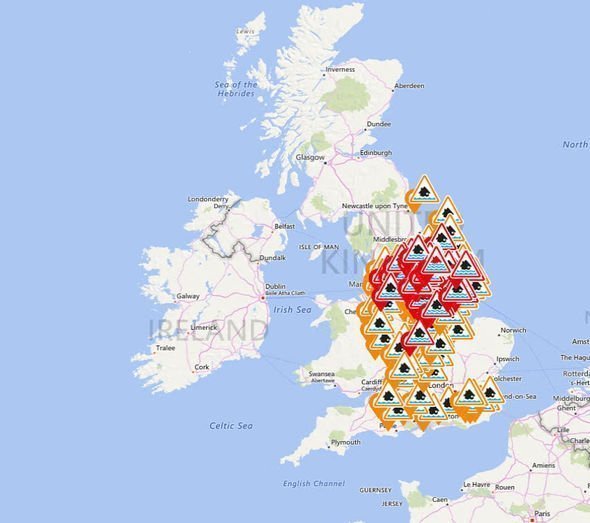
The Environment Agency took to social media to warn people about the potential impacts of flooding.
AMBER warning for flood risk today ⚠️- rain will rotate over north and north midlands bringing heavy rain on already sodden ground – take care – flood warning updates here https://t.co/K5GUW3z87V pic.twitter.com/mDDAC3uLXc — John Curtin (@johncurtinEA) November 7, 2019
The Environment Agency worked day and night to reduce the impact of flooding. The Environment Agency responded to the flood risk by working closely with police, fire and rescue, local authorities and partners to reduce the risk of flooding and keep communities safe. On the ground, Environment Agency field teams worked through the night to operate flood storage areas and pump away flood water.
A major incident was declared in South Yorkshire,
Some residents were “angry and frustrated” at Doncaster Council – claiming it had not provided sandbags early enough to prevent properties from flooding, the station reported.
Political leaders visited areas affected by floods. On the campaign trail Boris Johnson promised over £2 billion to improve flood defences.
South Yorkshire Police said it had extra officers out on patrol to “protect the evacuated areas and support those affected by the floods”.
Following a meeting of COBRA, the government’s emergency committee, Prime Minister Boris Johnson anounced the following measures :
- An extra 100 Army personnel deployed from Wednesday to support the recovery effort in South Yorkshire
- Funding for local councils where households and businesses have been affected – equivalent to £500 per eligible household
- Up to £2,500 for small and medium-sized businesses which have suffered severe impacts not covered by insurance
Six days after the heavy rain, army personnel provided support to flood-hit communities .
Environment Agency warnings
Environment Agency working day and night to reduce flood impact
How effective were the mitigation strategies introduced since the 2007 floods?
Flood defences put in place in South Yorkshire managed to significantly reduce the impact of Thursday’s floods, the Environment Agency (EA) has said.
River levels in parts of the county rose overnight to almost the same as they were in June 2007, when two people died in Millhouses and the Wicker.
Despite a major incident being declared on Thursday, the EA said the area was protected by new walls and flood gates.
The river levels around Meadowhall were high, but the EA said its defences, as well as the ones put in by Meadowhall, had lessened the damage.
Elsewhere in South Yorkshire, £3m was spent by the EA to repair and improve defences running along Ea Beck , in the villages of Toll Bar and Bentley near Doncaster.
However, people living in settlements downstream of Sheffield have complained about the impact of the recently constructed defences. In Bentley, a low-lying neighbourhood on the north side of the River Don, forlorn terraced streets are still knee-deep in water. “You don’t have to be a hydrologist to see what’s happened,” said one man interviewed by a Guardian journalist . “Sheffield built flood defences in 2015-16. They spent about £20m protecting the lower Don. So the water has nowhere to go than the next place, Rotherham and then Doncaster.” He went on to say that residents received a “red alert” on Thursday night that there was a risk of flooding. He phoned an emergency number and requested sandbags. He was told that the council was not going to distribute them because the River Don’s banks had not been breached.
When the sandbags eventually arrived the community worked together to distribute them.
South Yorkshire flooding: Defences ‘reduce impact’
Related articles:
How do you stop flooding?
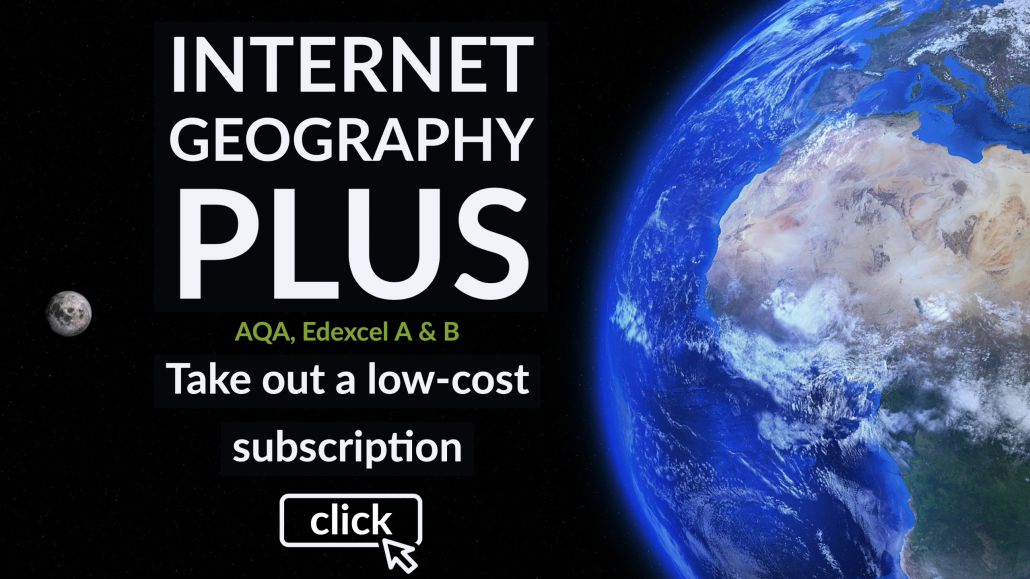
Premium Resources
Please support internet geography.
If you've found the resources on this page useful please consider making a secure donation via PayPal to support the development of the site. The site is self-funded and your support is really appreciated.
Related Topics
Use the images below to explore related GeoTopics.
Topic Home
Next topic page, share this:.
- Click to share on Twitter (Opens in new window)
- Click to share on Facebook (Opens in new window)
- Click to share on Pinterest (Opens in new window)
- Click to email a link to a friend (Opens in new window)
- Click to share on WhatsApp (Opens in new window)
- Click to print (Opens in new window)
If you've found the resources on this site useful please consider making a secure donation via PayPal to support the development of the site. The site is self-funded and your support is really appreciated.
Search Internet Geography
Top posts and pages.

Latest Blog Entries


Pin It on Pinterest
- Click to share
- Print Friendly
What caused Dubai floods? Experts cite climate change, not cloud seeding
- Medium Text
DID CLOUD SEEDING CAUSE THE STORM?
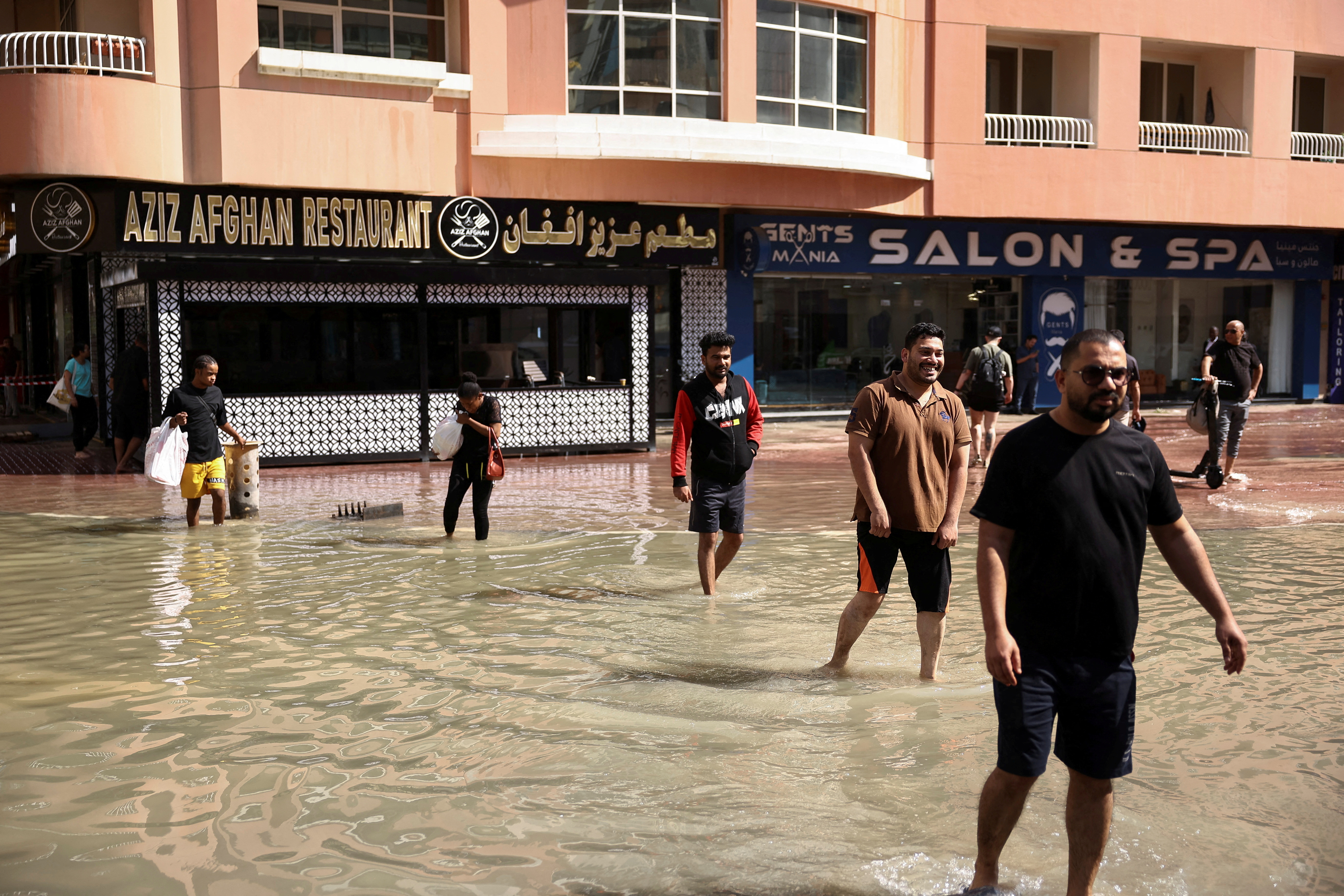
CAN'T CREATE CLOUDS FROM NOTHING
Sign up here.
Reporting by Alexander Cornwell; editing by Maha El Dahan and Alexandra Hudson
Our Standards: The Thomson Reuters Trust Principles. New Tab , opens new tab
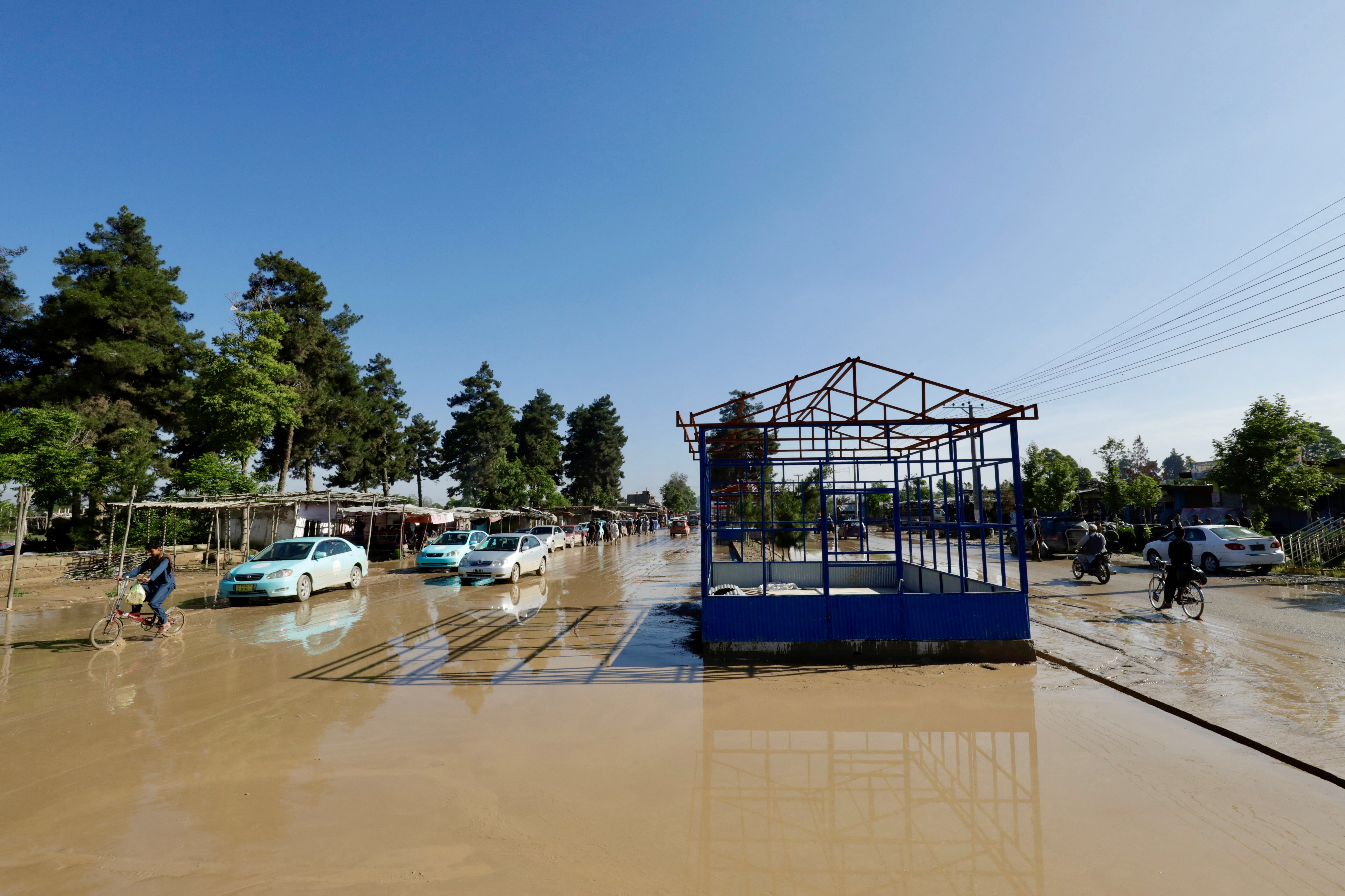
World Chevron
Search and rescue efforts were underway in South Africa on Saturday to find 11 fishermen missing at sea after their vessel sunk off the coast of Cape Town, the fisheries department said.
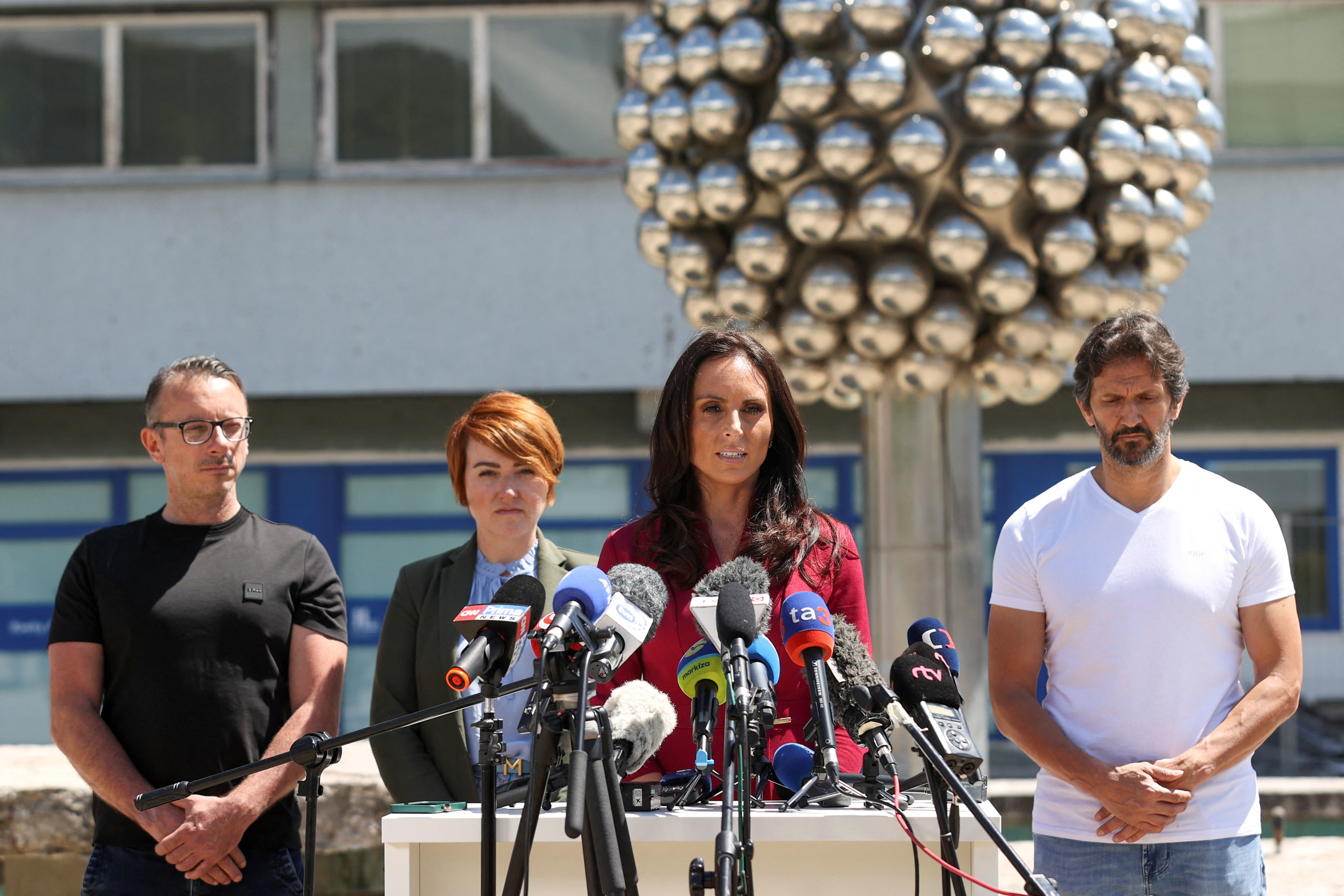
About 23 migrants were missing after setting off in a boat from Tunisia towards Italy, the country's national guard said on Saturday.
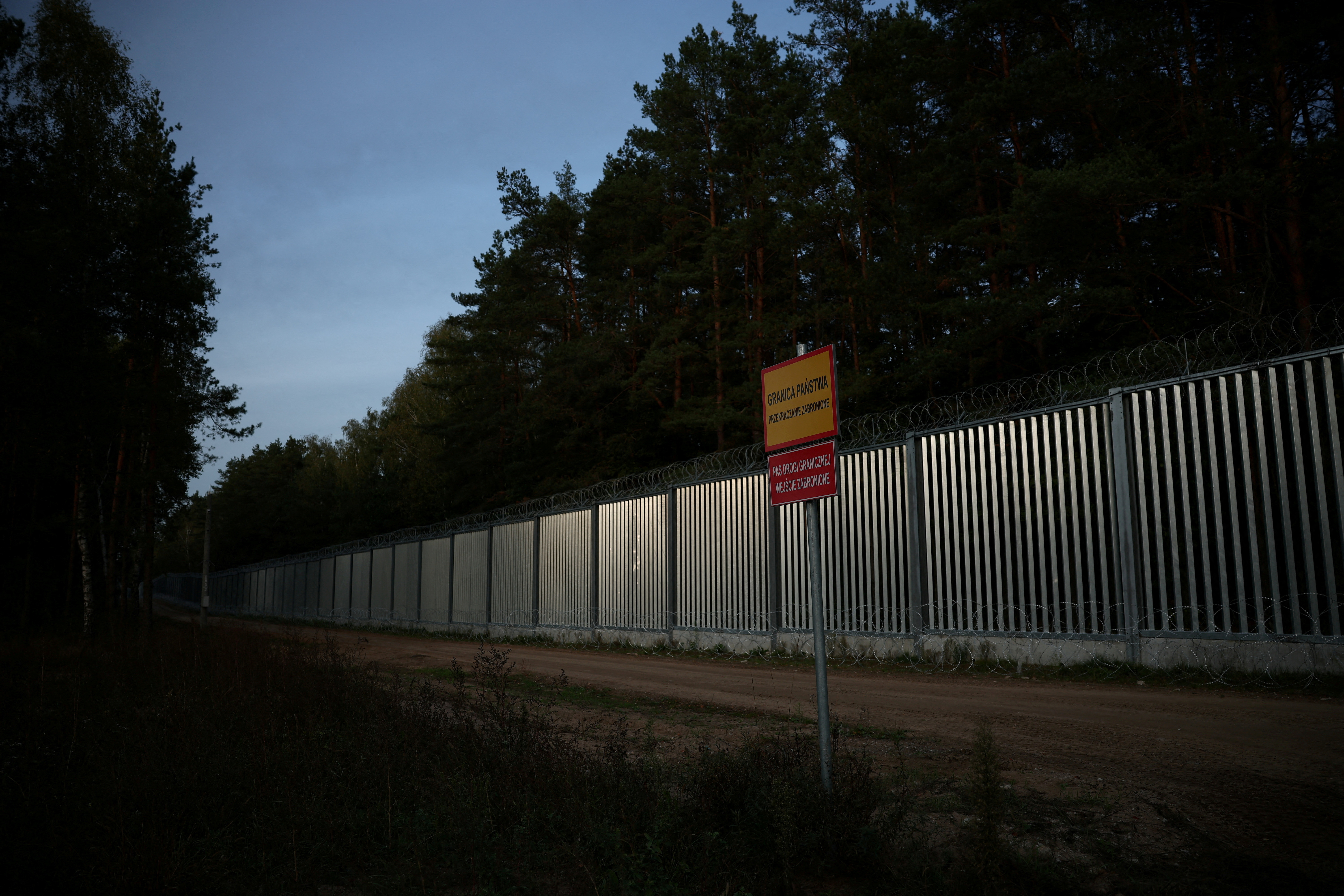
Thank you for visiting nature.com. You are using a browser version with limited support for CSS. To obtain the best experience, we recommend you use a more up to date browser (or turn off compatibility mode in Internet Explorer). In the meantime, to ensure continued support, we are displaying the site without styles and JavaScript.
- View all journals
- My Account Login
- Explore content
- About the journal
- Publish with us
- Sign up for alerts
- Open access
- Published: 16 May 2023
Flood hazard potential reveals global floodplain settlement patterns
- Laura Devitt ORCID: orcid.org/0000-0001-8800-429X 1 ,
- Jeffrey Neal ORCID: orcid.org/0000-0001-5793-9594 1 , 2 , 3 ,
- Gemma Coxon ORCID: orcid.org/0000-0002-8837-460X 1 , 2 ,
- James Savage ORCID: orcid.org/0000-0003-4807-0916 3 &
- Thorsten Wagener 2 , 4 , 5
Nature Communications volume 14 , Article number: 2801 ( 2023 ) Cite this article
12k Accesses
21 Citations
33 Altmetric
Metrics details
- Natural hazards
Flooding is one of the most common natural hazards, causing disastrous impacts worldwide. Stress-testing the global human-Earth system to understand the sensitivity of floodplains and population exposure to a range of plausible conditions is one strategy to identify where future changes to flooding or exposure might be most critical. This study presents a global analysis of the sensitivity of inundated areas and population exposure to varying flood event magnitudes globally for 1.2 million river reaches. Here we show that topography and drainage areas correlate with flood sensitivities as well as with societal behaviour. We find clear settlement patterns in which floodplains most sensitive to frequent, low magnitude events, reveal evenly distributed exposure across hazard zones, suggesting that people have adapted to this risk. In contrast, floodplains most sensitive to extreme magnitude events have a tendency for populations to be most densely settled in these rarely flooded zones, being in significant danger from potentially increasing hazard magnitudes given climate change.
Similar content being viewed by others
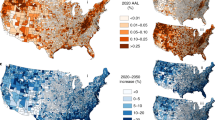
Inequitable patterns of US flood risk in the Anthropocene

Satellite imaging reveals increased proportion of population exposed to floods
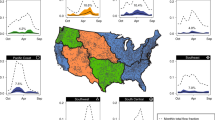
Floodplain inundation spectrum across the United States
Introduction.
Flooding causes devastating impacts worldwide, with global damages amounting to an estimated $651 billion (USD) and affecting 1.6 billion people between 2000 and 2019 alone 1 . Disastrous floods and their impacts are increasing in severity, duration, and frequency, mainly driven by population and economic growth in flood-prone areas 2 , 3 , and by climate change 4 , 5 , 6 , 7 . Losses from these events could increase by a factor of 20 by the end of 21st century 8 . Thus, understanding global fluvial flood risk and identifying population exposure is crucial for impact assessment and strategic management of future hazards 9 .
Climate change impact assessments regarding potential future global flood hazards commonly use a top-down, scenario propagation approach. They follow a modelling cascade starting with atmospheric projections produced by general circulation models (GCMs), which are downscaled and used to force hydrological models to produce simulations of future river flows e.g., ref. 10 . These flow simulations are then used to force global flood models to produce flood hazard maps, e.g., refs. 5 , 6 , 8 , which can in turn be used to derive impact estimates, such as population exposed and economic losses 11 .
The use of such model cascades can be problematic as there are numerous process parameterizations and other assumptions and uncertainties at each stage of the modelling chain, creating a ‘cascade of uncertainty’ 12 , with biases and errors inherited at each step. The precipitation outputs of GCMs are often significantly biased 13 , 14 , particularly regarding extremes 15 , which is then compounded with other uncertainties 16 . Additional modelling choices such as modelled river sizes have been shown to result in global flood exposure estimates varying by more than a factor of 2 17 . This uncertainty leads to drastically different estimates of flood hazards, with inundation extents found to vary by 80% between global flood models 18 . Each of these methodological choices fundamentally impact the portrayal of climate change impacts on future flood hazards 19 , 20 .
Instead of aiming to derive “scenario-led” assessments of future risk, one alternative strategy is to understand where socio-hydrological systems are sensitive to change by stress testing them against a plausible range of conditions 21 , 22 . While this approach contains its own assumptions and uncertainties (see discussion for review of uncertainties), it allows policymakers to identify the change in flood hazard that floodplains are most sensitive to. When used alongside model cascades, this approach can provide a more detailed climate change impact assessment and help to target future flood risk management interventions more effectively. This approach has been used to investigate the sensitivity of river flows 23 , 24 , 25 , 26 , but there are very few studies that assess the sensitivity of flood hazard and population exposure 27 . One recent study mapped the sensitivity of population exposure to changes in flood magnitude at the global scale 28 . However, their “downward counterfactual analysis” calculates a linear growth in exposure between event magnitudes, which is an unrealistic assumption of how societies have developed and settled on floodplains. The authors also exclude much of the global river network from the analysis as only river basins with upstream areas >5000 km 2 were included.
While a warming climate is expected to increase the frequency, intensity and duration of future flood hazards, human floodplain development activities are one of the main drivers for increases in damages and losses associated with flooding. Humans have a significant influence on the hydrological cycle 29 , whether it is intentional or not 30 , 31 , 32 . Human societies co-evolve in response to floods in many ways, formally or by necessity 33 and understanding the emergent phenomena and dynamics due to this co-evolution has been the focus of many country-specific and regional scale case studies 34 , 35 , 36 , 37 , 38 .
In this work, we provide a high-resolution stress test of the global river network to quantify and analyse the sensitivity of inundated areas (flood extent) and exposed population to plausible variability in flood magnitude. We analyse population exposure density in frequently and rarely flooded zones on floodplains and reveal previously unseen regional differences in settlement patterns with respect to flood hazards. We find that floodplains that are most sensitive to frequent, low-magnitude events have evenly distributed exposure across hazard zones, suggesting that people have adapted to this risk. Whereas for floodplains that are most sensitive to rare, extreme magnitude events, populations tend to have most densely settled in these rarely flooded zones, potentially being at significant risk from increasing hazard magnitudes induced by climate change.
We quantify whether river reaches are more sensitive to flooding from frequent, low magnitude or from rare, extreme magnitude flood events, and investigate the factors that control this sensitivity. Similarly, we also quantify the population that would be impacted by flooding across each floodplain, and thus estimate the sensitivity of population exposure to flood events of varying magnitudes. To do this, we propose a new sensitivity index which is calculated using fluvial flood hazard maps from the Fathom global flood model 39 (see ‘Methods’ for model description). This is the only global flood model based on a two-dimensional hydrodynamic model for floodplains that is coupled to a one-dimensional model of river channels. This level of model complexity is widely regarded as necessary for the accurate simulation of flood events as the river channel is the main conveyor of discharge and interacts with the floodplain as flood flows move both in and out of the channel 40 , 41 , 42 . These coupled processes are needed to simulate differences between low and high-magnitude floods, which is essential for a sensitivity analysis, such as the one performed in this study. The flood model hazard maps provide inundation extents from fluvial flooding from different exceedance probability for all rivers with an upstream area >50 km 2 at ~90 m resolution. We extracted the flooded extents for ~1.2 million river reaches (between 60 ° N and 56 ° S) for each exceedance probability, and then fitted a power law to the normalized growth curve (see ‘Methods’ for details). The exponent of this power law, b r , describes the shape of the growth in flooded extent with decreasing probability and is used to describe the sensitivity of flooding for each river reach (demonstrated in Fig. 1b ). Where b r is <1, flood extents grow most rapidly during frequent, low-magnitude events. When b r is >1, flood extents grow most rapidly during rare, extreme magnitude events. When b r is ~1, the flood extents grow linearly with return period.
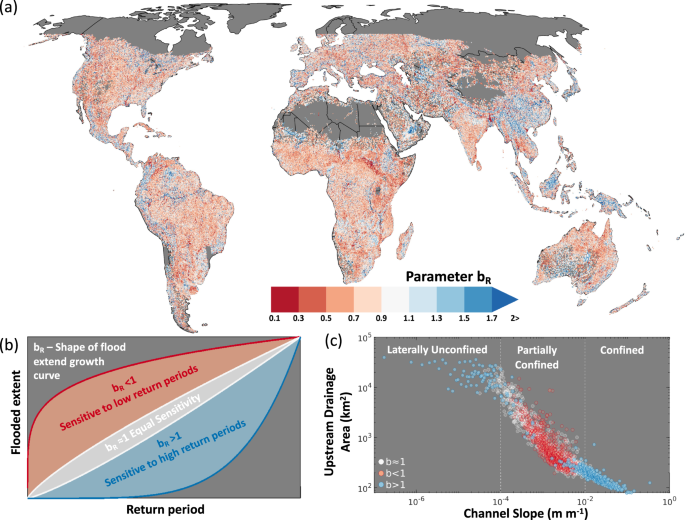
a Sensitivity of flood extents to increasing exceedance probability for the global river network (excluding river reaches beyond 56°N). b parameters <1 indicate a sensitivity of flood extents to short return periods (100-year flood event and less). b parameters >1 indicate a sensitivity of flood extents to long return periods (100-year flood event and greater). b Shape of the flood extent growth curve for a range of b parameters. c Relationship between river reach b parameter, channel slope and upstream drainage area. River reaches have been put into 2000 bins (each representing approx. 0.2% of the data). Thresholds are placed on the channel slope to define three dominant floodplain categories: confined, partially confined, and laterally unconfined.
Sensitivity of flood hazard
Figure 1a shows the sensitivity of flood extents to increasing event magnitudes for the global river network. There is a clear spatial pattern of reaches with different sensitivities to flooding. We investigated the relationship between reach sensitivity and numerous physical and climatic variables (see Supplement Fig. S1 ). We found that the dominant controls on the spatial pattern of floodplain sensitivity are local topography and upstream drainage area. Figure 1c shows how reach channel slope and upstream drainage area organize the sensitivity of 1.2 million global river reaches to flooding. Channel slope and upstream area relationships have previously been used to distinguish between bedrock and alluvial reaches along river longitudinal profiles, though mostly at the local scale 43 . Global scale studies are limited but have shown strong associations between channel slope and width with upstream drainage area within river networks 44 . We have used these findings to define thresholds for channel slope to group river reaches into three floodplain types: Confined, partially confined, and laterally unconfined (see Supplement Fig. S2 for conceptual diagrams). Confined floodplains are typically found alongside steep streams in mountainous bedrock regions and represent 8% of the reaches analysed here. In these confined floodplains, flood extents typically grow most rapidly during rare, extreme magnitude events, due to the steep slopes next to the river channel constraining how flood extents can grow laterally during frequent, low-magnitude events. Partially confined floodplains are the most common type, making up 89% of the reaches analysed. They are found on transitional streams, e.g., valley bottoms, and in these floodplains, flood extents typically grow most rapidly during frequent, low-magnitude events. This is due to the relatively wide and flat terrain next to the river channel that meets a break in slope which constrains the growth of inundated area. Laterally unconfined floodplains represent only 3% of the reaches analysed. They are very wide, flat, and unbound, which allows for exponential growth in inundation area, e.g., in deltas. The extent of flooding is typically discharge-limited, making them sensitive to changes in extreme discharge, and therefore flood extents grow most rapidly during rare, extreme magnitude events.
Global population exposure to flood hazards
To assess how physical flood hazard sensitivity interacts with population exposure, we first quantified the number of people living on floodplains (here defined as the 1000-year modelled flood extent) using WorldPop data 45 (see ‘Methods’ for description of dataset). We find that ~2 billion people live on floodplains globally, of which 1.4 billion live on the 100-year floodplain. The largest proportions of populations that have settled and developed on floodplains are found in North Africa, South America and South and East Asia (Fig. 2 ). The global distribution of reaches in each of the floodplain categories is rather even, with partially confined being the dominant type of floodplain in all regions (see pie charts in Fig. 2 ). However, the proportion of the population settled on these reaches differs regionally. This is critical as the impacts of changes in flood hazard will not affect the global population uniformly, instead, regions across the globe would have different experiences in how population exposure to floods would change. Populations on partially confined floodplains are most likely to be susceptible to increasing intensity of frequent flood events. They might therefore be impacted by deeper flood waters, such as those occurring in cases of overtopping of defences, leading to significant potential for damages and economic losses. In contrast, populations on laterally unconfined and confined reaches are more likely to be impacted by changes in the probability of extreme rare floods which would reach parts of the floodplain which were likely not inundated in living memory. Thus, providing the potential for catastrophic and unanticipated impacts.
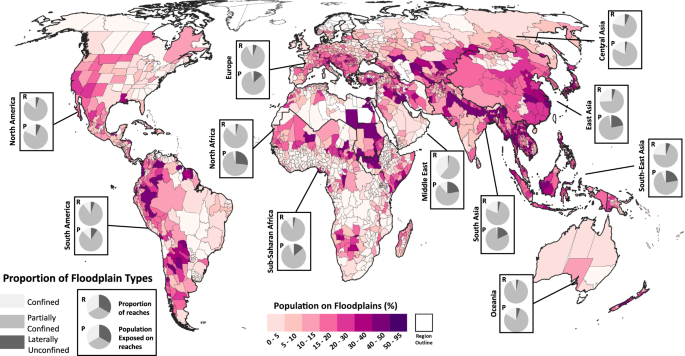
Percentage of the population in each sub-national administrative region that live on floodplains, which is defined as the 1000-year flood extent. The proportion of the reaches that are in each of the floodplain categories for each region is shown by the top pie charts in each box. The proportion of the population settled on the different types of floodplains is shown by the bottom pie chart in each box.
The region with the largest number of people exposed to flooding is Asia, with 1.5 billion people living on floodplains (35% of the continent’s total population). This number accounts for 75% of the world’s population on floodplains, of which China and India have the largest share (490 million and 456 million living on floodplains, respectively), forming almost half of all global population exposed. Laterally unconfined floodplains such as deltas only account for 5% of the reaches across Asia. However, 20% of the population exposure is encountered on this floodplain type. These floodplains are particularly sensitive to changes in extreme river flows, and therefore a large proportion of the population could be at risk from disastrous flood events with return periods of 100-years or greater. In Bangladesh, which also has a significant number of people living on floodplains (105 million), 57% of the population exposure is on laterally unconfined floodplains, meaning many people are at risk from flooding from extreme magnitude flood events. A summary of the exposure in other regions is given in the Supplemental Information (see regional exposure on floodplains in supplement).
Sensitivity of population exposure to flood magnitudes
To analyse the sensitivity of populations to flooding from varying event magnitudes, we also calculated a sensitivity index for exposure, b pop , in a similar manner to b r (see ‘Methods’ section for full description). There are three categories of exposure sensitivity (see Fig. S3A ): (1) the highest fraction of the exposed population lives in the flood zones that flood rarely, i.e. they flood only during extreme magnitude events ( b pop > 1; diagrams 1 and 2 in Fig. S 3A ), (2) population is approximately equally distributed across each of the flood zones ( b pop about 1; diagrams 3 and 4 in Fig. S 3A ), and (3) the highest population fraction lives in the flood zones that flood frequently, i.e. they flood already during low-magnitude events ( b pop < 1; diagrams 5 and 6 in Fig. S 3A ). Figure 3 shows the relationship between the mean flood hazard sensitivity and population exposure sensitivity for all floodplain types at the country level. This approach allows us to visualize the interaction between the sensitivity of flood extents to varying event magnitudes, with the distribution of populations across hazard zones for different floodplain types and countries. Points along the 1:1 line represent regions where population exposure grows at a similar rate as the flood hazard for increasing event magnitude. Points on this line have the same population density in both frequently and rarely flooded zones of the floodplain. Points below this line indicate floodplains where the highest density of people is found in the frequently flooded zones of the floodplain. Locations represented by points above the 1:1 line indicate floodplains where the highest density of exposed population are found within the rarely flooded areas.
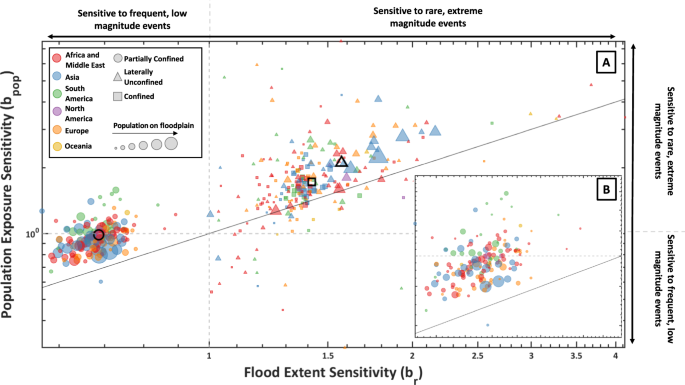
a The country level mean flood hazard and population exposure sensitivity parameters ( b r and b pop ) are calculated for each floodplain type. Each country has three marker shapes—one each for the mean sensitivity parameters on confined, partially confined, and laterally unconfined floodplains. The grey dashed lines indicate where the flood hazard (x axis) and population exposure (y axis) growth curves transition from growing most rapidly during frequent, low-magnitude events to during rare, extreme magnitude events. Marker closest to the 1:1 line have growth curves with similar shapes for flood extent and population, which indicates that population is distributed evenly across floodplains. Locations above the 1:1 line have greater population densities on rarely inundated areas of the natural floodplain, while locations below the line have greater population densities in frequently flooded areas. Where b pop < 1 more people, in absolute terms, live on the frequently flooded floodplain, while b pop > 1 indicates more people live in rarely flooded areas (see examples (4) and (3) in Fig. S3B ). Point shapes represent the floodplain category for each country, the sizes have been scaled based on the number of people living on the different floodplain types in each country, and the colour refers to the region/continent that the country is in. The bold open shapes represent the centroid of all the data points for each of the floodplain types. b Close view of the partially confined floodplain results. Note: Continent specific plots are included in Fig. S5 for ease of visualisation.
For partially confined floodplains, all countries, except for one outlier (East Timor), are located above the 1:1 line, indicating that population density is greater on rarely flooded zones of the floodplains. However, there are many countries that have a b pop below 1, mostly in Europe and Asia. This means that in absolute terms more people live on frequently flooded zones of floodplains because they cover a greater area. This indicates that people have either found ways to cope with flooding from frequent, low-magnitude events, or have taken on risk through choice or necessity 46 . In Fig. S4 (supplement), countries have been sized based on their mean standard of protection extracted from the FLOPROS global flood defense database 47 , which reveals that some of these countries that have larger populations on frequently flooded floodplains and also have high standards of protection. In Europe, 22 countries (58%) have a mean standard of protection of at least a 50-year return period, while 10 countries (26%) have very high standards of protection of at least a 100-year return period (Austria, Belgium, Germany, United Kingdom, Croatia, Hungary, Ireland, Netherlands, Poland, Portugal, Romania, and Slovakia). 39 million people are settled on partially confined floodplains in these countries, accounting for 48% of the total exposure on this floodplain type in Europe. It is likely that in these countries significant investments have been made to mitigate the impacts of flooding from frequent flooding. In Asia, the FLOPROS database suggests that the standard of protection in many countries is low, even though the number of people exposed in frequently flooded zones of partially confined floodplains is high (1.1 billion people living on this floodplain type). This could be due to population pressure in rapidly expanding urban areas 48 , 49 or due to a reliance on frequently flooded floodplains for agricultural practices 50 . However, the FLOPROS database has very little data in these regions and might underestimate the degree of adaptation present.
Many countries in South and Central America exhibit different settlement patterns, where the highest density of population exposure is found in the rarely flooded zones of the floodplain. These countries include Brazil, Haiti, Paraguay, Costa Rica, Suriname, Puerto Rico, Argentina, and El Salvador. In these countries, the mean b pop is >1, which means that while the flood extents are growing most rapidly during frequent, low-magnitude events, the population exposed is found most densely in rarely flooded zones to the extent that the absolute numbers exposed are greater there (see example (1) in Fig. S3B ). This indicates that people are more likely to mitigate risk posed by frequent flooding by living away from rivers in these parts of the world (see Figs. S6 and S7 for analysis of floodplain population density).
For the confined and laterally unconfined floodplains, most countries are also located above the 1:1 line. There are examples where the population is most densely populated in the frequently flooded zones, for example, the Dominican Republic, Zimbabwe, Cuba, Panama, Togo, Syria, and Greece. However, these places have very small population totals in these floodplain categories (<10,000 exposed) and they only make up a very small proportion of the total exposure in the country (all <1%). On steep confined reaches, many countries are close to the 1:1 line, indicating an even distribution of population across flood hazard zones. For laterally unconfined floodplains (deltas), Asian countries have the largest total populations exposed (~312 million). These people are found above the 1:1 line meaning the highest density of exposure is found in the rarely flooded zones (see example (2) in Fig. S3B for example growth curves). These results imply that people are preferentially settling and developing on parts of the floodplain that they deem to be at low risk. China, India, Bangladesh and Vietnam have the largest populations settled on laterally unconfined floodplains, with over 160 million people settled in the rarely flooded zones of the floodplain (e.g., >100-year return period). There are few countries whose points plot below the 1:1 line but which have b pop values >1 (i.e., where the population density is slightly higher in the frequent flood zones—example (3) in Fig. S3B ). This indicates that, in terms of absolute population numbers, the development on frequently flooded areas of laterally unconfined floodplains is less prevalent than on partially confined floodplains.
In this study, we have quantified the sensitivity of flood hazard and population exposure at the global scale. Our results contribute to the understanding of global flood risk, and the relationship between rivers, their floodplains, and human settlements. We find clear regional differences in settlement patterns that are related to whether floodplains are most sensitive to flooding from frequent and low magnitude, or to rare and extreme magnitude events.
Flood extents on partially confined floodplains grow most rapidly during frequent, low-magnitude events. On this floodplain type, nearly all countries are found to have the highest density of exposure in the rarely flooded zones of the floodplain. However, we find regional differences in whether more people are living in the frequently or rarely flooded zones. In Europe, countries exhibit a mostly even distribution of population density throughout all flood zones, with population totals being greatest on areas of the floodplain that would naturally flood frequently given that they make up the largest areas. For Europe and North America, this is likely due to significant investments in structural defences to protect against flooding from frequent events (see analysis of global protection standards in Fig. S4 ). However, structural protection tends to encourage development in flood-prone zones, a socio-hydrological process known as the “levee effect” 51 , 52 . This effect can lead to floodplain settlements becoming vulnerable to low-probability but potentially high-consequence flood events 51 , 53 , 54 , 55 which is problematic under climate change induced non-stationarity of flood extremes. This effect was for example seen during the catastrophic 2005 flooding of New Orleans brought by Hurricane Katrina 56 and the devasting impacts of the European floods of 2021 57 .
Asian countries have a similar settlement pattern on partially confined floodplains. Given the particularly high density of population on this floodplain type in this region—14% of total land area experiences flooding but 35% of the total population are settled here (see Fig. S7 )—it is likely that pressures on land have resulted in people settling in areas of the floodplain at risk from frequent flooding. A study that analysed urban growth between 1985 and 2015 finds that growth of settlements in high hazard areas has been most rapid in Asia. In fact, ‘no risk’ settlements expanded by about 100%, while ‘very high risk’ settlements expanded by over 160% 49 . As the economies of these countries grow rapidly and safe locations in urban areas become increasingly scare, new developments are built on hazardous and often cheap land to match the pace of population growth 58 , 59 . Another study that analysed the prioritizing of floodplains for development and farming finds the percent of cropland area in floodplains in East, Southeast and South Asia to be 51%, 67% and 59%, respectively 48 . This large fraction highlights the reliance on frequently flooded land for agriculture in the region and the necessity for human presence in floodplains. Although the FLOPROS global flood defence database suggests a far lower standard of protection (see Fig. S4 ) in this region compared to Europe and North America, the database is sparsely populated, and we have low confidence in the information provided. Given the very substantial exposure to frequent flooding in Asia addressing this data gap should be a priority.
South America is the only continent where more people live in rarely flooded areas of partially confined floodplains relative to the frequently flooded areas. These countries also have the highest population density in these low hazard zones. Standards of protection are generally low, however, the relatively low population density on floodplains in general (see Figs. S6 and S7 ) has allowed for preferential settlement in less hazardous locations. In Fig. S7 we find around 24% of total land area in South America has potential for flooding, but only 13% of the total population lives there. Further, there is empirical evidence that during flood-rich periods impacts of flood events are reduced when an event of similar magnitude had occurred not long before. An example of this are the floods that occurred during the 1982–83 and 1991–92 ENSO events on the Paraná River. Flood damages and economic losses were reduced by 80% in the later event 60 . This dynamic has been termed the “adaptation effect” 38 , which refers to the increased coping capabilities of a population due to their experiences of earlier flooding, unless subsequent flooding is more extreme 61 . The combination of relatively low population pressure on floodplains and regular experience of flood-rich periods might have facilitated relatively hazard averse settlement patterns. Our results contrast with the findings of a recent analysis of the sensitivity of flood exposure 28 , where populations in South America are found to be most sensitive to flooding from frequent flood events. This difference is likely due to the choice of global flood model and methodological choices. The previous study used the JRC flood model 62 , which has a coarser spatial resolution (~1 km) and only includes rivers with an upstream area >5000 km 2 . The reaches that are included are known to show a significant overprediction bias due to the models’ resolution and a lack sensitivity to event magnitude (about 5 times less than Fathom Global Flood model which is used here) 18 , 63 . To mitigate for overprediction of frequent flooding, flood exposure was set to zero below the standard of protection return period extracted from the FLOPROS database, essentially identifying floodplain settlement outside of Europe and North America as relatively sensitive to frequent flooding.
Laterally unconfined floodplains experience flood extents that grow most rapidly during rare, extreme magnitude events. We find that there is a strong tendency for populations to have preferentially settled on these rarely flooded zones worldwide. Although only making up 5% of the populated river reaches in our analysis, laterally unconfined floodplains are home to ~412 million people, thus accounting for 21% of the total population exposure globally. The highest population density on laterally unconfined floodplains is found in Asia and North Africa. Figure S8 (supplement) shows that these regions also have the highest density of exposure per river reach on these floodplains, with 4519, 2536, 1973 and 902 people exposed per kilometre of reach length in South-, East, South-East Asia, and North Africa, respectively. This is for example compared with only 183 and 247 people exposed per kilometre of reach length in North America and Europe. With potential increases in the frequency of extreme river flows in the projected future for these regions 64 , an exponential growth in population impacted by flooding could be experienced in locations that typically would not have flooded within living memory. For example, in China, 82% of the reaches with laterally unconfined floodplains have the highest density of exposure found in the rare, extreme magnitude flood zones (69 million people living in the 100-year flood zone or greater). These regions have experienced rapid population growth, urbanisation, and industrialisation in the last two decades, including the growth of some of the world’s largest urban agglomerates on large river floodplains and deltas, such as Shanghai, Cairo, Dhaka, and Bangkok. Urban population is expected to continue to grow in the future with 90% of the projected increase taking place in Asia and Africa 65 . This continued growth will put additional strain on the existing floodplain settlements, placing even more people at risk from flooding in the future.
These results provide new insights into the sensitivity of floodplains and their populations to the potential of changing flood hazard. They are helpful to identify the greatest potential for significantly larger flood damage if flood magnitudes should increase anywhere across the world. These results therefore provide a first-order global guide for policy making on how a change in hazard might interact with exposure. Adaptation will be a critical factor in determining the severity of impacts from flood hazards in the coming decades 27 . The approach demonstrated here can provide crucial information for global agencies on where it would be most beneficial to implement flood management strategies—guidance that is urgently needed for a wide range of hazards 66 . Recent devasting floods across the globe have sharply brought into focus the urgent need to make societies more resilient to flooding now and even more so in the future 1 . Effective flood risk adaptation efforts must be based on a robust understanding of the physical dangers posed by the hazard and the increasing exposure to these events. In most regions, partially confined floodplains reveal a distribution of population across flood hazards zones that means more people live on floodplain areas that would naturally flood frequently. These populations are most likely to be susceptible to increasing intensity of frequent flood events and deeper flood waters, which may lead to significant damages danger and risk to human life if existing structural protection is insufficient. This was shown to be a major problem during the European floods of 2021 where river flows exceeded the 400-year magnitude in some places and resulted in over 200 fatalities and $46 billion in damages 57 . Such floodplains would benefit from restricting development on areas of the floodplain and from investments to raise the standard of protection of existing defences. Other measures, such as enacting zoning regulations and enhancing building codes may contribute to reducing flood damages 67 .
Populations on laterally unconfined floodplains are typically most densely settled in the rarely flooded zones of the floodplain (i.e., greater than the 100-year flood event), with many people living in areas that may not have experienced large-scale flood events in living memory. 76% of all populations settled on laterally unconfined floodplains are found in Asia (~312 million people), which is particularly prone to flood hazards. Recent disastrous flooding, such as the 2017 1-in-200-year flood event in South Asia, impacted over 40 million people and killed over 1000 68 , highlighting the need for investment in adaptation strategies to extreme magnitude events in this region. One of the key challenges is how to address the role of individual perceptions of risk and how these perceptions influence risk-reducing behaviour 67 . Bangladesh and Vietnam are typical examples of societies ‘living with floods’ 50 , 69 with people adapting to regular flooding by adjusting economic activities to benefit from regular inundation, e.g., through farming and fisheries 38 , 50 , 70 .
Our study has been motivated by a need for complementary methods to assess global scale flood risk that are independent of at least some of the uncertainties associated with the traditional, top-down model cascade approach 27 . We therefore chose an exploratory modelling framework in which we stress test the socio-hydrologic system of global floodplains. However, our methodology and subsequent analysis are of course not free from their own uncertainties and assumptions. We have used simulated fluvial flood hazard maps from a state-of-the-art global flood model to calculate our sensitivity index, which contains its own uncertainties, originating from the quality of the elevation dataset used, knowledge of river morphology and choice of hydrodynamic model 71 . How discharge grows with return period will influence the shape of the flooded area and population sensitivity curve. Thus, one notable source of uncertainty is the use of regional flood frequency analyses 72 to estimate the growth in discharge towards more extreme conditions from pooled gauged discharge data in the Fathom flood model. These methods are limited by their need for gauged discharge data, themselves most uncertain during measurements of out-of-bank flows 73 , 74 , and are limited by the length of observation periods, meaning statistical extrapolation methods are needed to estimate the probability of as yet unobserved extremes 75 , 76 . Discharge observations are particularly sparse in arid areas, and analysis of extreme flows used in our model, and other flood models, have shown that uncertainty in extreme river flows is greatest here 16 . Furthermore, validation of the global flood model used here demonstrated that the accuracy of estimated flood hazard is lowest in arid areas relative to other climate zones 77 . The alternative to gauge-based methods is using discharge simulated by global hydrological models, however, these flows are typically generated with a lack of data to calibrate and parameterise models. They are also driven with meteorological data that poorly represent extremes 78 , 79 , 80 , leading to predictions that are highly variable between models 16 .
One key assumption is that the shape of the discharge growth curve will remain the same in the future 80 . Some studies have found differing trends in the median flood and more extreme return periods (i.e., 100-year flood) due to changing flood-generating processes in some regions 27 , 81 , 82 , 83 . However, an extensive review of flood estimation guidance in different countries 84 reveals a strong focus on using multiplicative scaling (uplifts) applied directly to peak flow rates uniformly across return period 85 . This assumption is often made due to a lack of data and poorly understood impacts of future climate change on flood-generating processes. Hydrologic non-stationarity has been discussed 86 , 87 , 88 , but incorporating the multiple sources of changes to flood hazard (e.g., land-use changes and climate change impacts on flood-generating processes) into specific flood estimation methodologies and carrying this into meaningful guidance to be used by decision-makers remains a major challenge 89 , 90 , 91 , 92 , 93 . We assume that the shape of the growth curve between flood extend, or population exposed, and return period is representative for current as well as for potential future conditions (in line with current hydrological practice as discussed above). Under this assumption, our sensitivity index remains a representative indicator which allows us to understand whether a floodplain would be impacted more by an increasing probability of extreme events or a change in intensity of frequent flood events.
Another issue faced by global flood hazard models is the challenge of accounting for flood defences, especially in regions with high protection standards. Although we consider the impact of flood defences in this study, our use of undefended fluvial flood maps means we define sensitivity before these are considered. This will have overestimated exposure in countries with extensive flood protection. However, existing flood defence databases, e.g., FLOPROS 47 are, at best, informative at the national scale, as they do not make distinctions for different floodplain types and can only provide data to be superimposed onto global flood hazard maps 94 . Improving these databases to include data on defences in places where we currently have little knowledge, e.g., Asia, is crucial as this is likely to be the greatest uncertainty in global flood exposure estimates. Also, expanding these datasets to include data on spatial planning and flood zoning policies implemented as adaptation measures would be incredibly beneficial for continuing to develop our understanding of floodplain settlement patterns.
The results presented here are based on the sensitivity of river reaches and population exposed to fluvial flood hazards. We have not included other types of flood hazards, such as floods from storm surges or coastal flooding. This will be an important factor in many coastal river reaches, deltas and in regions that experience compound flood events, i.e., where fluvial and coastal flooding occurs simultaneously 95 , 96 . A recent study of flood risk and its interaction with poverty 97 included fluvial, coastal and pluvial flood hazard types in its estimate of global flood exposure. The authors found 1.8 billion people exposed to 1-in-100-year floods, compared with 1.4 billion exposed to fluvial flooding. Our results have only focused on population exposure to flooding. Future work could use the proposed methodology to calculate the sensitivity of other assets, for example infrastructure, traffic systems, or public and private property 11 , 63 , 98 , 99 . While the results shown here represent a large proportion of the global river network, they are also not complete. We have only included rivers with an upstream drainage area >50 km 2 , as this is the threshold for inclusion of rivers in the global flood hazard model used. We quantified the sensitivity of ~1.4 million river reaches and removed 12% of reaches due to a poor power law fit. We deem these reaches to have relationships between flood extent and event magnitudes that are more complex, and therefore additional work is needed to look at their behaviour and sensitivity. However, the reaches included in our analysis cover 89% of the global population living on floodplains.
Global flood hazard data
To investigate the sensitivity of global inundation extents to changes in flood magnitude, flood hazard data from the Fathom global flood model are used. Flood hazard for ten return periods (5-, 10-, 20-, 50-, 75-, 100-, 200-, 250-, 500- and 1000-year) with a horizontal resolution of 3 arc s ~90 m was simulated using the global flood model methodology of Sampson et al. 39 . The global flood modelling framework begins with extreme river flows being estimated using a regional flood frequency analysis (RFFA) 72 applied to GRDC gauging station data. These fluvial model boundary conditions are routed through 1D subgrid-scale river channels based on MERIT Hydro 100 , 101 and a river bathymetry estimation routine 42 . To simulate floodplain inundation, the 1D model is coupled to a 2D hydrodynamic model for out-of-bank flows. All hydrodynamic calculations are based on the LISFLOOD-FP model, which solves a local inertial formulation of the shallow water equations 101 , 102 , 103 . Elevation data used is from the MERIT DEM (~90 m resolution) 104 that corrects for multiple errors, including absolute bias, stripe noise, speckle noise, and tree and building height biases. Inundation simulations are made for all rivers with a drainage area >50 km 2 . We have used the undefended flood maps, which do not include flood protection structures. No new model components are introduced in this study beyond those that have previously been described and validated 39 , 105 , 106 , 107 .
Global population data
The data used here is the 2020 WorldPop constrained population counts dataset 108 . WorldPop uses a complex model to disaggregate population over an area 29 . It uses a random forest model and a number of ancillary datasets to dynamically weight the distribution of census data over a ~90 m gridded area 108 . We processed the country-level GeoTiff data provided by WorldPop to match the 10° × 10° tile format of the global flood hazard maps.
Quantifying sensitivity of flood extents to flow magnitude
To quantify the sensitivity of flooded extent to increasing flow magnitudes a generalised methodology has been developed. The global flood model produces hazard maps of flood depth at ~90 m resolution for ten return periods. Each grid was firstly converted to binary flood extents using flood depth threshold of >0 m. Inflow points are defined along the global river network as the location for the boundary conditions of the hydraulic modelling component. These were extracted and assigned as upstream and downstream points, and this was used to define each reach along the river network. A flooded area was calculated between each upstream and downstream segment of the river network for each of the return periods (see Fig. S8 for a diagram). The reach length is used as an effective search radius along a diagonal transect between the upstream and downstream points. For each inflow point, the flooded area was derived for each return period and normalised. A power law with the form: F = RP b r , where F is the normalised flooded area, RP is the log normalised return period of the flood event and b r is the exponent, was fitted to the data. We chose to fit the curve between the return period and flooded extent to produce comparable sensitivity parameters at all sites by removing the influence of river flow magnitude.
The ‘ b r ’ parameter of the power law is used to describe the shape of the flood extent growth curve and is used as the measure of floodplain sensitivity to increasing return period. When b r is <1, the growth curve has a concave shape and grows most rapidly during the low return periods. When b r is >1, the growth curve has a convex shape and grows most rapidly during the high return periods. When b r is 1, the growth curve is linear. An R 2 value was calculated to measure the goodness-of-fit of the power law. Sites with an R 2 < 0.9 were removed from the analysis. Of 1,380,430 inflow sites across the domain, 161,947 (12% of sites) were excluded.
Quantifying the sensitivity of population exposure to flow magnitude
Human exposure here is defined as the intersection of the flood hazard data with a spatially distributed population dataset. We multiplied the binary flood extent layers with the WorldPop data to obtain the number of people exposed for each return period. Using the same method as for the flood extents, we extracted the total number of people exposed on each river reach. We then calculated the sensitivity of the population exposure by first normalising the totals and fitting a power law of the form: E = RP b pop , where E is the normalised exposure, RP is the log normalised return period of the flood event and b pop is the exponent.
The ‘ b pop ’ parameter of the power law is used to describe how population exposure grows with increasing return period of the flood hazard. When b pop is <1, the growth curve has a concave shape and grows most rapidly during the low return periods. When b pop is >1, the growth curve has a convex shape and grows most rapidly during the high return periods. When b r is 1, the growth curve is linear.
Data availability
Fathom global flood model data are available for academic purposes and were provided by Fathom. The WorldPop unconstrained high-resolution population counts are available to download online ( https://hub.worldpop.org/geodata/listing?id=29 ). The FLOPROS database is available to download from the supplementary material of the dataset description paper ( https://nhess.copernicus.org/articles/16/1049/2016/ ).
UNDRR. Annual Report (2021).
Jongman, B., Ward, P. J. & Aerts, J. C. J. H. Global exposure to river and coastal flooding: long term trends and changes. Glob. Environ. Change 22 , 823–835 (2012).
Article Google Scholar
Visser, H., Petersen, A. C. & Ligtvoet, W. On the relation between weather-related disaster impacts, vulnerability and climate change. Clim. Change 125 , 461–477 (2014).
Article ADS Google Scholar
Gudmundsson, L. et al. Globally observed trends in mean and extreme river flow attributed to climate change. Science 371 , 1159–1162 (2021).
Article ADS CAS PubMed Google Scholar
Alfieri, L. et al. Global projections of river flood risk in a warmer world. Earth ’ s Futur. 5 , 171–182 (2017).
Google Scholar
Hirabayashi, Y. et al. Global flood risk under climate change. Nat. Clim. Chang. 3 , 816–821 (2013).
Cloke, H. L., Wetterhall, F., He, Y., Freer, J. E. & Pappenberger, F. Modelling climate impact on floods with ensemble climate projections. Q. J. R. Meteorol. Soc. 139 , 282–297 (2013).
Winsemius, H. C. et al. Global drivers of future river flood risk. Nat. Clim. Chang. 6 , 381–385 (2016).
Rentschler, J. & Salhab, M. People in Harm’s Way: Flood Exposure and Poverty in 189 Countries (The World Bank, 2020).
Arnell, N. W. & Gosling, S. N. The impacts of climate change on river flood risk at the global scale. Clim. Change 134 , 387–401 (2016).
Dottori, F. et al. Increased human and economic losses from river flooding with anthropogenic warming. Nat. Clim. Chang. 8 , 781–786 (2018).
Wilby, R. L. & Dessai, S. Robust adaptation to climate change. Weather 65 , 180–185 (2010).
Felder, G. et al. From global circulation to local flood loss: coupling models across the scales. Sci. Total Environ. 635 , 1225–1239 (2018).
Smith, A., Bates, P., Freer, J. & Wetterhall, F. Investigating the application of climate models in flood projection across the UK. Hydrol. Process 28 , 2810–2823 (2014).
Schewe, J. et al. State-of-the-art global models underestimate impacts from climate extremes. Nat. Commun. 10 , 1005 (2019).
Article ADS PubMed PubMed Central Google Scholar
Devitt, L., Neal, J., Wagener, T. & Coxon, G. Uncertainty in the extreme flood magnitude estimates of large-scale flood hazard models. Environ. Res. Lett. 16 , 064013 (2021).
Bernhofen, M. V., Trigg, M. A., Sleigh, P. A., Sampson, C. C. & Smith, A. M. Global flood exposure from different sized rivers. Nat. Hazards Earth Syst. Sci. 21 , 2829–2847 (2021).
Trigg, M. A. et al. The credibility challenge for global fluvial flood risk analysis. Environ. Res. Lett. 11 , 094014 (2016).
Clark, M. P. et al. Characterizing uncertainty of the hydrologic impacts of climate change. Curr. Clim. Change Rep. 2 , 55–64 (2016).
Melsen, L. A. et al. Subjective modeling decisions can significantly impact the simulation of flood and drought events. J. Hydrol. (Amst.) 568 , 1093–1104 (2019).
Wagener, T., Reinecke, R. & Pianosi, F. On the evaluation of climate change impact models. WIREs Clim. Change 13 , e772 (2022).
Hadjimichael, A. et al. Defining robustness, vulnerabilities, and consequential scenarios for diverse stakeholder interests in institutionally complex river basins. Earth ’ s Futur. 8 , e2020EF001503 (2020).
Kim, D., Chun, J. A. & Aikins, C. M. An hourly-scale scenario-neutral flood risk assessment in a mesoscale catchment under climate change. Hydrol. Process 32 , 3416–3430 (2018).
Guo, D., Westra, S. & Maier, H. R. Use of a scenario-neutral approach to identify the key hydro-meteorological attributes that impact runoff from a natural catchment. J. Hydrol. 554 , 317–330 (2017).
Broderick, C. et al. Using a scenario‐neutral framework to avoid potential maladaptation to future flood risk. Water Resour. Res. 55 , 1079–1104 (2019).
Keller, L., Rössler, O., Martius, O. & Weingartner, R. Comparison of scenario‐neutral approaches for estimation of climate change impacts on flood characteristics. Hydrol. Process 33 , 535–550 (2019).
Merz, B. et al. Causes, impacts and patterns of disastrous river floods. Nat. Rev. Earth Environ. 2 , 592–609 (2021).
Zischg, A. P. & Bermúdez, M. Mapping the sensitivity of population exposure to changes in flood magnitude: prospective application from local to global scale. Front. Earth Sci. 8 , 390 (2020).
Vörösmarty, C. J., Pahl-Wostl, C., Bunn, S. E. &Lawford, R. Global water the anthropocene and the transformation of a science. Curr Opin Environ Sustainability 5 , 539–550 (2013).
Blöschl, G., Nester, T., Komma, J., Parajka, J. & Perdigão, R. A. P. The June 2013 flood in the Upper Danube Basin, and comparisons with the 2002, 1954 and 1899 floods. Hydrol. Earth Syst, Sci . 17 , 5197–5212 (2013)
Savenije, H. H. G., Hoekstra, A. Y. & van der Zaag, P. Evolving water science in the Anthropocene. Hydrol. Earth Syst. Sci. 18 , 319–332 (2014).
Adger, W. N., Quinn, T., Lorenzoni, I., Murphy, C. & Sweeney, J. Changing social contracts in climate-change adaptation. Nat. Clim. Chang. 3 , 330–333 (2013).
McMillan, H. et al. Panta Rhei 2013–2015: global perspectives on hydrology, society and change. Hydrol. Sci. J. 1–18, https://doi.org/10.1080/02626667.2016.1159308 (2016).
Liu, Y., Tian, F., Hu, H. & Sivapalan, M. Socio-hydrologic perspectives of the co-evolution of humans and water in the Tarim River basin, Western China: the Taiji–Tire model. Hydrol. Earth Syst. Sci. 18 , 1289–1303 (2014).
Ertsen, M. W., Murphy, J. T., Purdue, L. E. & Zhu, T. A journey of a thousand miles begins with one small step – human agency, hydrological processes and time in socio-hydrology. Hydrol. Earth Syst. Sci. 18 , 1369–1382 (2014).
Di Baldassarre, G. et al. Socio-hydrology: conceptualising human-flood interactions. Hydrol. Earth Syst. Sci. 17 , 3295–3303 (2013).
Di Baldassarre, G. et al. Debates-Perspectives on socio-hydrology: capturing feedbacks between physical and social processes. Water Resour. Res. 51 , 4770–4781 (2015).
Sampson, C. C. et al. A high-resolution global flood hazard model. Water Resour. Res. 51 , 7358–7381 (2015).
Fewtrell, T. J., Neal, J. C., Bates, P. D. & Harrison, P. J. Geometric and structural river channel complexity and the prediction of urban inundation. Hydrol. Process 25 , 3173–3186 (2011).
Knight, D. & Shiono, K. in Floodplain Processes (eds. Anderson, M., Walling, D. & Bates, P.) 139–181 (Wiley, 1996).
Neal, J. et al. Estimating river channel bathymetry in large scale flood inundation models. Water Resour. Res. 57 , e2020WR028301 (2021).
Brocard, G. Y. & van der Beek, P. A. in Tectonics, Climate, and Landscape Evolution (Geological Society of America, 2006).
Frasson, R. P. et al. Global relationships between river width, slope, catchment area, meander wavelength, sinuosity, and discharge. Geophys. Res. Lett. 46 , 3252–3262 (2019).
Tatem, A. J. WorldPop, open data for spatial demography. Sci. Data 4 , 170004 (2017).
Article PubMed PubMed Central Google Scholar
Loayza, N. V., Olaberría, E., Rigolini, J. & Christiaensen, L. Natural disasters and growth: going beyond the averages. World Dev. 40 , 1317–1336 (2012).
Scussolini, P. et al. FLOPROS: an evolving global database of flood protection standards. Nat. Hazards Earth Syst. Sci. 16 , 1049–1061 (2016).
Dryden, R., Anand, M., Lehner, B. & Fluet-Chouinard, E. Do we prioritize floodplains for development and farming? Mapping global dependence and exposure to inundation. Glob. Environ. Change 71 , 102370 (2021).
Rentschler, J. et al. Rapid Urban Growth in Flood Zones: Global Evidence since 1985 (2022).
Nguyen, K. V. & James, H. Measuring household resilience to floods: a case study in the Vietnamese Mekong River Delta. Ecol. Soc. 18 , art13 (2013).
Collenteur, R. A., de Moel, H., Jongman, B. & Di Baldassarre, G. The failed-levee effect: do societies learn from flood disasters? Nat. Hazards 76 , 373–388 (2015).
White, G. Human adjustment to floods. A geographical approach to the flood problem in the United States. in Research Paper No. 29 (The University of Chicago, 1945).
Mård, J., Di Baldassarre, G. & Mazzoleni, M. Nighttime light data reveal how flood protection shapes human proximity to rivers. Sci. Adv. 4 , eaar5779 (2018).
Di Baldassarre, G., Castellarin, A. & Brath, A. Analysis of the effects of levee heightening on flood propagation: example of the River Po, Italy. Hydrol. Sci. J. 54 , 1007–1017 (2009).
Burton, C. & Cutter, S. L. Levee failures and social vulnerability in the Sacramento-San Joaquin Delta Area, California. Nat. Hazards Rev. 9 , 136–149 (2008).
Kates, R. W., Colten, C. E., Laska, S. & Leatherman, S. P. Reconstruction of New Orleans after Hurricane Katrina: a research perspective. Proc. Natl Acad. Sci. USA 103 , 14653–14660 (2006).
Article ADS CAS PubMed PubMed Central Google Scholar
Kreienkamp, F. et al. Bert Van Schaeybroeck 13, Robert Vautard 5, Demi Vonk 8, Niko Wanders 12 1-Deutscher Wetterdienst (DWD). R. Neth. Meteorol. Inst. 13 , 18 (2021).
Hallegatte, S. How Economic Growth and Rational Decisions Can Make Disaster Losses Grow Faster Than Wealth (2011).
Hallegatte, S. An Exploration of the Link between Development, Economic Growth, and Natural Risk (2012).
Latrubesse, E. M. & Brea, D. Floods in Argentina . 333–349 https://doi.org/10.1016/S0928-2025(08)10016-5 (2009).
Kreibich, H. et al. The challenge of unprecedented floods and droughts in risk management. Nature 608 , 80–86 (2022).
Dottori, F. et al. Development and evaluation of a framework for global flood hazard mapping. Adv. Water Resour. 94 , 87–102 (2016).
Aerts, J. P. M., Uhlemann-Elmer, S., Eilander, D. & Ward, P. J. Comparison of estimates of global flood models for flood hazard and exposed gross domestic product: a China case study. Nat. Hazards Earth Syst. Sci. 20 , 3245–3260 (2020).
Hirabayashi, Y., Tanoue, M., Sasaki, O., Zhou, X. & Yamazaki, D. Global exposure to flooding from the new CMIP6 climate model projections. Sci. Rep. 11 , 3740 (2021).
United Nations. World Population Prospects 2019 Highlights (2019).
Ozturk, U. et al. How climate change and unplanned urban sprawl bring more landslides. Nature 608 , 262–265 (2022).
Aerts, J. C. J. H. et al. Integrating human behaviour dynamics into flood disaster risk assessment. Nat. Clim. Chang. 8 , 193–199 (2018).
Palash, W., Akanda, A. S. & Islam, S. The record 2017 flood in South Asia: state of prediction and performance of a data-driven requisitely simple forecast model. J. Hydrol. 589 , 125190 (2020).
di Baldassarre, G., Yan, K., Ferdous, M. D. R. & Brandimarte, L. The interplay between human population dynamics and flooding in Bangladesh: a spatial analysis. Proc. Int. Assoc. Hydrol. Sci. 364 , 188–191 (2014).
Penning-Rowsell, E. C., Sultana, P. & Thompson, P. M. The ‘last resort’? Population movement in response to climate-related hazards in Bangladesh. Environ. Sci. Policy 27 , S44–S59 (2013).
Ward, P. J. et al. Usefulness and limitations of global flood risk models. Nat. Clim. Chang. 5 , 712–715 (2015).
Smith, A., Sampson, C. & Bates, P. Regional flood frequency analysis at the global scale. Water Resour. Res. 51 , 539–553 (2015).
Coxon, G. et al. A novel framework for discharge uncertainty quantification applied to 500 UK gauging stations. Water Resour. Res. 51 , 5531–5546 (2015).
Pappenberger, F. et al. Influence of uncertain boundary conditions and model structure on flood inundation predictions. Adv. Water Resour. 29 , 1430–1449 (2006).
De Michele, C. & Rosso, R. Uncertainty assessment of regionalized flood frequency estimates. J. Hydrol. Eng. 6 , 453–459 (2001).
Sousa, P. M. et al. Trends and extremes of drought indices throughout the 20th century in the Mediterranean. Nat. Hazards Earth Syst. Sci. 11 , 33–51 (2011).
Wing, O. E. J. et al. Validation of a 30 m resolution flood hazard model of the conterminous United States. Water Resour. Res. 53 , 7968–7986 (2017).
Beck, H. E. et al. MSWEP V2 global 3-hourly 0.1° precipitation: methodology and quantitative assessment. Bull. Am. Meteorol. Soc. 100 , 473–500 (2019).
Kendon, E. J. et al. Do convection-permitting regional climate models improve projections of future precipitation change? Bull. Am. Meteorol. Soc. 98 , 79–93 (2017).
Kidd, C. et al. Intercomparison of high-resolution precipitation products over northwest Europe. J. Hydrometeorol. 13 , 67–83 (2012).
Slater, L. et al. Global changes in 20‐year, 50‐year, and 100‐year river floods. Geophys. Res. Lett. 48 , e2020GL091824 (2021).
Bertola, M. et al. Do small and large floods have the same drivers of change? A regional attribution analysis in Europe. Hydrol. Earth Syst. Sci. 25 , 1347–1364 (2021).
Bertola, M., Viglione, A., Lun, D., Hall, J. & Blöschl, G. Flood trends in Europe: are changes in small and big floods different? Hydrol. Earth Syst. Sci. 24 , 1805–1822 (2020).
Wasko, C. et al. Incorporating climate change in flood estimation guidance. Philos. Trans. R. Soc. A: Math., Phys. Eng. Sci. 379 , 20190548 (2021).
Kuklicke, C. & Demeritt, D. Adaptive and risk-based approaches to climate change and the management of uncertainty and institutional risk: the case of future flooding in England. Glob. Environ. Change 37 , 56–68 (2016).
Milly, P. C. D. et al. Stationarity is dead: whither water management?. Science 319 , 573–574 (2008).
Article CAS PubMed Google Scholar
Salas, J. D., Obeysekera, J. & Vogel, R. M. Techniques for assessing water infrastructure for nonstationary extreme events: a review. Hydrological Sci. J. 63 , 325–352 (2018).
Serinaldi, F. & Kilsby, C. G. Stationarity is undead: uncertainty dominates the distribution of extremes. Adv. Water Resour. 77 , 17–36 (2015).
Villarini, G. et al. Floods and Nonstationarity—A Review (2018).
Wright, D. B., Bosma, C. D. & Lopez‐Cantu, T. U.S. hydrologic design standards insufficient due to large increases in frequency of rainfall extremes. Geophys Res. Lett. 46 , 8144–8153 (2019).
Madsen, H., Lawrence, D., Lang, M., Martinkova, M. & Kjeldsen, T. R. Review of trend analysis and climate change projections of extreme precipitation and floods in Europe. J. Hydrol. 519 , 3634–3650 (2014).
Lopez-Cantu, T. & Samaras, C. Temporal and spatial evaluation of stormwater engineering standards reveals risks and priorities across the United States. Environ. Res. Lett. 13 , 074006 (2018).
François, B., Schlef, K. E., Wi, S. & Brown, C. M. Design considerations for riverine floods in a changing climate—a review. J. Hydrol. 574 , 557–573 (2019).
Bernhofen, M. V. et al. The role of global data sets for riverine flood risk management at national scales. Water Resour. Res. 58 , e2021WR031555 (2022).
Bevacqua, E. et al. Higher probability of compound flooding from precipitation and storm surge in Europe under anthropogenic climate change. Sci. Adv. 5 , eaaw5531 (2019).
Edmonds, D. A., Caldwell, R. L., Brondizio, E. S. & Siani, S. M. O. Coastal flooding will disproportionately impact people on river deltas. Nat. Commun. 11 , 4741 (2020).
Rentschler, J., Salhab, M. & Jafino, B. A. Flood exposure and poverty in 188 countries. Nat. Commun. 13 , 3527 (2022).
Nirandjan, S., Koks, E. E., Ward, P. J. & Aerts, J. C. J. H. A spatially-explicit harmonized global dataset of critical infrastructure. Sci. Data 9 , 150 (2022).
Rebally, A., Valeo, C., He, J. & Saidi, S. Flood impact assessments on transportation networks: a review of methods and associated temporal and spatial scales. Front. Sustain. Cities 3 , 732181 (2021).
Yamazaki, D. et al. MERIT hydro: a high‐resolution global hydrography map based on latest topography dataset. Water Resour. Res. 55 , 5053–5073 (2019).
Neal, J., Schumann, G. & Bates, P. A subgrid channel model for simulating river hydraulics and floodplain inundation over large and data sparse areas. Water Resour. Res. 48 , W11506 (2012).
Bates, P. D., Horritt, M. S. & Fewtrell, T. J. A simple inertial formulation of the shallow water equations for efficient two-dimensional flood inundation modelling. J. Hydrol. 387 , 33–45 (2010).
de Almeida, G. A. M., Bates, P., Freer, J. E. & Souvignet, M. Improving the stability of a simple formulation of the shallow water equations for 2-D flood modeling. Water Resour. Res. 48 , W05528 (2012).
Yamazaki, D. et al. A high-accuracy map of global terrain elevations. Geophys. Res. Lett. 44 , 5844–5853 (2017).
Wing, O. E. J. et al. Simulating historical flood events at the continental scale: observational validation of a large-scale hydrodynamic model. Nat. Hazards Earth Syst. Sci. 21 , 559–575 (2021).
Bates, P. D. et al. Combined modeling of US fluvial, pluvial, and coastal flood hazard under current and future climates. Water Resour. Res. 57 , e2020WR028673 (2021).
Wing, O. E. J. et al. Estimates of present and future flood risk in the conterminous United States. Environ. Res. Lett. 13 , 034023 (2018).
Stevens, F. R., Gaughan, A. E., Linard, C. & Tatem, A. J. Disaggregating census data for population mapping using random forests with remotely-sensed and ancillary data. PLoS ONE 10 , e0107042 (2015).
Leyk, S. et al. The spatial allocation of population: a review of large-scale gridded population data products and their fitness for use. Earth Syst. Sci. Data 11 , 1385–1409 (2019).
Download references
Acknowledgements
This work is funded as part of the Water Informatics Science and Engineering Centre for Doctoral Training (WISE CDT) under a grant from the Engineering and Physical Science Research Council (EPSRC), grant EP/L016214/1. J.N. was supported by NERC Grants NE/S003061/1 and NE/S006079/1. G.C. was supported by a UKRI Future Leaders Fellowship Award [MR/V022857/1]. Support to T.W. was provided by the Alexander von Humboldt Foundation in the framework of the Alexander von Humboldt Professorship endowed by the German Federal Ministry of Education and Research.
Author information
Authors and affiliations.
School of Geographical Sciences, University of Bristol, Bristol, UK
Laura Devitt, Jeffrey Neal & Gemma Coxon
Cabot Institute, University of Bristol, Bristol, UK
Jeffrey Neal, Gemma Coxon & Thorsten Wagener
Fathom, Bristol, UK
Jeffrey Neal & James Savage
Department of Civil Engineering, University of Bristol, Bristol, UK
Thorsten Wagener
Institute for Environmental Science and Geography, University of Potsdam, Potsdam, Germany
You can also search for this author in PubMed Google Scholar
Contributions
J.S. processed the global flood model data and provided the flood hazard data and inflow boundary conditions. L.D. conducted the sensitivity analysis and produced the figures, with input from J.N., G.C. and T.W. L.D. prepared the manuscript with contributions from all authors.
Corresponding author
Correspondence to Laura Devitt .
Ethics declarations
Competing interests.
The authors declare no competing interests.
Peer review
Peer review information.
Nature Communications thanks Giuliano Di Baldassarre and the other, anonymous, reviewer(s) for their contribution to the peer review of this work. Peer reviewer reports are available.
Additional information
Publisher’s note Springer Nature remains neutral with regard to jurisdictional claims in published maps and institutional affiliations.
Supplementary information
Supplementary information, peer review file, rights and permissions.
Open Access This article is licensed under a Creative Commons Attribution 4.0 International License, which permits use, sharing, adaptation, distribution and reproduction in any medium or format, as long as you give appropriate credit to the original author(s) and the source, provide a link to the Creative Commons license, and indicate if changes were made. The images or other third party material in this article are included in the article’s Creative Commons license, unless indicated otherwise in a credit line to the material. If material is not included in the article’s Creative Commons license and your intended use is not permitted by statutory regulation or exceeds the permitted use, you will need to obtain permission directly from the copyright holder. To view a copy of this license, visit http://creativecommons.org/licenses/by/4.0/ .
Reprints and permissions
About this article
Cite this article.
Devitt, L., Neal, J., Coxon, G. et al. Flood hazard potential reveals global floodplain settlement patterns. Nat Commun 14 , 2801 (2023). https://doi.org/10.1038/s41467-023-38297-9
Download citation
Received : 25 November 2022
Accepted : 19 April 2023
Published : 16 May 2023
DOI : https://doi.org/10.1038/s41467-023-38297-9
Share this article
Anyone you share the following link with will be able to read this content:
Sorry, a shareable link is not currently available for this article.
Provided by the Springer Nature SharedIt content-sharing initiative
This article is cited by
Limited progress in global reduction of vulnerability to flood impacts over the past two decades.
- Inga J. Sauer
- Benedikt Mester
- Christian Otto
Communications Earth & Environment (2024)
Integrating social vulnerability into high-resolution global flood risk mapping
- Felix Agyemang
- Jeffrey Neal
Nature Communications (2024)
By submitting a comment you agree to abide by our Terms and Community Guidelines . If you find something abusive or that does not comply with our terms or guidelines please flag it as inappropriate.
Quick links
- Explore articles by subject
- Guide to authors
- Editorial policies
Sign up for the Nature Briefing newsletter — what matters in science, free to your inbox daily.


An official website of the United States government
The .gov means it’s official. Federal government websites often end in .gov or .mil. Before sharing sensitive information, make sure you’re on a federal government site.
The site is secure. The https:// ensures that you are connecting to the official website and that any information you provide is encrypted and transmitted securely.
- Publications
- Account settings
Preview improvements coming to the PMC website in October 2024. Learn More or Try it out now .
- Advanced Search
- Journal List
- Indian J Community Med
- v.37(3); Jul-Sep 2012
Disaster Management in Flash Floods in Leh (Ladakh): A Case Study
Preeti gupta.
Regimental Medical Officer, Leh, Ladakh, India
Anurag Khanna
1 Commanding Officer, Army Hospital, Leh, India
2 Registrar, Army Hospital, Leh, India
Background:
On August 6, 2010, in the dark of the midnight, there were flash floods due to cloud burst in Leh in Ladakh region of North India. It rained 14 inches in 2 hours, causing loss of human life and destruction. The civil hospital of Leh was badly damaged and rendered dysfunctional. Search and rescue operations were launched by the Indian Army immediately after the disaster. The injured and the dead were shifted to Army Hospital, Leh, and mass casualty management was started by the army doctors while relief work was mounted by the army and civil administration.
The present study was done to document disaster management strategies and approaches and to assesses the impact of flash floods on human lives, health hazards, and future implications of a natural disaster.
Materials and Methods:
The approach used was both quantitative as well as qualitative. It included data collection from the primary sources of the district collectorate, interviews with the district civil administration, health officials, and army officials who organized rescue operations, restoration of communication and transport, mass casualty management, and informal discussions with local residents.
234 persons died and over 800 were reported missing. Almost half of the people who died were local residents (49.6%) and foreigners (10.2%). Age-wise analysis of the deaths shows that the majority of deaths were reported in the age group of 25–50 years, accounting for 44.4% of deaths, followed by the 11–25-year age group with 22.2% deaths. The gender analysis showed that 61.5% were males and 38.5% were females. A further analysis showed that more females died in the age groups <10 years and ≥50 years.
Conclusions:
Disaster preparedness is critical, particularly in natural disasters. The Army's immediate search, rescue, and relief operations and mass casualty management effectively and efficiently mitigated the impact of flash floods, and restored normal life.
Introduction
In the midnight of August 6, 2010, Leh in Ladakh region of North India received a heavy downpour. The cloud burst occurred all of a sudden that caught everyone unawares. Within a short span of about 2 h, it recorded a rainfall of 14 inches. There were flash floods, and the Indus River and its tributaries and waterways were overflowing. As many as 234 people were killed, 800 were injured, and many went missing, perhaps washed away with the gorging rivers and waterways. There was vast destruction all around. Over 1000 houses collapsed. Men, women, and children were buried under the debris. The local communication networks and transport services were severely affected. The main telephone exchange and mobile network system (BSNL), which was the lifeline in the far-flung parts of the region, was completely destroyed. Leh airport was flooded and the runway was covered with debris, making it non-functional. Road transport was badly disrupted as roads were washed away and blocked with debris at many places. The civil medical and health facilities were also severely affected, as the lone district civil hospital was flooded and filled with debris.
Materials and Methods
The present case study is based on the authors’ own experience of managing a natural disaster caused by the flash floods. The paper presents a firsthand description of a disaster and its prompt management. The data was collected from the records of the district civil administration, the civil hospital, and the Army Hospital, Leh. The approach used was both quantitative as well as qualitative. It included data collection from the primary sources of the district collectorate, interviews with the district civil administration and army officials who organized rescue operations, restoration of communication, and transport, mass casualty management, and informal discussions with local residents.
Disaster management strategies
Three core disaster management strategies were adopted to manage the crisis. These strategies included: i) Response, rescue, and relief operations, ii) Mass casualty management, and iii) Rehabilitation.
Response, rescue, and relief operations
The initial response was carried out immediately by the Government of India. The rescue and relief work was led by the Indian Army, along with the State Government of Jammu and Kashmir, Central Reserve Police Force (CRPF), and Indo-Tibetan Border Police (ITBP). The Indian Army activated the disaster management system immediately, which is always kept in full preparedness as per the standard army protocols and procedures.
There were just two hospitals in the area: the government civil hospital (SNM Hospital) and Army Hospital. During the flash floods, the government civil hospital was flooded and rendered dysfunctional. Although the National Disaster Management Act( 1 ) was in place, with the government civil hospital being under strain, the applicability of the act was hampered. The Army Hospital quickly responded through rescue and relief operations and mass casualty management. By dawn, massive search operations were started with the help of civil authorities and local people. The patients admitted in the civil hospital were evacuated to the Army Hospital, Leh in army helicopters.
The runway of Leh airport was cleared up within a few hours after the disaster so that speedy inflow of supplies could be carried out along with the evacuation of the casualties requiring tertiary level healthcare to the Army Command Hospital in Chandigarh. The work to make the roads operational was started soon after the disaster. The army engineers had started rebuilding the collapsed bridges by the second day. Though the main mobile network was dysfunctional, the other mobile network (Airtel) still worked with limited connectivity in the far-flung areas of the mountains. The army communication system was the main and the only channel of communication for managing and coordinating the rescue and relief operations.
Mass casualty management
All casualties were taken to the Army Hospital, Leh. Severely injured people were evacuated from distant locations by helicopters, directly landing on the helipad of the Army Hospital. In order to reinforce the medical staff, nurses were flown in from the Super Specialty Army Hospital (Research and Referral), New Delhi, to handle the flow of casualties by the third day following the disaster. National Disaster Cell kept medical teams ready in Chandigarh in case they were required. The mortuary of the government civil hospital was still functional where all the dead bodies were taken, while the injured were handled by Army Hospital, Leh.
Army Hospital, Leh converted its auditorium into a crisis expansion ward. The injured started coming in around 0200 hrs on August 6, 2010. They were given first aid and were provided with dry clothes. A majority of the patients had multiple injuries. Those who sustained fractures were evacuated to Army Command Hospital, Chandigarh, by the Army's helicopters, after first aid. Healthcare staff from the government civil hospital joined the Army Hospital, Leh to assist them. In the meanwhile, medical equipment and drugs were transferred from the flooded and damaged government civil hospital to one of the nearby buildings where they could receive the casualties. By the third day following the disaster, the operation theatre of the government civil hospital was made functional. Table 1 gives the details of the patients admitted at the Army Hospital.
Admissions in the Army Hospital, Leh

The analysis of the data showed that majority of the people who lost their lives were mainly local residents (49.6%). Among the dead, there were 10.3% foreign nationals as well [ Table 2 ]. The age-wise analysis of the deaths showed that the majority of deaths were reported in the age group 26–50 years, accounting for 44.4% of deaths, followed by 11–25 year group with 22.2% deaths.
Number of deaths according to status of residence

The gender analysis showed that 61.5% were males among the dead, and 38.5% were females. A further analysis showed that more females died in <10 years and ≥50 years age group, being 62.5% and 57.1%, respectively [ Table 3 ].
Age and sex distribution of deaths

Victims who survived the disaster were admitted to the Army Hospital, Leh. Over 90% of them suffered traumatic injuries, with nearly half of them being major traumatic injuries. About 3% suffered from cold injuries and 6.7% as medical emergencies [ Table 4 ].
Distribution according to nature of casualty among the hospitalized victims

Rehabilitation
Shelter and relief.
Due to flash floods, several houses were destroyed. The families were transferred to tents provided by the Indian Army and government and non-government agencies. The need for permanent shelter for these people emerged as a major task. The Prime Minister of India announced Rs. 100,000 as an ex-gratia to the next of kin of each of those killed, and relief to the injured. Another Rs. 100,000 each would be paid to the next of kin of the deceased from the Chief Minister's Relief Fund of the State Government.
Supply of essential items
The Army maintains an inventory of essential medicines and supplies in readiness as a part of routing emergency preparedness. The essential non-food items were airlifted to the affected areas. These included blankets, tents, gum boots, and clothes. Gloves and masks were provided for the persons who were working to clear the debris from the roads and near the affected buildings.
Water, sanitation, and hygiene
Public Health is seriously threatened in disasters, especially due to lack of water supply and sanitation. People having lost their homes and living in temporary shelters (tents) puts a great strain on water and sanitation facilities. The pumping station was washed away, thus disrupting water supply in the Leh Township. A large number of toilets became non-functional as they were filled with silt, as houses were built at the foothills of the Himalayan Mountains. Temporary arrangements of deep trench latrines were made while the army engineers made field flush latrines for use by the troops.
Water was stagnant and there was the risk of contamination by mud or dead bodies buried in the debris, thus making the quality of drinking water questionable. Therefore, water purification units were installed and established. The National Disaster Response Force (NDRF) airlifted a water storage system (Emergency Rescue Unit), which could provide 11,000 L of pure water. Further, super-chlorination was done at all the water points in the army establishments. To deal with fly menace in the entire area, anti-fly measures were taken up actively and intensely.
Food and nutrition
There was an impending high risk of food shortage and crisis of hunger and malnutrition. The majority of food supply came from the plains and low-lying areas in North India through the major transport routes Leh–Srinagar and Leh–Manali national highways. These routes are non-functional for most part of the winter. The local agricultural and vegetable cultivation has always been scanty due to extreme cold weather. The food supplies took a further setback due to the unpredicted heavy downpour. Food storage facilities were also flooded and washed away. Government agencies, nongovernmental organizations, and the Indian Army immediately established food supply and distribution system in the affected areas from their food stores and airlifting food supplies from other parts of the country.
There was a high risk of water-borne diseases following the disaster. Many human bodies were washed away and suspected to have contaminated water bodies. There was an increased fly menace. There was an urgent need to prevent disease transmission due to contaminated drinking water sources and flies. There was also a need to rehabilitate people who suffered from crush injuries sustained during the disaster. The public health facilities, especially, the primary health centers and sub-health centers, were not adequately equipped and were poorly connected by roads to the main city of Leh. Due to difficult accessibility, it took many hours to move casualties from the far-flung areas, worsening the crisis and rescue and relief operations. The population would have a higher risk of mental health problems like post-traumatic stress disorder, deprivation, and depression. Therefore, relief and rehabilitation would include increased awareness of the symptoms of post-traumatic stress disorder and its alleviation through education on developing coping mechanisms.
Economic impact
Although it would be too early to estimate the impact on economy, the economy of the region would be severely affected due to the disaster. The scanty local vegetable and grain cultivation was destroyed by the heavy rains. Many houses were destroyed where people had invested all their savings. Tourism was the main source of income for the local people in the region. The summer season is the peak tourist season in Ladakh and that is when the natural disaster took place. A large number of people came from within India and other countries for trekking in the region. Because of the disaster, tourism was adversely affected. The disaster would have a long-term economic impact as it would take a long time to rebuild the infrastructure and also to build the confidence of the tourists.
The floods put an immense pressure and an economic burden on the local people and would also influence their health-seeking behavior and health expenditure.
Political context
The disaster became a security threat. The area has a high strategic importance, being at the line of control with China and Pakistan. The Indian Army is present in the region to defend the country's borders. The civil administration is with the Leh Autonomous Hill Development Council (LAHDC) under the state government of Jammu and Kashmir.
Conclusions
It is impossible to anticipate natural disasters such as flash floods. However, disaster preparedness plans and protocols in the civil administration and public health systems could be very helpful in rescue and relief and in reducing casualties and adverse impact on the human life and socio economic conditions.( 2 ) However, the health systems in India lack such disaster preparedness plans and training.( 3 ) In the present case, presence of the Indian Army that has standard disaster management plans and protocols for planning, training, and regular drills of the army personnel, logistics and supply, transport, and communication made it possible to immediately mount search, rescue, and relief operations and mass casualty management. Not only the disaster management plans were in readiness, but continuous and regular training and drills of the army personnel in rescue and relief operations, and logistics and communication, could effectively facilitate the disaster management operations.
Effective communication was crucial for effective coordination of rescue and relief operations. The Army's communication system served as an alternative communication channel as the public communication and mobile network was destroyed, and that enabled effective coordination of the disaster operations.
Emergency medical services and healthcare within few hours of the disaster was critical to minimize deaths and disabilities. Preparedness of the Army personnel, especially the medical corps, readiness of inventory of essential medicines and medical supplies, logistics and supply chain, and evacuation of patients as a part of disaster management protocols effectively launched the search, rescue, and relief operations and mass casualty reduction. Continuous and regular training and drills of army personnel, health professionals, and the community in emergency rescue and relief operations are important measures. Emergency drill is a usual practice in the army, which maintains the competence levels of the army personnel. Similar training and drill in civil administration and public health systems in emergency protocols for rescue, relief, mass casualty management, and communication would prove very useful in effective disaster management to save lives and restore health of the people.( 2 – 4 )
Lessons learnt and recommendations
Natural disasters not only cause a large-scale displacement of population and loss of life, but also result in loss of property and agricultural crops leading to severe economic burden.( 3 – 6 ) In various studies,( 3 , 4 , 7 , 8 ) several shortcomings have been observed in disaster response, such as, delayed response, absence of early warning systems, lack of resources for mass evacuation, inadequate coordination among government departments, lack of standard operating procedures for rescue and relief, and lack of storage of essential medicines and supplies.
The disaster management operations by the Indian Army in the natural disaster offered several lessons to learn. The key lessons were:
- Response time is a critical attribute in effective disaster management. There was no delay in disaster response by the Indian Army. The rescue and relief operations could be started within 1 h of disaster. This was made possible as the Army had disaster and emergency preparedness plans and protocols in place; stocks of relief supplies and medicines as per standard lists were available; and periodic training and drill of the army personnel and medical corps was undertaken as a routine. The disaster response could be immediately activated.
- There is an important lesson to be learned by the civil administration and the public health system to have disaster preparedness plans in readiness with material and designated rescue officers and workers.
- Prompt activation of disaster management plan with proper command and coordination structure is critical. The Indian Army could effectively manage the disaster as it had standard disaster preparedness plans and training, and activated the system without any time lag. These included standard protocols for search, rescue, and evacuation and relief and rehabilitation. There are standard protocols for mass casualty management, inventory of essential medicines and medical supplies, and training of the army personnel.
- Hospitals have always been an important link in the chain of disaster response and are assuming greater importance as advanced pre-hospital care capabilities lead to improved survival-to-hospital rate.( 9 ) Role of hospitals in disaster preparedness, especially in mass casualty management, is important. Army Hospital, Leh emergency preparedness played a major role in casualty management and saving human lives while the civil district hospital had become dysfunctional due to damage caused by floods. The hospital was fully equipped with essential medicines and supplies, rescue and evacuation equipments, and command and communication systems.
- Standard protocols and disaster preparedness plans need to be prepared for the civil administration and the health systems with focus on Quick Response Teams inclusive of healthcare professionals, rescue personnel, fire-fighting squads, police detachments, ambulances, emergency care drugs, and equipments.( 10 ) These teams should be trained in a manner so that they can be activated and deployed within an hour following the disaster. “TRIAGE” has to be the basic working principle for such teams.
- Effective communication system is of paramount importance in coordination of rescue and relief operations. In the present case study, although the main network with the widest connectivity was extensively damaged and severely disrupted, the army's communication system along with the other private mobile network tided over the crisis. It took over 10 days for reactivation of the main mobile network through satellite communication system. Thus, it is crucial to establish the alternative communication system to handle such emergencies efficiently and effectively.( 2 , 11 )
- Disaster management is a multidisciplinary activity involving a number of departments/agencies spanning across all sectors of development.( 2 ) The National Disaster Management Authority of India, set up under National Disaster Management Act 2005,( 1 ) has developed disaster preparedness and emergency protocols. It would be imperative for the civil administration at the state and district levels in India to develop their disaster management plans using these protocols and guidelines.
- Health system's readiness plays important role in prompt and effective mass casualty management.( 2 ) Being a mountainous region, the Ladakh district has difficult access to healthcare, with only nine Primary Health Centers and 31 Health Sub-Centers.( 12 ) There is a need for strengthening health systems with focus on health services and health facility network and capacity building. More than that, primary healthcare needs to be augmented to provide emergency healthcare so that more and more lives can be saved.( 7 )
- Training is an integral part of capacity building, as trained personnel respond much better to different disasters and appreciate the need for preventive measures. Training of healthcare professionals in disaster management holds the key in successful activation and implementation of any disaster management plan. The Army has always had standard drills in all its establishments at regular intervals, which are periodically revised and updated. The civil administration and public health systems should regularly organize and conduct training of civil authorities and health professionals in order to be ready for action.( 1 – 4 )
- Building confidence of the public to avoid panic situation is critical. Community involvement and awareness generation, particularly that of the vulnerable segments of population and women, needs to be emphasized as necessary for sustainable disaster risk reduction. Increased public awareness is necessary to ensure an organized and calm approach to disaster management. Periodic mock drills and exercise in disaster management protocols in the general population can be very useful.( 1 , 3 , 4 )
Source of Support: Nil
Conflict of Interest: None declared.
- Alzheimer's disease & dementia
- Arthritis & Rheumatism
- Attention deficit disorders
- Autism spectrum disorders
- Biomedical technology
- Diseases, Conditions, Syndromes
- Endocrinology & Metabolism
- Gastroenterology
- Gerontology & Geriatrics
- Health informatics
- Inflammatory disorders
- Medical economics
- Medical research
- Medications
- Neuroscience
- Obstetrics & gynaecology
- Oncology & Cancer
- Ophthalmology
- Overweight & Obesity
- Parkinson's & Movement disorders
- Psychology & Psychiatry
- Radiology & Imaging
- Sleep disorders
- Sports medicine & Kinesiology
- Vaccination
- Breast cancer
- Cardiovascular disease
- Chronic obstructive pulmonary disease
- Colon cancer
- Coronary artery disease
- Heart attack
- Heart disease
- High blood pressure
- Kidney disease
- Lung cancer
- Multiple sclerosis
- Myocardial infarction
- Ovarian cancer
- Post traumatic stress disorder
- Rheumatoid arthritis
- Schizophrenia
- Skin cancer
- Type 2 diabetes
- Full List »
share this!
May 17, 2024
This article has been reviewed according to Science X's editorial process and policies . Editors have highlighted the following attributes while ensuring the content's credibility:
fact-checked
trusted source
More leptospirosis cases occur after floods, study shows
by K. W. Wesselink, University of Twente
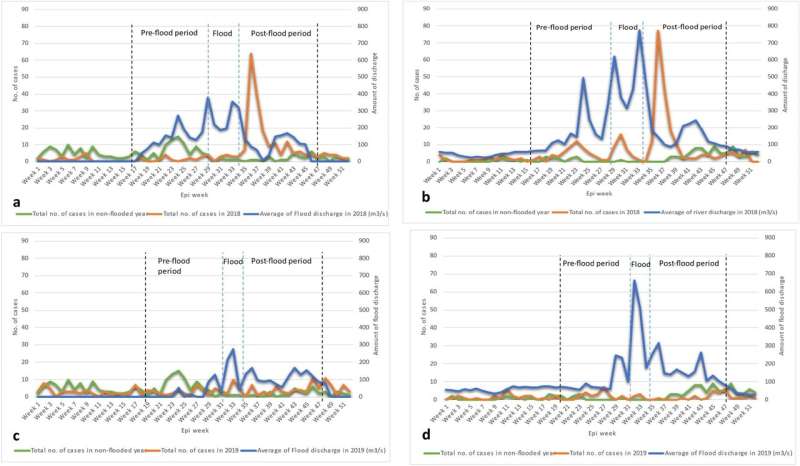
A recent study, published by researchers at the Faculty of ITC in the International Journal of Health Geographics , has shown that flooding leads to increased leptospirosis cases.
First author, John Ifejube is a recent graduate of the master's program in Spatial Engineering. This publication is a direct result of his thesis on GeoHealth.
Flooding is a climate-related disaster that impacts not only the environment but also the well-being of people. Leptospirosis is a blood infection caused by the bacteria Leptospira. People become infected through interaction with contaminated water or urine.
Infected humans can get headaches, muscle pains, and fevers, but severe forms can lead to kidney failure. More and more studies link the spread of leptospirosis with floods, but it has not been extensively studied, until now.
Master's thesis
For his master's thesis, Ifejube conducted a study on the association between the incidence of leptospirosis and the occurrence of multiple floods in Kerala, India. He found that flooding leads to an increase in leptospirosis cases. His study reveals that flood duration is the most important characteristic of floods that can be used to predict the number of infections. According to his research, severe floods lead to more leptospirosis cases than moderate floods.
He compared leptospirosis cases across three different years in time and space. Specifically, he compared the number of cases in 2018 and 2019, which were years with heavy and moderate flooding respectively, to the number of cases in 2017, a year that did not experience any floods.
For each flood year, he looked at the reported cases three months before, during and three months after the flood. To assess the severity of floods, he determined the population exposed to each flood event using high-resolution satellite images. Finally, he used spatial regression to examine the relationship between post-flood cases and flood extents.
Explore further
Feedback to editors

Sweet taste receptor affects how glucose is handled metabolically by humans

Better medical record-keeping needed to fight antibiotic overuse, studies suggest
7 hours ago

Repeat COVID-19 vaccinations elicit antibodies that neutralize variants, other viruses

A long-term ketogenic diet accumulates aged cells in normal tissues, new study shows
19 hours ago

Gut bacteria enhance cancer immunotherapy in mouse study
20 hours ago

Research finds the protein VISTA directly blocks T cells from functioning in immunotherapy

Study opens the door to designing therapies to improve lung development in growth-restricted fetuses
21 hours ago

Researchers make strides in microbiome-based cancer therapies via iron deprivation in the tumor microenvironment
22 hours ago

Study reveals that the brain modulates visual signals according to internal states

Limiting certain light exposure has potential to prevent inherited retinal dystrophy
Related stories.

What dog owners should know about leptospirosis
Apr 26, 2024

Researchers find the more flood driving factors there are, the more extreme a flood is
Mar 27, 2024

First the floods, then the diseases: Why NZ should brace for outbreaks of spillover infections from animals
Mar 8, 2023

Don't go wading in flood water if you can help it. It's health a risk for humans, and dogs too
Feb 28, 2022
Deadly 'rat fever' in flood-ravaged Indian state
Sep 4, 2018

Leptospirosis strains identified in Uruguay cattle
Sep 13, 2018
Recommended for you

Clinicians report success with first test of drug in a patient with life-threatening blood clotting disorder

Global life expectancy projected to increase by nearly 5 years by 2050 despite various threats
May 16, 2024

Researchers profile clinical, gene and protein changes in 'brain fog' from long COVID

Despite its 'nothingburger' reputation, COVID-19 remains deadlier than the flu
Let us know if there is a problem with our content.
Use this form if you have come across a typo, inaccuracy or would like to send an edit request for the content on this page. For general inquiries, please use our contact form . For general feedback, use the public comments section below (please adhere to guidelines ).
Please select the most appropriate category to facilitate processing of your request
Thank you for taking time to provide your feedback to the editors.
Your feedback is important to us. However, we do not guarantee individual replies due to the high volume of messages.
E-mail the story
Your email address is used only to let the recipient know who sent the email. Neither your address nor the recipient's address will be used for any other purpose. The information you enter will appear in your e-mail message and is not retained by Medical Xpress in any form.
Newsletter sign up
Get weekly and/or daily updates delivered to your inbox. You can unsubscribe at any time and we'll never share your details to third parties.
More information Privacy policy
Donate and enjoy an ad-free experience
We keep our content available to everyone. Consider supporting Science X's mission by getting a premium account.
E-mail newsletter
Summer of 2023 was hottest in 2,000 years, study finds

- Show more sharing options
- Copy Link URL Copied!
An extreme summer marked by deadly heat waves, explosive wildfires and record warm ocean temperatures will go down as among the hottest in the last 2,000 years, new research has found.
The summer of 2023 saw the temperature in the Northern Hemisphere soar 3.72 degrees above the average from 1850 to 1900, when modern instrumental recordkeeping began, according to a study published Tuesday in the journal Nature. The study focused on surface air temperatures across the extra-tropical region, which sits at 30 to 90 degrees north latitude and includes most of Europe and North America.
June, July and August last year were also 3.96 degrees warmer than the average from the years 1 through 1890, which the researchers calculated by combining observed records with tree ring records from nine global regions.
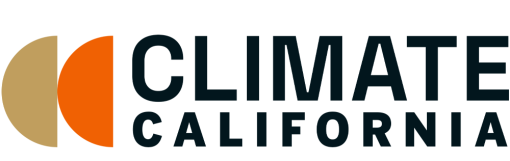
Aggressive and impactful reporting on climate change, the environment, health and science.
Jan Esper, the study’s lead author and a professor of climate geography at Johannes Gutenberg University in Germany, said that he was not expecting summer last year to be quite so anomalous, but that he was ultimately not surprised by the findings. The high temperatures built on an overall warming trend driven by greenhouse gas emissions and were further amplified by the onset of El Niño in the tropical Pacific.
“It’s no surprise — this really, really outstanding 2023 — but it was also, step-wise, a continuation of a trend that will continue,” Esper told reporters Monday. “Personally I’m not surprised, but I am worried.”
He said it was important to place 2023’s temperature extreme in a long-term context. The difference between the region’s previous warmest summer, in the year 246, and the summer of 2023 is 2.14 degrees, the study found.
The heat is even more extreme when compared with the region’s coldest summers — the majority of which were influenced by volcanic eruptions that spewed heat-blocking sulfur into the stratosphere. According to the study, 2023’s summer was 7.07 degrees warmer than the coldest reconstructed summer from this period, in the year 536.
“Although 2023 is consistent with a greenhouse gases-induced warming trend that is amplified by an unfolding El Niño event, this extreme emphasizes the urgency to implement international agreements for carbon emission reduction,” the study says.
The sweltering summer temperatures contributed to scores of heat illnesses and deaths, including at least 645 heat-associated deaths in Maricopa County, Ariz., where Phoenix saw temperatures of 110 degrees or hotter for a record 31 consecutive days.
Wildfires exacerbated by high temperatures raged across Canada and sent hazardous smoke down the East Coast of the United States and across the Atlantic. Meanwhile, ocean temperatures off Florida soared above 101 degrees , the temperature of a hot tub.
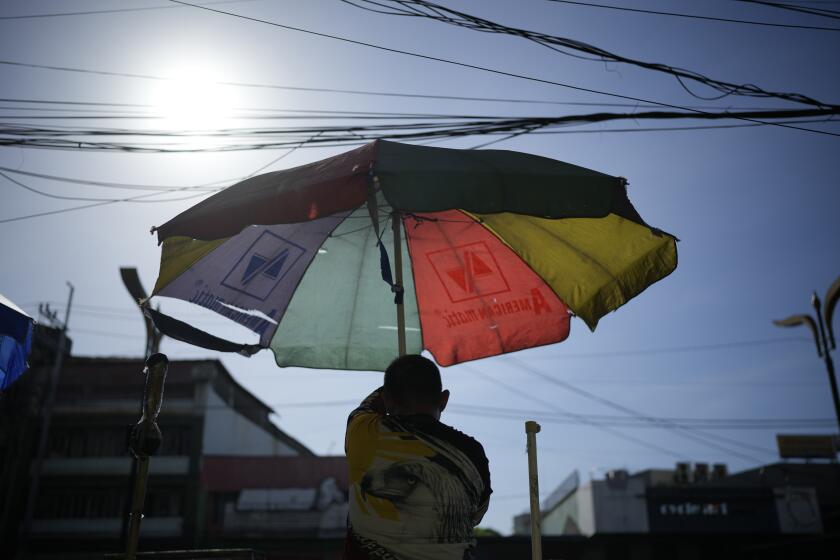
Climate & Environment
Warmest April on record extends planet’s hot streak to 11 months
With an average surface temperature of 59.05 degrees, the month was about 0.25 of a degree warmer than the previous hottest April, in 2016.
May 8, 2024
Multiple climate agencies, including the National Oceanic and Atmospheric Administration and the European Union’s Copernicus Climate Change Service, have declared 2023 the hottest year on record globally.
Notably, Copernicus found that the summer months of June, July and August last year measured 1.18 degrees warmer than average — still hot, but not nearly as warm as the study’s findings for the Northern Hemisphere’s extra-tropical region.
That region was especially hot in part because it is home to so much land, which warms faster than oceans, said Karen McKinnon, an assistant professor of statistics and the environment at UCLA who did not work on the study. (June, July and August are also winter months in the Southern Hemisphere.)
McKinnon said the study’s findings are not unexpected, as there was already good evidence that the summer of 2023 was record-breaking when compared with measurable data going back to the mid-1800s. But by going back 2,000 years, the researchers also helped illuminate “the full range of natural variability that could have occurred in the past,” she said.
She noted that tree rings can serve as a helpful proxy for climate conditions in the past, as trees tend to grow more in a given year if they receive the right amount of warmth, water and sunshine. But although last year’s heat was undeniable, the study also underscores that the summer temperature in this region was notably higher than the global target of 2.7 degrees — or 1.5 degrees Celsius — of warming over the preindustrial period, which was established by the Intergovernmental Panel on Climate Change in 2015.
It also notes that some recent research has found the data used to calculate that baseline may be off by several tenths of a degree, meaning it could need to be recalibrated, with the target landing closer to an even more challenging 1.6 or 1.7 degrees.
“I don’t think we should use the proxy instead of the instrumental data, but there’s a good indication that there’s a warm bias,” Esper said. “Further research is needed.”
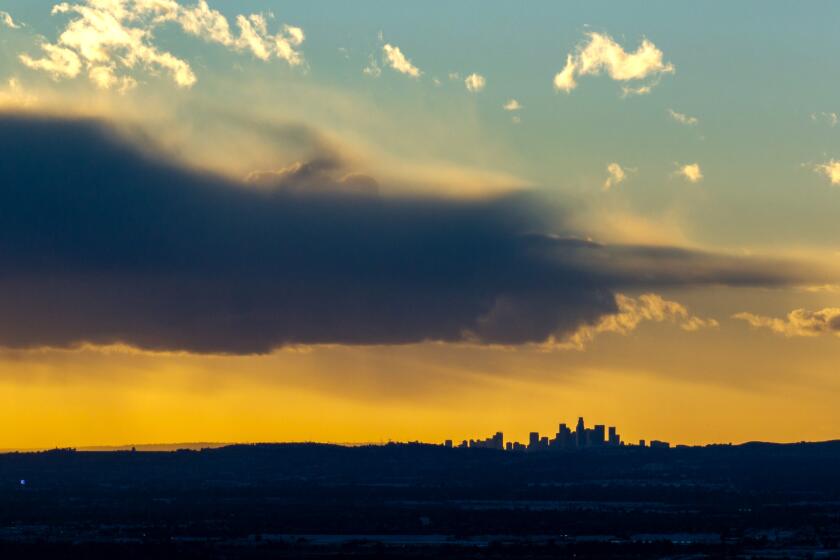
World & Nation
The planet is dangerously close to this climate threshold. Here’s what 1.5°C really means
Every bit of planetary warming will have impacts beyond those already occurring, including biodiversity loss, longer heat waves and extreme rainfall.
Feb. 1, 2024
McKinnon said there is always going to be some degree of uncertainty when comparing present-day temperatures to past temperatures, but that the 1.5-degree limit is as symbolic as it is literal. Many effects of climate change, including worsening heat waves, have already begun.
“There are definitely tipping points in the climate system, but we don’t understand the climate system well enough to say 1.5 C is the temperature for certain tipping points,” she said. “This is just a policy goal that gives you a temperature change that maybe would be consistent with averting some damages.”
In fact, the study’s publication comes days after a survey of 380 leading scientists from the IPCC revealed deep concerns about the world’s ability to limit global warming to 1.5 degrees. That report, published last week in the Guardian , found that only 6% of surveyed scientists think the 1.5-degree limit will be met. Nearly 80% said they foresee at least 2.5 degrees Celsius of warming.
The report caused a stir among the scientific community, with some saying it focused too heavily on pessimism and despair. But Daniel Swain, a climate scientist with UCLA who participated in the survey, said its findings are worthy of consideration.
“There are many kinds of scientists, myself included, who are very worried and concerned and increasingly alarmed by what is going on and what the data is showing,” Swain said during a briefing Friday . “But if anything, I think that really results in a stronger sense of resolve and urgency to do even more, and to do better.”
Indeed, while scientists continue to weigh in on whether — or how quickly — humanity can alter the planet’s worsening warming trajectory, Esper said he hopes the latest study will serve as motivation for changing outdated modes of energy consumption that contribute to planet-warming greenhouse gases.
“I am concerned about global warming — I think it’s one of the biggest threats out there,” he said.
He added that he is particularly worried for his children and for younger generations who will bear the brunt of adverse climate outcomes. There is a strong likelihood that the summer of 2024 will be even hotter, the study says.
“The longer we wait, the more extensive it will be, and the more difficult it will be to mitigate or even stop that process and reverse it,” Esper said. “It’s just so obvious: We should do as much as possible, as soon as possible.”
Toward a more sustainable California
Get Boiling Point, our newsletter exploring climate change, energy and the environment, and become part of the conversation — and the solution.
You may occasionally receive promotional content from the Los Angeles Times.
More to Read
Flooding in Americas, brutal heat in Asia and Africa: Extreme weather across the globe
May 10, 2024
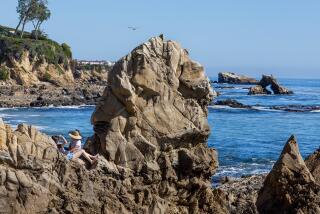
Summer heat is coming. Here’s a new interactive tool to help you deal with your health conditions
April 24, 2024
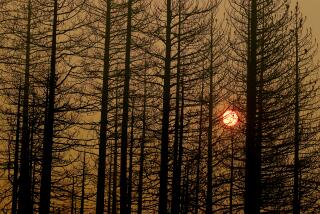
Climate change supercharged a heat dome, intensifying 2021 fire season, study finds
April 22, 2024
Start your day right
Sign up for Essential California for news, features and recommendations from the L.A. Times and beyond in your inbox six days a week.
Hayley Smith is an environment reporter for the Los Angeles Times, where she covers the many ways climate change is reshaping life in California, including drought, floods, wildfires and deadly heat.
More From the Los Angeles Times
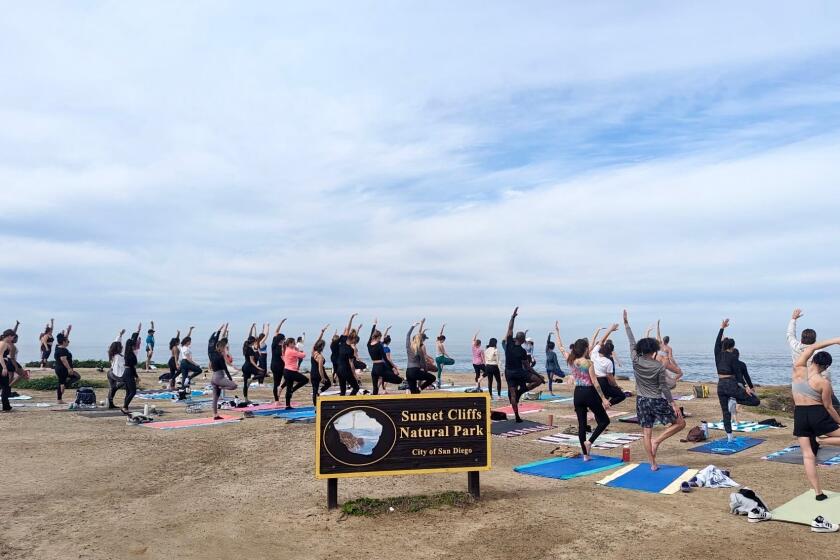
Namaste away: Rangers bar yoga classes at cliffside San Diego park
May 18, 2024
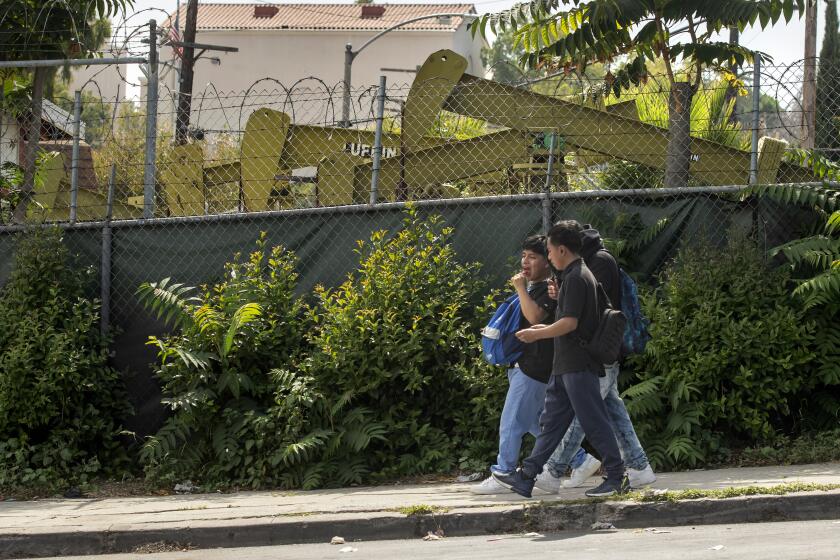
California’s effort to plug abandoned, chemical-spewing oil wells gets $35-million boost
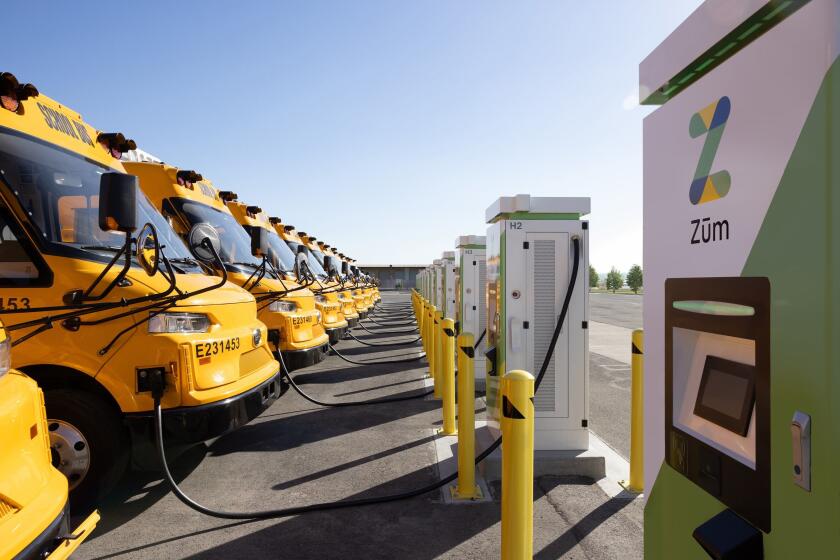
California school district becomes first in nation to go all electric buses
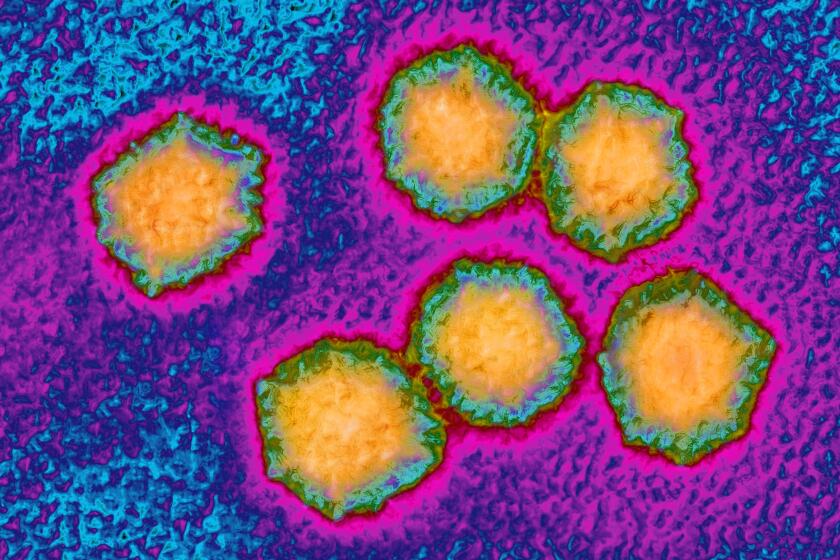
L.A. County investigating reported hepatitis A case at Beverly Hills Whole Foods
May 17, 2024

IMAGES
VIDEO
COMMENTS
Recent floods are also revealing vulnerabilities in how communities are designed and managed. ... case studies show that: Floods can damage resources that are essential in flood recovery, ...
The Missouri River and North Central Flood were the result of a powerful storm that occurred near the end of the wettest 12-month period on record in the U.S. (May 2018 - May 2019). CS_55, CS_56 The storm struck numerous states, specifically Nebraska (see Figure 1), Iowa, Missouri, South Dakota, North Dakota, Minnesota, Wisconsin, and Michigan.
A more recent study documents the worrying trend of increasing flood exposure using satellite data for 2000 to 2018, though omits at-risk populations who remained unaffected during the study ...
Although a previous analysis of eight case studies 21 identified vulnerability as ... whereas in one case (floods in Jakarta, Indonesia in 2002 and 2007 (ID 18)) there was a large increase in ...
Early projections were mostly limited to changes in flood hazard 163. More recent studies include exposure and ... U. Flood risks and impacts: a case study of Thailand's floods in 2011 and ...
Climate change is making extreme flooding worse, and a study published Wednesday in the journal Nature concluded the population exposed to those floods since 2000 is 10 times higher than previous ...
This recent study examined coastal flooding along the Eastern and Gulf coasts of the US, and did not include the Great Lakes. However, the report concludes that extreme weather events and floods are occurring nationwide, and that other coastal communities are also at higher risk. Coastal communities and states, and the nation as a whole, need to prepare for near-term changes in tidal flooding ...
As well as the large natural disasters, chronic smaller floods cause financial strain. Indeed, Merz et al had shown that higher probability, low damage events had enacted more strain in case studies of riverine flooding [114]. Regrettably, the costs of lower magnitude and frequent urban floods are not as well documented [128]. The reason may be ...
A lack of recent floods in the community, in turn, may impede the emergence of community ... Flood experience has long been acknowledged as a driver for community engagement in flood management. This case study illustrates how specific flood events served as catalysts for two local groups' bottom-up involvement in flood risk management in the ...
This section presents the review of each case study about risk of flooding, history and current standing of flood management systems, and their institutional framework affecting the flood management processes. 5.1. New York City. ... In recent times, local governments have assumed a greater share of the burden of developing resilient ...
The white paper found that the most consequential flooding effects were on housing safety and stability. In addition to water damage and lost possessions, worsened mental and physical health are key consequences of flooding. The report found that recovery in the aftermath of flooding can be costly, isolating, and time consuming.
Devastating floods in Pakistan affected 33 million people in 2022, with 8 million displaced, 13,000 injured and 1,700 killed - the latest in a series of increasingly frequent and severe climate-induced disasters. This case study explores how the empowered UN Resident Coordinator (RC) system was invaluable for responding to the complex crisis.
That has resulted in one of the worst floods ever recorded in Assam. As of 3 July, flood has affected 1,835,551 people in 26 out of the state's 35 districts and it submerged 471.98 square kilometers (47,198.87 hectares) of cropland. [1] About 200 people have died so far. Earlier on 22 June the floods had affected 5,457,601 people in 32 ...
It should be noted that urban flooding threat is not unique to China as evidenced by urban floods in recent years in the United States (National Academies of Science, Engineering, and Medicine [NASEM], 2019), in Germany (Bosseler et al., 2021), in India (Gupta, 2020), and in Thailand (Jular, 2017), and lessons learned from this study should be ...
Oct 19, 2021. --. Manimalayar witnesses biggest flood in 64 years. Being a coastal state of India and much of the land being part of the fragile Western Ghats, Kerala is very sensitive to climate ...
Floods are the most frequent type of weather-related disaster, accounting for about 47% of all weather-related disasters from 1995 to 2015 [1, 2].Between 1995 and 2015, more than 2.3 billion people were affected by flood disasters, with over 157 thousand people dying directly as a result of floods [].In recent years, many intense urban flooding events have been recorded in the UK, resulting in ...
Accurate early warning systems are crucial for reducing damages caused by flooding events. In this study, we demonstrate the potential of Long Short-Term Memory Networks for enhancing the forecast accuracy of hydrologic models employed in operational flood forecasting.
One study using coarse flood data projected that the global number of flood-exposed people will reach 1.3 billion by 2050 (ref. 28), but a more recent high-resolution study showed that this ...
Some of the recent case studies about Chennai floods of December 2015 investigated the impact of climate change based on extreme rainfall data and the relationship with the global phenomenon like El-Nino and La-Nina, but the observations are contrary because of different data sets and methodologies for analysis (Krishnamurthy et al. 2018; Boyaj ...
In this study, we present the application of remote sensing observations to map flooded areas, using the Vembanad-Kol-Wetland system in the southwest of India as a case study. In August 2018, this region experienced an extremely heavy monsoon season, which caused once-in-a-century floods that led to nearly 500 deaths and the displacement of ...
The devastating flooding across the UK in February 2020 was remarkable in terms of the combination of duration, scale and severity, according to a preliminary analysis by the UK Centre for Ecology & Hydrology.Three successive storms - Ciara on February 8 and 9, Dennis on February 15 and 16, and Jorge from February 28 to March 1 - resulted ...
UK Floods Case Study November 2019. The UK experienced an extreme weather event in November 2019 when exceptionally heavy rainfall caused flooding in parts of the UK. Heavy downpours across large parts of northern England led to surface water and river flooding in parts of Yorkshire, Nottinghamshire, Greater Manchester, Derbyshire and ...
India's Information Technology (IT) hub, Bengaluru, was recently in the limelight as it struggled to cope up with the recent urban floods triggered by the incessant rains in the last week of August and first week of September this year.. With a 131.6 millimeter rain in September this year, breaking records of the last 34 years, several IT parks in areas like Eco Space and other residential ...
Item 1 of 5 People walk through flood water caused by heavy rains, in Dubai, United Arab Emirates, April 17, 2024. ... "Rainfall from thunderstorms, like the ones seen in UAE in recent days, sees ...
However, enhancing community flood resilience presents numerous obstacles and potential downsides. To address such a challenge, this dissertation investigates the interactions among natural, built environment, and actor systems using a case study of flooding in Jakarta, Indonesia, focusing on the Ciliwung river watershed.
A recent study of flood risk and its interaction with poverty 97 included fluvial, coastal and pluvial flood hazard types in its estimate of global flood exposure. The authors found 1.8 billion ...
The present case study is based on the authors' own experience of managing a natural disaster caused by the flash floods. The paper presents a firsthand description of a disaster and its prompt management. The data was collected from the records of the district civil administration, the civil hospital, and the Army Hospital, Leh.
A recent study, published by researchers at the Faculty of ITC in the International Journal of Health Geographics, has shown that flooding leads to increased leptospirosis cases.
This study aimed to examine post-flood management, with a particular focus on enhancing the inclusivity of marginalised communities through stakeholder analysis. This study was based on an interpretivist mixed method approach, under which 30 semi-structured stakeholder interviews were conducted. Interest versus power versus actual engagement matrix, social network analysis, and thematic ...
In fact, the study's publication comes days after a survey of 380 leading scientists from the IPCC revealed deep concerns about the world's ability to limit global warming to 1.5 degrees.