
An official website of the United States government
The .gov means it's official. Federal government websites often end in .gov or .mil. Before sharing sensitive information, make sure you're on a federal government site.
The site is secure. The https:// ensures that you are connecting to the official website and that any information you provide is encrypted and transmitted securely.
- Publications
- Account settings
- Browse Titles
NCBI Bookshelf. A service of the National Library of Medicine, National Institutes of Health.
National Academies of Sciences, Engineering, and Medicine; Division on Earth and Life Studies; Water Science and Technology Board; Policy and Global Affairs; Program on Risk, Resilience, and Extreme Events; Committee on Urban Flooding in the United States. Framing the Challenge of Urban Flooding in the United States. Washington (DC): National Academies Press (US); 2019 Mar 29.


Framing the Challenge of Urban Flooding in the United States.
- Hardcopy Version at National Academies Press
Appendix D Chicago Case Study
For the Chicago case study, the committee convened a workshop in Chicago, Illinois, followed by site visits to three Chicago locations. Additional information was collected from some participants via telephone interviews. The workshop was structured to gather information from local, state, regional, and federal stakeholders. Participants were divided into small working groups to address four aspects of urban flooding:
- Physical aspects of urban flooding (built and natural environment),
- Social aspects of urban flooding (people and institutions),
- Data and informational aspects of urban flooding (forecasts, maps, demographics), and
- Actions and decision-making aspects of urban flooding.
Detailed comments from each working group conversation are available at http://nationalacademies.org/Urban-Flooding-Visits .
- WORKSHOP AGENDA
Kimpton Hotel Allegro Chicago, Illinois September 19, 2017
View in own window
- SITE VISITS
July 5, 2017
- CASE STUDY PARTICIPANTS
The following individuals participated in the workshop, Chicago site visits, and/or in subsequent telephone interviews:
- Bulent Agar, Department of Water Management, City of Chicago
- Yvette Alexander-Maxie, American Red Cross
- Dana Al-Qadi, AECOM
- Nora June Beck, Chicago Metropolitan Agency for Planning
- Marcella Bondie Keenan, Center for Neighborhood Technology
- David Bucaro, U.S. Army Corps of Engineers (USACE)
- Shannon Burke, American Planning Association
- Lori Burns, RainReady Chatham
- Anthony Comerio, Hanson Professional Services, Inc.
- Kathleen Dickhut, Department of Planning, City of Chicago
- Mike Drake, Department of Transportation, City of Chicago
- Edward Fenelon, National Oceanic and Atmospheric Administration
- Joan Frietag, Hanson Professional Services, Inc.
- Danielle Gallet, Metropolitan Planning Council
- Ludovica Gazze, University of Chicago
- Peter Haas, Center for Neighborhood Technology
- Beth Hall, Midwestern Regional Climate Center
- Jenny Hwang, Humanitarian Disaster Institute
- Ora Jackson, RainReady Chatham
- Edde Jones, Department of Transportation, City of Chicago
- Katherine Jordan, Zurich North America
- Karen Kreis, Village of Midlothian
- Curtis McKinney, Department of Transportation, City of Chicago
- Peter Mulvaney, West Monroe Partners
- Paul Osman, Illinois Office of Water Resources
- Chris Parker, Floodlothian Midlothian
- Joshua Peschel, Iowa State University
- Marcus Quigley, OptiRTC
- Jennifer Rath, Allstate Insurance Company
- Aaron Reisinger, USACE
- Roderick Sawyer, Chatham
- Brendan Schreiber, Department of Water Management, City of Chicago
- James Schwab, American Planning Association
- Krys Shaw, Office of Congressman Mike Quigley
- Tom Sivak, Office of Emergency Management and Communications
- Hal Sprague, Center for Neighborhood Technology
- Kari Steele, Metropolitan Water Reclamation District of Greater Chicago
- Dawn Thompson-Ellis, Center for Neighborhood Technology
- Anapam Verma, Department of Water Management, City of Chicago
- Zach Vernon, Chicago Metropolitan Agency for Planning
- Cheryl Watson, RainReady Chatham
- John Watson, Metropolitan Water Reclamation District of Greater Chicago
- Cite this Page National Academies of Sciences, Engineering, and Medicine; Division on Earth and Life Studies; Water Science and Technology Board; Policy and Global Affairs; Program on Risk, Resilience, and Extreme Events; Committee on Urban Flooding in the United States. Framing the Challenge of Urban Flooding in the United States. Washington (DC): National Academies Press (US); 2019 Mar 29. Appendix D, Chicago Case Study.
- PDF version of this title (11M)
In this Page
Recent activity.
- Chicago Case Study - Framing the Challenge of Urban Flooding in the United State... Chicago Case Study - Framing the Challenge of Urban Flooding in the United States
Your browsing activity is empty.
Activity recording is turned off.
Turn recording back on
Connect with NLM
National Library of Medicine 8600 Rockville Pike Bethesda, MD 20894
Web Policies FOIA HHS Vulnerability Disclosure
Help Accessibility Careers

Along with Stanford news and stories, show me:
- Student information
- Faculty/Staff information
We want to provide announcements, events, leadership messages and resources that are relevant to you. Your selection is stored in a browser cookie which you can remove at any time using “Clear all personalization” below.
Rising seas and extreme storms fueled by climate change are combining to generate more frequent and severe floods in cities along rivers and coasts, and aging infrastructure is poorly equipped for the new reality. But when governments and planners try to prepare communities for worsening flood risks by improving infrastructure, the benefits are often unfairly distributed.
A new modeling approach from Stanford University and University of Florida researchers offers a solution: an easy way for planners to simulate future flood risks at the neighborhood level under conditions expected to become commonplace with climate change, such as extreme rainstorms that coincide with high tides elevated by rising sea levels.
The approach, described May 28 in Environmental Research Letters , reveals places where elevated risk is invisible with conventional modeling methods designed to assess future risks based on data from a single past flood event. “Asking these models to quantify the distribution of risk along a river for different climate scenarios is kind of like asking a microwave to cook a sophisticated souffle. It’s just not going to go well,” said senior study author Jenny Suckale, an associate professor of geophysics at the Stanford Doerr School of Sustainability . “We don’t know how the risk is distributed, and we don’t look at who benefits, to which degree.”
Helping other flood-prone communities
The new approach to modeling flood risk can help city and regional planners create better flood risk assessments and avoid creating new inequities, Suckale said. The algorithm is publicly available for other researchers to adapt to their location.
A history of destructive floods
The new study came about through collaboration with regional planners and residents in bayside cities including East Palo Alto, which faces rising flood risks from the San Francisco Bay and from an urban river that snakes along its southeastern border.
The river, known as the San Francisquito Creek, meanders from the foothills above Stanford’s campus down through engineered channels to the bay – its historic floodplains long ago developed into densely populated cities. “We live around it, we drive around it, we drive over it on the bridges,” said lead study author Katy Serafin , a former postdoctoral scholar in Suckale’s research group.
The river has a history of destructive floods. The biggest one, in 1998, inundated 1,700 properties, caused more than $40 million in damages, and led to the creation of a regional agency tasked with mitigating future flood risk.
Nearly 20 years after that historic flood, Suckale started thinking about how science could inform future flood mitigation efforts around urban rivers like the San Francisquito when she was teaching a course in East Palo Alto focused on equity, resilience, and sustainability in urban areas. Designated as a Cardinal Course for its strong public service component, the course was offered most recently under the title Shaping the Future of the Bay Area .
Around the time Suckale started teaching the course, the regional agency – known as the San Francisquito Creek Joint Powers Authority – had developed plans to redesign a bridge to allow more water to flow underneath it and prevent flooding in creekside cities. But East Palo Alto city officials told Suckale and her students that they worried the plan could worsen flood risks in some neighborhoods downstream of the bridge.
Suckale realized that if the students and scientists could determine how the proposed design would affect the distribution of flood risks along the creek, while collaborating with the agency to understand its constraints, then their findings could guide decisions about how to protect all neighborhoods. “It’s actionable science, not just science for science’s sake,” she said.
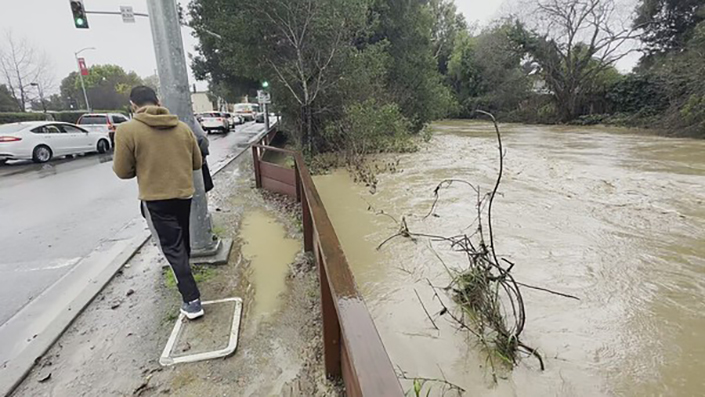
San Francisquito Creek waters rose along a temporary wooden floodwall in East Palo Alto, California, during a storm event on Dec. 31, 2022. | Jim Wiley, courtesy of the San Francisquito Creek Joint Powers Authority
Science that leads to action
The Joint Powers Authority had developed the plan using a flood-risk model commonly used by hydrologists around the world. The agency had considered the concerns raised by East Palo Alto city staff about downstream flood risks, but found that the standard model couldn’t substantiate them.
“We wanted to model a wider range of factors that will contribute to flood risk over the next few decades as our climate changes,” said Serafin, who served as a mentor to students in the Cardinal Course and is now an assistant professor at University of Florida.
Serafin created an algorithm to simulate millions of combinations of flood factors, including sea-level rise and more frequent episodes of extreme rainfall – a consequence of global warming that is already being felt in East Palo Alto and across California .
Serafin and Suckale incorporated their new algorithm into the widely used model to compute the statistical likelihood that the San Francisquito Creek would flood at different locations along the river. They then overlaid these results with aggregated household income and demographic data and a federal index of social vulnerability .
They found that the redesign of the upstream bridge would provide adequate protection against a repeat of the 1998 flood, which was once considered a 75-year flood event. But the modeling revealed that the planned design would leave hundreds of low-income households in East Palo Alto exposed to increased flood risk as climate change makes once-rare severe weather and flood events more common.
Related story
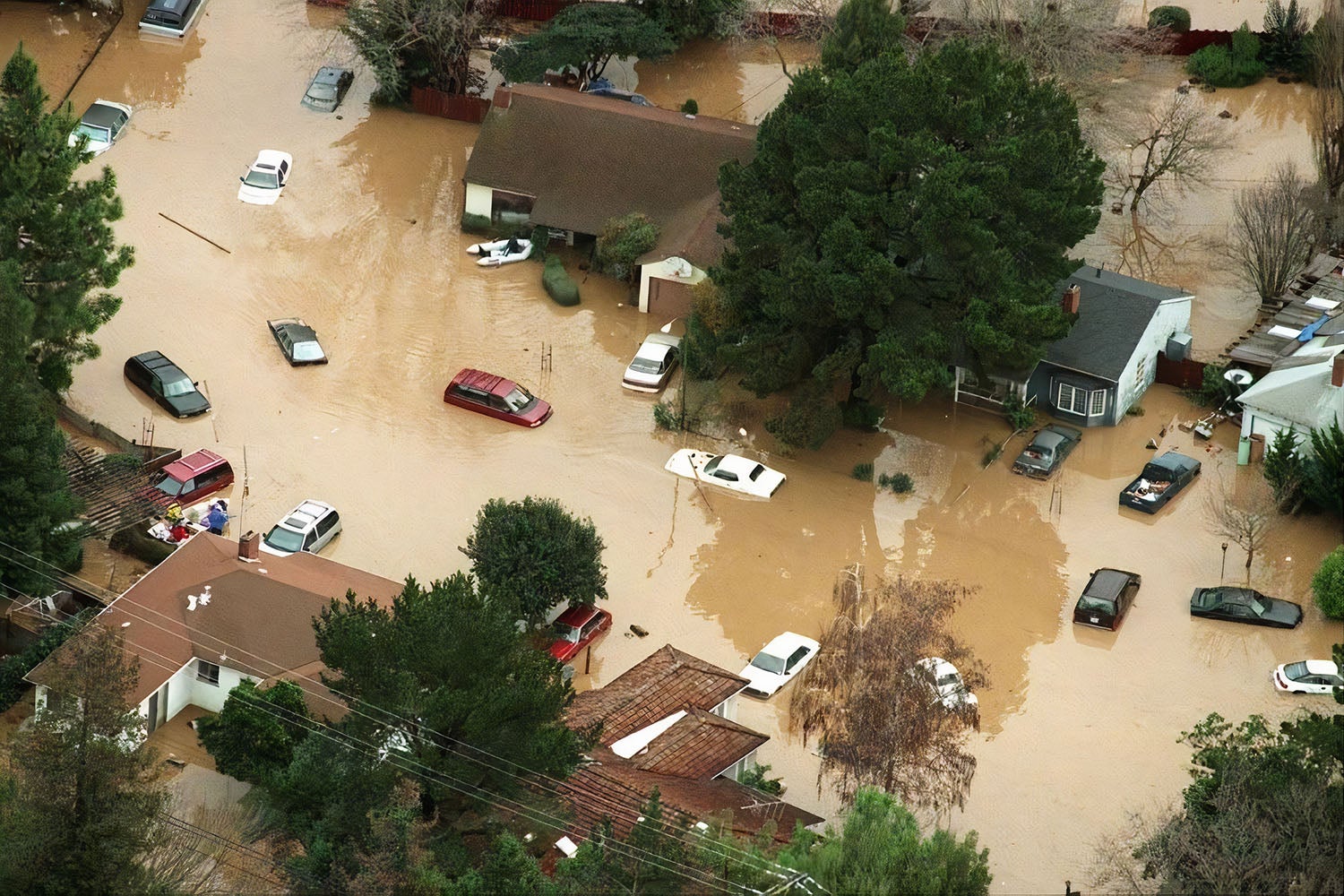
Sea-level rise may worsen existing Bay Area inequities
A beneficial collaboration.
When the scientists shared their findings with the city of East Palo Alto, the Joint Powers Authority, and other community collaborators in conversations over several years, they emphasized that the conventional model wasn’t wrong – it just wasn’t designed to answer questions about equity.
The results provided scientific evidence to guide the Joint Powers Authority’s infrastructure plans, which expanded to include construction of a permanent floodwall beside the creek in East Palo Alto. The agency also adopted a plan to build up the creek’s bank in a particularly low area to better protect neighboring homes and streets.
Ruben Abrica, East Palo Alto’s elected representative to the Joint Powers Authority board, said researchers, planners, city staff, and policymakers have a responsibility to work together to “carry out projects that don’t put some people in more danger than others.”
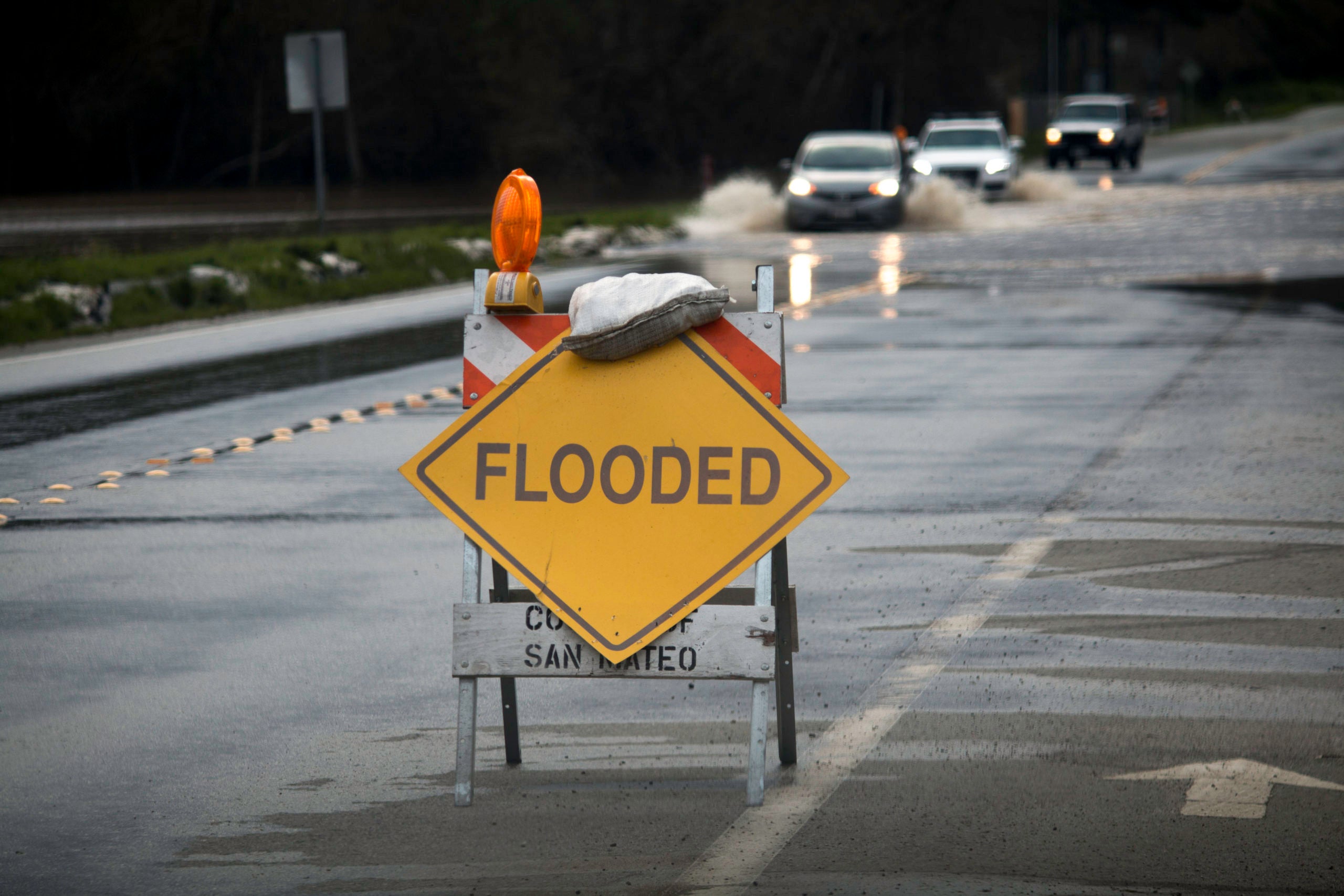
Bay Area coastal flooding triggers regionwide commute disruptions
The results of the Stanford research demonstrated how seemingly neutral models that ignore equity can lead to uneven distributions of risks and benefits. “Scientists have to become more aware of the impact of the research, because the people who read the research or the people who then do the planning are relying on them,” he said.
Serafin and Suckale said their work with San Francisquito Creek demonstrates the importance of mutual respect and trust among researchers and communities positioned not as subjects of study, but active contributors to the creation of knowledge. “Our community collaborators made sure we, as scientists, understood the realities of these different communities,” Suckale said. “We’re not training them to be hydrological modelers. We are working with them to make sure that the decisions they’re making are transparent and fair to the different communities involved.”
For more information
Co-authors of the study include Derek Ouyang, Research Manager of the Regulation, Evaluation, and Governance Lab (RegLab) at Stanford and Jeffrey Koseff , the William Alden Campbell and Martha Campbell Professor in the School of Engineering , Professor of Civil and Environmental Engineering in the School of Engineering and the Stanford Doerr School of Sustainability, and a Senior Fellow at the Woods Institute for the Environment . Koseff is also the Faculty Director for the Change Leadership for Sustainability Program and Professor of Oceans in the Stanford Doerr School of Sustainability.
This research was supported by Stanford’s Bill Lane Center for the American West. The work is the product of the Stanford Future Bay Initiative, a research-education-practice collaboration committed to co-production of actionable intelligence with San Francisco Bay Area communities to shape a more equitable, resilient and sustainable urban future.
Jenny Suckale, Stanford Doerr School of Sustainability: [email protected] Katy Serafin, University of Florida: [email protected] Josie Garthwaite, Stanford Doerr School of Sustainability: (650) 497-0947, [email protected]
Thank you for visiting nature.com. You are using a browser version with limited support for CSS. To obtain the best experience, we recommend you use a more up to date browser (or turn off compatibility mode in Internet Explorer). In the meantime, to ensure continued support, we are displaying the site without styles and JavaScript.
- View all journals
- My Account Login
- Explore content
- About the journal
- Publish with us
- Sign up for alerts
- Open access
- Published: 01 June 2023
GIS-based hydrodynamic modeling for urban flood mitigation in fast-growing regions: a case study of Erbil, Kurdistan Region of Iraq
- Andam Mustafa 1 ,
- Michał Szydłowski 1 ,
- Mozafar Veysipanah 2 &
- Hasan Mohammed Hameed 3
Scientific Reports volume 13 , Article number: 8935 ( 2023 ) Cite this article
4638 Accesses
3 Citations
1 Altmetric
Metrics details
- Civil engineering
- Climate-change mitigation
- Environmental impact
- Natural hazards
Floods threaten urban infrastructure, especially in residential neighborhoods and fast-growing regions. Flood hydrodynamic modeling helps identify flood-prone locations and improve mitigation plans' resilience. Urban floods pose special issues due to changing land cover and a lack of raw data. Using a GIS-based modeling interface, input files for the hydrodynamic model were developed. The physical basin's properties were identified using soil map data, Land Use Land Cover (LULC) maps, and a Digital Elevation Model (DEM). So, the HEC-RAS 2-D hydrodynamic model was developed to estimate flood susceptibility and vulnerability in Erbil, Iraq. The case study examines the quality of flood modeling results using different DEM precisions. Faced with the difficulty, this study examines two building representation techniques: Building Block (BB) and Building Resistance (BR). The work presented here reveals that it is possible to apply the BR technique within the HEC-RAS 2-D to create urban flood models for regions that have a lack of data or poor data quality. Indeed, the findings confirmed that the inundated areas or areas where water accumulated in past rainfall events in Erbil are the same as those identified in the numerical simulations. The study's results indicate that the Erbil city is susceptible to flood hazards, especially in areas with low-lying topography and substantial precipitation. The study's conclusions can be utilized to plan and develop flood control structures, since it identified flood-prone areas of the city.
Similar content being viewed by others
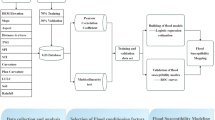
Flood sensitivity assessment of super cities
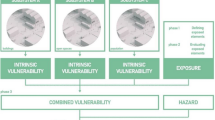
A GIS-based framework to assess heatwave vulnerability and impact scenarios in urban systems
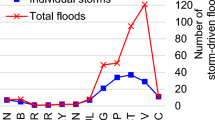
Current and projected flood exposure for Alaska coastal communities
Introduction.
Floods have developed into an event that people in developing countries regard as routine during the rainy season. Flash floods caused by climate change are one of the most common causes of fatalities and property damage in the twenty-first century. Recently, frequent urban flash floods have become the most popular topic among scientists, engineers, and local authorities in prone regions. Droughts and floods have historically been the most deadly natural disasters 1 . Aptly, the effects of flooding are expected to worsen as the world's population grows, the economy grows, and climate change 2 . Samanta, et al. 3 classified floods into three types: flash floods that last a few hours; floods that last several hours to several days; and floods that occur gradually over a relatively long period of time. Cities in general have become locations that are more prone to be flooded as a result of continued urbanization and population growth 4 . Therefore, even though these natural disasters were unavoidable, it is necessary to investigate flood hazards in order to better manage them going forward. Flood hazard assessment and modeling, in conjunction with inundation maps, can be of assistance to climate experts and scientists in this regard.
Building detailed flood inundation maps is typically done through the use of hydrodynamic models. A significant amount of data, time, and computational resources are required for the development of a hydrodynamic model for a large hydrological basin, all of which must be available at the same time 5 . In developing countries, obtaining archived data for rainfall-runoff modelling, high-resolution DEM for topography and accurate LULC for basin characteristics is a challenge. As Loudyi and Kantoush 6 pointed out, a serious challenge for flood risk assessment in the Middle East and North Africa (MENA) region is the unavailability and sometimes unreliability of data, particularly for the calibration and validation of flood models. There are some approaches that could be considered to fill such gaps, for example, as part of hydrological and hydrodynamic modeling of urban floods in Erbil, previously LULC from Landsat satellite images were prepared for the study area 7 . Furthermore, the availability of such data allows a more precise description of hydrological processes occurring in the real world, paving the way for a more numerical approximation of the processes involved in urban flooding. As a result of historical efforts to mitigate flood impacts, flood loss and damage in developed countries, particularly when measured in terms of the number of fatalities, is generally less severe than in developing countries 8 .
Researchers in many parts of the world have attempted to use geospatial techniques to model floods in urban and rural areas 9 , 10 , 11 , 12 , 13 , 14 , 15 , 16 for surface and ground water detection 17 , 18 , analyzing rainfall 19 , 20 , 21 , LULC analysis 22 , 23 , 24 , 25 , 26 , 27 , and finally flood risk mapping and analysis 28 , 29 , 30 , 31 , 32 . A model that utilizes artificial neural networks (ANN), geographic information systems (GIS), and remote sensing (RS) was developed by 33 , 34 to estimate ground water recharge mapping in the Iraqi Western Desert. Using a long-term perspective, a group of Polish researchers presented a method for analyzing the impact of urbanization on the frequency of flooding inside an urban catchment 35 . The integration of GIS remote sensing data and survey data with the ANN was used by 36 to generate a hydrological soil group map for Alghadaf Wadi in Iraq, providing timely, fast, and relatively cheap data for mapping and monitoring soil texture. Samela, et al. 14 developed a GIS tool that is able to delineate areas that are prone to flooding in an efficient and cost-effective manner. El-Saoud and Othman 37 estimated flash floods in Makkah's catchment region utilizing spatial parameter distribution and mathematical hydrological and hydrodynamic modelling. This study created a flash flood risk map. SZYDLOWSKI 38 presented flash flood propagation computations in natural and urban areas, shallow water equations are used to model free surface unsteady water flow. There are a variety of 2-D numerical models and software packages available, each with its own set of capabilities and developed by a variety of different developers; some must be purchased, while others are free and open-source 39 . Glenis, et al. 40 presented and validated City Catchment Analysis Tool – CityCAT, a novel software for modeling, analysis, and visualization of surface water flooding. It consists of a 2-D overland flow routing model that provides quick evaluation of combined pluvial and fluvial urban flood risk and the effects of various flood mitigation strategies. Macalalad, et al. 41 set up the Liuxihe model in order to develop a forecasting scheme and simulate the observed flood process. The study reproduced past flash flooding events and demonstrated a significant correlation between past dense storm events and their respective simulated river discharges. With the help of a 2-D rainfall-runoff simulation at the basin scale, Costabile, et al. 42 investigated the performance and capabilities of the HEC-RAS 2-D model. Mustafa and Szydłowski 43 demonstrated how different building representation techniques and hydrodynamic models can influence the results of urban flood simulations using the HEC-RAS 2-D package. Both BB and BR can be applied in HEC-RAS 2-D. Alipour, et al. 44 examined HEC-RAS 2-D's configuration factors and parameters. They investigated the effects of several model configuration factors, such as the floodplain and channel roughness coefficients, terrain and mesh size, and river boundary conditions, on the dynamics of water levels, maximum water level and flood extent.
Following the invasion of Iraq by the coalition forces in April 2003, Erbil, the capital of the Kurdistan Region of Iraq (KRI), became the focal point of development in the region and is now regarded as an important political, economic, and administrative center not only for Iraq but also for the neighboring countries 45 . KRI has taken steps to develop its oil and gas industry and attract investment from international oil companies since 2009, in order to increase its capacity and prepare for a gradual transition to independence 46 . Rapid urbanization is one of the main causes of flash floods in Erbil. As a result, the patterns of LULC in Erbil have changed significantly over the last two decades, particularly in the urban areas 7 . The most important factor that has a significant impact on creating the danger of flash flooding is the improper use of land for urbanization where the paths of the waterways have been closed. In the aftermath of this, the number of urban flash floods has also increased significantly 47 . Changes and developments to the land surface within cities will have an effect not only on the likelihood of increased flooding, but also on urban water management generally 48 . On the other hand, there have been studies that have linked the increasing frequency of floods to the impact of climate change 49 , 50 . For applications in urban settings, high temporal resolution of precipitation, such as sub-daily, is required for establishing a relationship between extreme precipitation Intensity–Duration–Frequency IDF 51 , 52 , 53 . When it comes to developing countries such as Iraq, the number and distribution of stations capable of recording rainfall on a shorter time scale are limited. The Indian Meteorological Department (IMD) equation was used to determine the rainfall data for periods shorter than 24 h, and IDF curves and empirical IDF formulas for the city of Erbil were developed as a result of these limitations 54 . To assess flood risk, scientists consider the likelihood and severity of a flood in a specific area at a specific time, along with the potential consequences 55 , 56 , 57 .
From 1950 to 2010, the number of people who died in Iraq as a result of flooding was only 11 1 . In the fourth quarter of 2021, Erbil was struck by flash floods on October 30 and December 17, the latter of which was particularly devastating, resulting in the deaths of 12 people and the disappearance of the body of a 10-month-old child for almost two months. Another flash flood hit Erbil on January 13, 2022, approximately one month after the previous one. Specifically, this one was located mainly within the study area that we modeled in HEC-RAS 2-D in this paper. Flash floods are more common in areas with a dry climate and impervious terrain because lack of pervious surface or vegetation allows torrential rains to flow overland rather than infiltrate into the ground. According to the available evidence, the city has experienced three floods in the span of just three months. According to a press release from the province of Erbil, more than 7000 people were declared to be in a state of special calamity 58 , and the total flood damage in Erbil was estimated to be $14,5 million 59 . The economic impact of recent flash floods has increased dramatically. Despite the devastation caused by Erbil's flash floods, the study area's summer fresh-water shortage makes flash floods a valuable source of water.
This paper addresses a critical issue of urban flash floods in Erbil and presents a new approach to model and identify flood-prone areas using HEC-RAS 2-D hydrodynamic model, where there has been no such work previously. The novelty of the work lies in discussing the challenges of accurately assessing and modeling flood hazards in this area, particularly regarding the availability and reliability of data. Previous studies on stormwater management in Erbil have been lacking, and there is a need to establish sustainable strategies to mitigate the adverse effects of flooding on the socio-economic fabric of society. The study highlights the importance of accurate topographic representation using a DEM of different precision to improve the flood modeling results. The impact of floods on society is also discussed, emphasizing the need for effective management strategies. This study is the first of its kind in the study area, and it is expected to provide valuable insights into developing sustainable urban stormwater management practices.
Materials and methods
Erbil (also known as Hawler in the Kurdish language) is the capital city of the Kurdistan Region in northern Iraq, and it is the region's largest city by population. Erbil, Iraq's second-largest city in the north of the country after Mosul, is the region's economic hub. In 2020, it was expected to have a population of 2,254,422 inhabitants and more than one million of them living in the city center. Erbil Province has a total land area of 14,873.68 km 2 2 , 60 . The investigation will be focused on the central district of Erbil. In the hydrodynamic modeling using HEC-RAS 2-D, the area that was considered was within the 120 m ring road (circular highway around the city), which is approximately 102.38 km 2 . Due to the fact that a DEM with an acceptable resolution is only available within this boundary, only this area is considered in the modeling as the study area in HEC-RAS 2-D (Fig. 1 ). In general, Erbil Province has a semi-arid continental climate, which is characterized by hot and dry summers and cold and wet winters, owing to its geographic location on the Arabian Plate. It is only possible to consider this climate for the city center because the mountainous region to the north and northeast of the province, which has a Mediterranean climate, cannot be considered. More detailed information can be found in 47 , 61 , 62 .
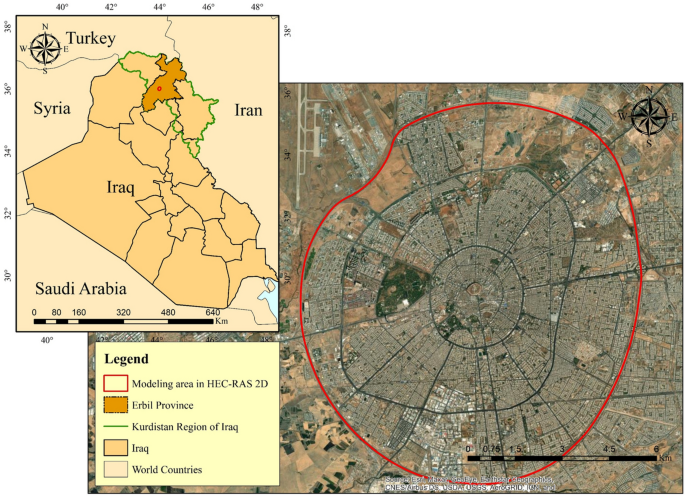
© ESRI, URL: https://www.esri.com/en-us/arcgis/products/index ).
Study area. Base imagery sources: Esri, DigitalGlobe, GeoEye, i-cubed, USDA FSA, USGS, AEX, Getmapping, Aerogrid, IGN, IGP, swisstopo, and the GIS User Community. These maps were processed and generated in ArcGIS 10.7 software (
Data availability and processing
In the development and simulation of hydrological and hydrodynamic models, the availability of raw data is the most important factor to be considered. It was necessary to acquire a DEM from the Shuttle Radar Topography Mission (SRTM) ( http://dwtkns.com/srtm30m/ ) with a resolution of one arc-second (30 m) in order to delineate the watershed boundary of the study area (Fig. 2 ). Li and Wong 63 conducted an analysis to determine how various DEM data sources may influence the outcomes of hydrologic applications. A DEM with a resolution of 10 m was created using the point clouds of the LIDAR images (the data source was the Erbil municipality, the work date back to 2010). Then, a Feature Manipulation Engine (FME) was used to generate another DEM over the study area, from the layout map of the city, the heights of the buildings were extracted to create a building layer in three dimensions (3D). From the buildings, a raster with spatial resolution of 1 m was created. After resampling the DEM of the city (only the area inside 120 m ring road) from 10 to 1 m, it was then overlaid on the raster accompanied by the buildings. Raster calculation with maximum operation was applied to create a DEM of the city with a resolution of 1 m (Fig. 3 ).
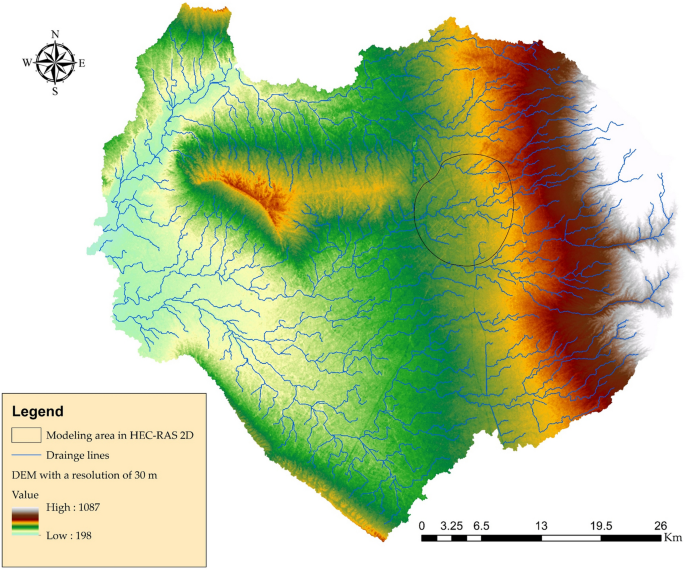
Delineation of watershed using a Digital Elevation Model (DEM) with a resolution of 30 m. This map is processed and generated in ArcGIS 10.7 software (
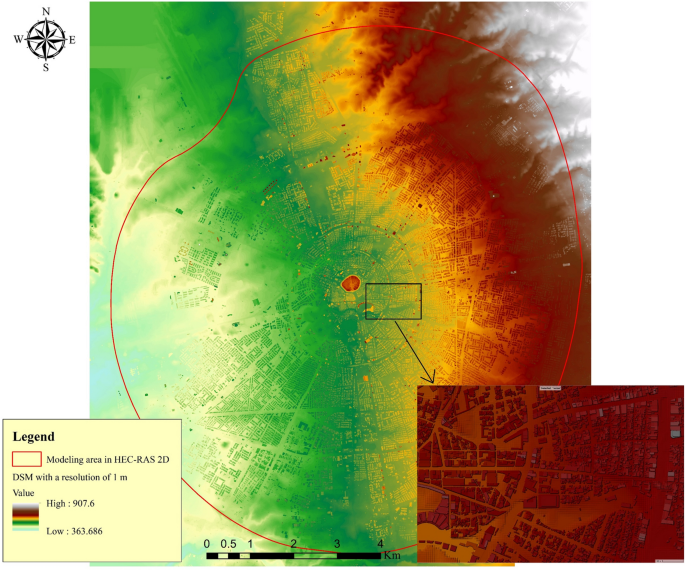
Digital Elevation Model (DEM) with 1 m resolution for modeling area (BB technique simulations) in HEC-RAS 2-D. This map is processed and generated in ArcGIS 10.7 software (
The Food and Agriculture Organization of the United Nations (FAO) prepared a digital soil map of Iraq, from which a soil map of the studied area was extracted. Brown soils with deep phases have dominated the soil profile in the area under consideration for 2-D hydrodynamic modeling (Fig. 4 ). The Natural Resource Conservation Service (NRCS) divided soils into four Hydrologic Soil Groups (HSG) based on their runoff potential 64 . A, B, C, and D are the four HSGs, where A has the least potential for runoff and D have the most. The study area for modelling in HEC-RAS 2-D is in group C 62 .
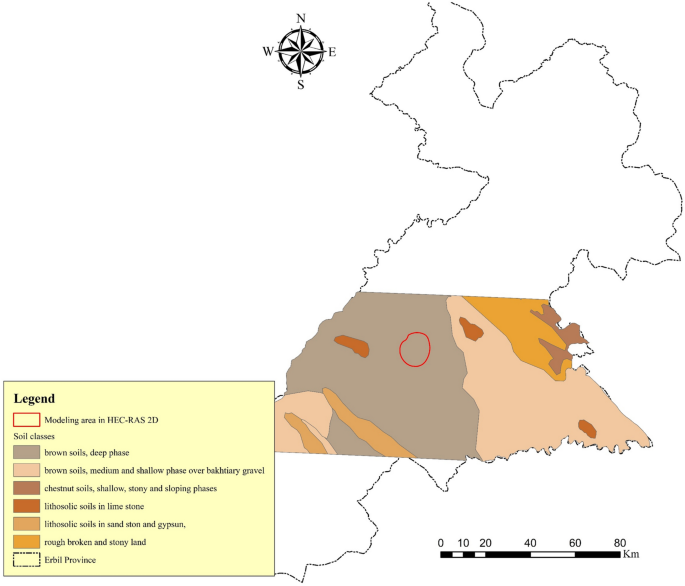
The soil classes in the study area. This map is processed and generated in ArcGIS 10.7 software (
The LULC map was created using data collected through remote sensing. When cloudy coverage is less than 5%, a satellite image from Sentinel-2B (2017–present) was downloaded from the Copernicus open access hub platform ( https://scihub.copernicus.eu/ ), which is operated by the European Space Agency. The image was captured by a satellite on September 13, 2021. Regarding the LULC classification, the study area was divided into three categories: built-up (which included residential, industrial, commercial, local streets, roads, and other urban areas); bare land (which included uncovered soils, unused areas, and dry river beds); and vegetation (including forests, orchards, vegetable fields, parks, lawns, shrubs, and others) (Fig. 5 ). Approximately 66.36 km 2 (64.82%) of the total study area is covered by built-up areas, while 25.038 km 2 (24.45%) and 10.97 km 2 (10.71%) respectively are covered by bare land and vegetated areas.
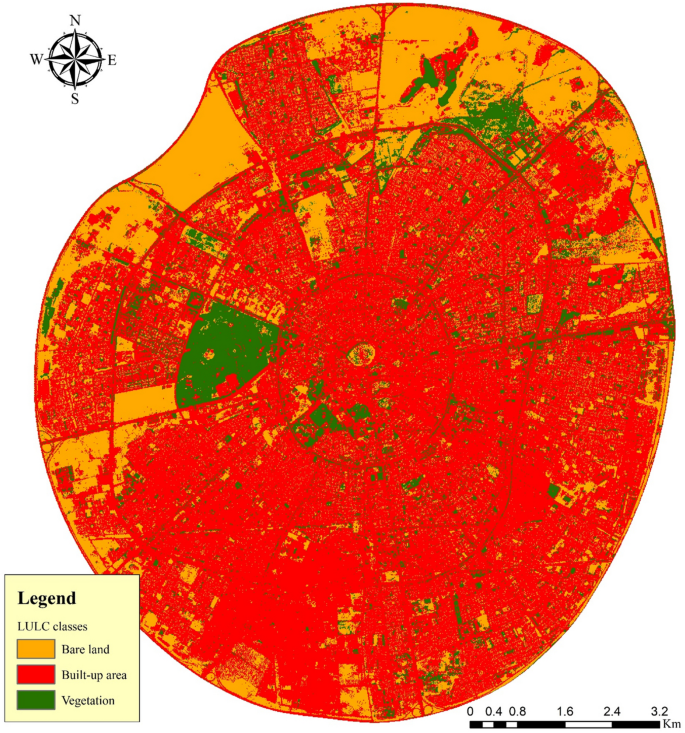
Land use land cover classes inside the modeling area in HEC-RAS 2-D. This map is processed and generated in ArcGIS 10.7 software (
HEC-RAS 2-D model setup
Water flow through natural rivers and other channels is modelled using HEC-RAS 6.1, an open-source software developed by the U.S. Army Corps of Engineers (USACE) to simulate hydrodynamics of water flow. One-dimensional 1-D steady and unsteady flow modeling, as well as two-dimensional (2-D) unsteady flow modeling, as well as combined one-dimensional and two-dimensional 1-D/2-D unsteady flow routing, sediment transport/mobile bed computations, and water temperature/water quality modeling are all possible with this software 65 , 66 . The Digital Terrain Model (DTM) is responsible for inducing the majority of the topographic characteristics of the study into the HEC RAS 2-D model. Using a DEM as an input file, HEC RAS 2-D is capable of creating a DTM within the program. Specifically, a DEM plus buildings with a resolution of 1 m was prepared for use in the generation of the study area's DTM in this case. In the geometric data editor of the software, a 2-D flow area describing the boundary of the assumed flood domain is created by drawing a polygon with the orthophoto as the background layer. Afterwards, an automatically generated computational mesh within the boundary layer is created with a 10 × 10 m cell size. This results in a total of 1,022,897 grid cells with an average size of 100 m 2 . To demonstrate the effects of computational cell size on the model outputs and model run time, two more mesh configurations with 8 × 8 and 20 × 20 m are examined. The total number of grid cells in these mesh configurations is 1,598,552 (average cell size = 64 m 2 ), and 255,501 (average cell size = 400 m 2 ), respectively. The four building representation techniques that are commonly used to model built-up area flooding in hydrodynamic numerical models are the BB, BR, Building Hole technique (BH), and Building Porosity technique (BP) 67 , 68 , 69 , 70 , 71 . In the HEC-RAS 2-D, both BB and BR are applicable. BB technique means increasing the ground elevation of building units by modifying distributed ground elevation data, by configuring the buildings to a real height or a sufficiently high artificial elevation value to ensure no water flows over the buildings. Here, the entire simulated flow area should be meshed as a unified grid, so water flows around buildings. In BR, the modeler may give each grid a different Manning coefficient. High Manning coefficients result in low water flow velocity. In this method, the simulated building areas are given a high Manning n value to increase friction. In other simulated areas, a low value represents the real land cover. Due to the high friction coefficient assigned to the building units, water flows slowly over them but behaves as if there is a high resistance against flow. A detailed description can be found in 43 .
The model's other parameters, which include 2-D surface roughness, curve number for LULC classes, boundary conditions, and rainfall data, are also required. Table 1 categorizes the LULC classes, Erbil City center is a densely urbanized area with predominant brown soil and deep phase. Thus, based on imperviousness and soil type, three different Manning's n are selected for the 2-D area 72 ; see Table 2 . Defining boundary conditions within the HEC RAS 2-D flow area is required in order to run the 2-D model simulations. Boundary conditions for defined 2-D flow areas can include a variety of features such as flow and stage hydrographs, normal depths, rating curves and precipitation boundary conditions, among other things. In the current study, only 23 outlets are defined as boundary conditions with normal depth. A time series of rainfall is used as meteorological data in the unsteady flow section for each storm event, and a simulation run is carried out to generate flood inundation for each storm event.
Analyzed long-term series of maximum daily rainfall data were used in this study and within them, a theoretical probability distribution of 10, 5, and 1%, which is equal to 71.16, 83.21, and 113.49 mm 47 (see Fig. 6 ). The method that was used to prepare the temporal rainfall distribution was based on Huff's second quartile 74 . The reasons for using this method are explained in 47 . Uniform rainfall distribution in space is considered. Unsteady flow routing can be done two-dimensionally with either the Shallow Water Equations (SWE) or the Diffusion Wave equations (DWE). To solve the flow over the computational mesh using HEC-RAS, three sets of equations are available: the Diffusion Wave equations; the Shallow Water Equations (SWE-ELM, or Shallow Water Equations, Eulerian–Lagrangian Method) original equations; and a more momentum-conserving Shallow Water Equations solution (SWE-EM, which stands for Shallow Water Equations, Eulerian Method) 66 . In the present study, the original (SWE-ELM) was considered for HEC-RAS 2-D. The flowchart shown in Fig. 7 illustrates the process of developing a HEC-RAS 2-D model.
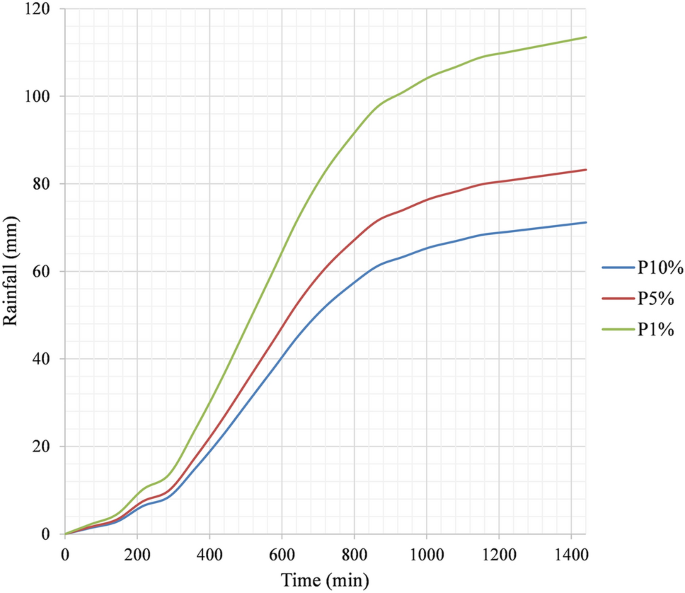
Temporal distribution of rainfall for three probability distributions of 10, 5, and 1%.
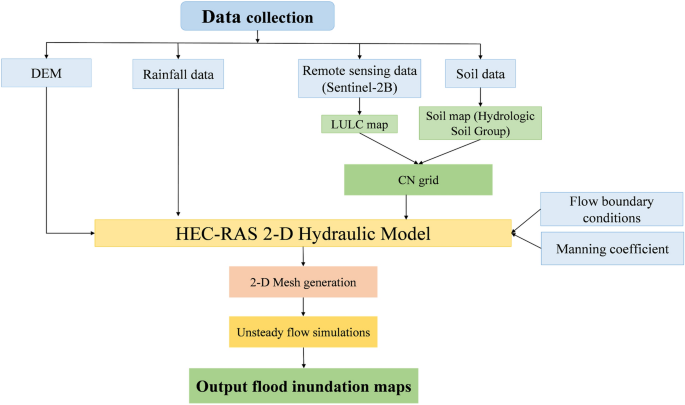
A flowchart illustrating the steps involved in developing a HEC-RAS 2-D model.
Results and discussion
Analysis of different mesh resolutions and building representation techniques.
This study compared two different building representation techniques as well as the outputs of various simulations using fine and coarse grid sizes in order to analyze the results. For modelling floods in urban areas, a fine 2-D grid, typically less than 5 m, is required to accurately simulate the flow on streets, intersections, around houses and buildings 75 . In the beginning, we focused on the BB technique to determine whether or not the prepared DEM was suitable for such numerical simulation. Because we believe that the quality of the DEM that was prepared is at an acceptable level. In this case, we were looking for the answer to the question of whether or not this model would provide us with results that were satisfactory. In our study, we used three different grid sizes, starting at 8 m and the others at 10 and 20 m. In fact, because of the size of the area considered in the modeling in HEC-RAS 2-D, it was difficult to simulate the flow using a grid size smaller than 8 m. The fact should be noted, however, that shrinking the size of hydrodynamic grid cells results in denser mesh and therefore requires a longer run-time to complete 76 , 77 , 78 , necessitating a trade-off between simulation accuracy and calculation time. In a study by 79 , two novel methodologies for reducing the processing time of 2-D large-scale flood simulations are compared in order to evaluate their merits and drawbacks and provide advice for their effective application. Specifically, Yalcin 80 stated that mesh resolutions created at the basin scale with a size greater than 10 × 10 m, referred to as a "coarse grid," cause significant errors in the estimated inundation extent because they are unable to capture rapid changes in the terrain geometry.
To shed light on the role of grid size in numerical simulation, multiple simulations have been done. When the BB technique was simulated using cell sizes of 8, 10, and 20 m, the results of the simulations revealed an error in the water depth calculation. In front of the buildings, a large amount of water has accumulated. We believe that this error is due to the disparity between the dimensions of the street and the size of the cell (See Fig. 8 a, b, and c). When more precise cell sizes are utilized, it is anticipated that the error will decrease. Our attention was drawn to something, water propagation and water depth in front of buildings can be improved if the grid size is reduced. The DEM and mesh resolutions are the most significant elements to consider when running simulations of water level dynamics using the BB technique. On the other hand, the floodplain roughness coefficient is a crucial consideration for mapping the extent of floodplain. According to Alipour, et al. 44 , the resolution of the DEM is the most essential factor across all scales for the simulation of the dynamics of water levels. In their research on the flood inundation simulation of the Mahanadi River in Odisha, Surwase, et al. 81 found that the HEC-RAS model is sensitive to the manning roughness coefficient. Figures 8 a, b, and c show a random location within the 2-D geometry area to demonstrate flow propagation. Figure 9 illustrates the levels to which the inundation depth is sensitive to the grid size used in the BB technique simulations. Because these simulations give a false impression of the extent and depth of the flood, they should be ignored in most cases. Because of the capabilities of the computer processor, it was not possible to reduce the cell size to less than 8 m. A mesh of this size would be unable to accurately simulate the abrupt change in the terrain. As a result, we came to the conclusion that the size of the 2-D flow area needed to be reduced in order for us to be able to model the area using a grid size of 2 × 2 m or even less than 2 m.
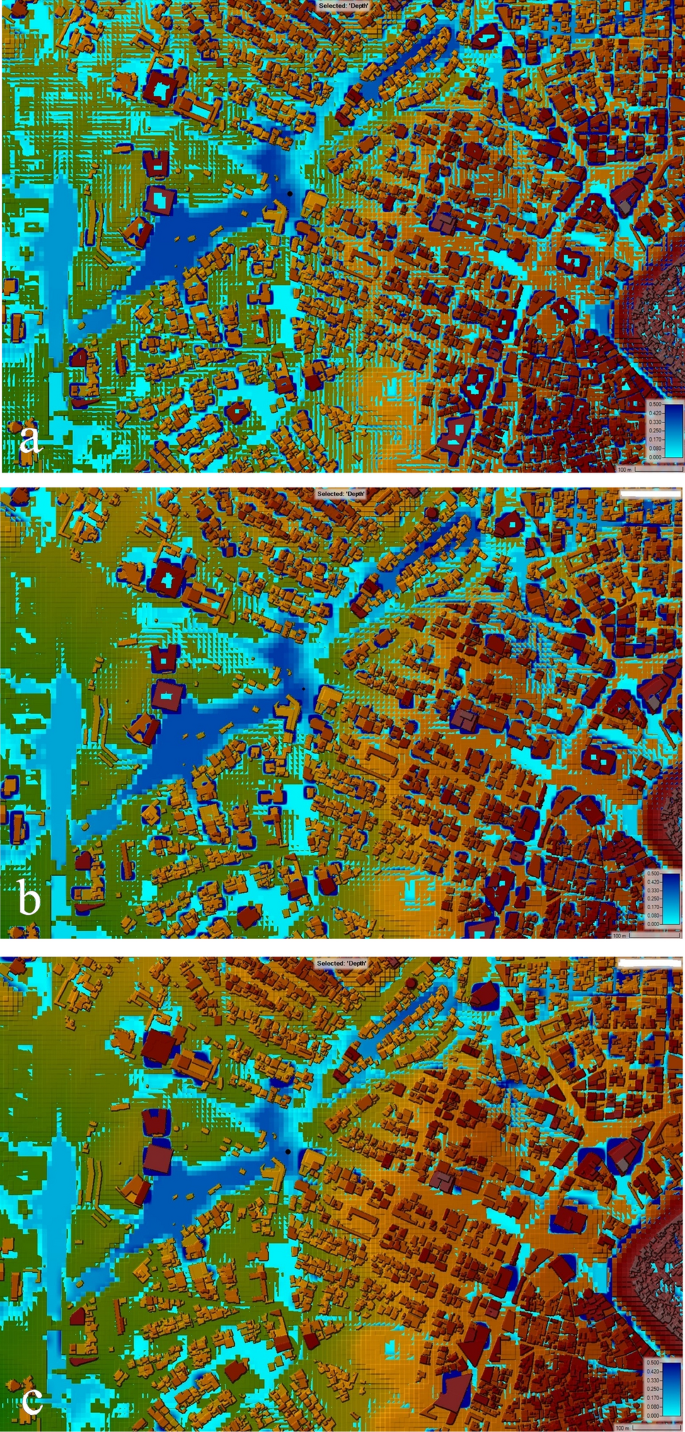
Analysis of the numerical simulation using different mesh resolutions with the BB technique: ( a ) Grid size 8 × 8 m. ( b ) Grid size 10 × 10 m. c Grid size 20 × 20 m. These maps were processed and generated in ArcGIS 10.7 software (
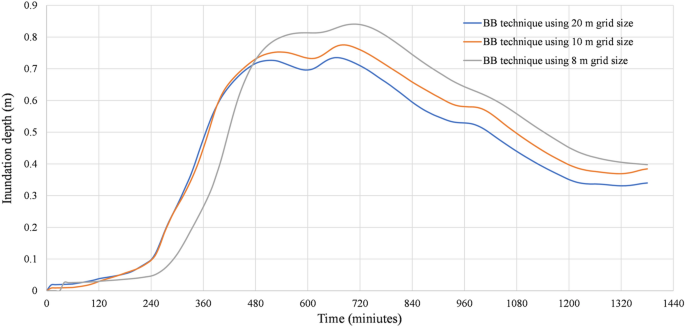
Comparison of inundation depth between BB technique simulations.
This is not the end of the story because it is the responsibility of the researchers to investigate all possible avenues so that they can eventually present a conclusion to society. Because we were interested in determining the effect of urban flooding inside of the 120 m ring road in Erbil, we made the decision to model this zone using a different method, which is known as the BR technique. Previously, Mustafa and Szydłowski 43 have validated this method by applying it on a small scale to a physical model of the Toce river. The Manning coefficient is a coefficient describing the roughness or friction of a surface in the field of flow, that estimates the average flow velocity. In the BR technique, Manning coefficient values (such as 10 m −1/3 s) are assigned to all the mesh regions that represent the building blocks (which here are known as user-defined polygons) to examine the resistance against the flow. When compared to the BB techniques, the results demonstrated a more accurate estimation of the water depth within the same grid size and geometry area. In point of fact, the BR method allows for the possibility of water entering the houses and buildings, whereas the BB method ensures that this will not occur because the building is modelled in the form of a block. In terms of the calculation of water depth, BR may give better results, which is something we could say in response to the question of which method is more realistic. If a high-resolution DEM is used in the modeling and simulation process, it is expected that the BB technique will give more accurate results about the flood extent and the most vulnerable areas.
Assessments of the areas affected by flooding
We came to the realization that BR is an effective technique for conducting analysis on floods in the study area, obviously, we reached this conclusion due to the lack of reliable data. Hence, the analyses presented are based on the BR technique with a 10 m grid size. Moving on, it is clear that we made some assumptions in order to develop the model for the area considered in HEC-RAS 2-D, one of which was that there is no inflow to the area at all, which is not true because there is inflow to the 2-D area through main roads and some underground box culverts that cross the 120 m ring road. However, because there is no recorded data regarding the flow, we made the decision to keep the boundary closed. Despite this, we are aware that this assumption has an impact on the results; however, by constructing some retention basins, we can prevent flow from the outside of the studied area into the inside. Beyond assumptions, we can confidently state that our modeling is accurate to a significant degree because when comparing our results with those areas known as prone areas, the accumulation of water in the HEC-RAS 2-D is calculated.
Using two orthogonal components of the flow, 2-D surface flow modeling attempts to model the propagation of overland flow. It is possible to predict the maximum inundation area and the flow dynamics, such as water depth and velocity, using 2-D modeling of the surface flow 82 . When we look at the extent of the flood, it is immediately apparent that water has accumulated in low-lying areas. Flood accumulation areas are often identified by their low elevation, which is a good indicator of their risk of flooding 28 . Furthermore, despite changes in the city's layout, the flow movement continues to follow the same path that it did a hundred or even a 1000 years ago. For example, the source of accumulated water in both Tayrawa street, particularly Tayrawa Bazar, and the main gate of the council of ministers on 30 m street is coming from the northeastern areas of the studied areas, such as the North industrial area and the area near the Family Mall shopping center, according to the findings (Fig. 10 a). The source of the flow that causes problems in Setaqan quarter on 60 m street, then in Saydawa quarter near the old Saydawa bridge, and near Nishtiman Bazar, before finally passing through the city via an underground box culvert, is located in the eastern part of the study area, and it comes from the Havalan quarter (Fig. 10 b). When the volume of flow exceeds the capacity of the box culvert, overflows occur in this zone. This event occurs after heavy rainfalls for a variety of reasons, including the flow from the surrounding area being accumulated over there, the area serving as a flow route, the stormwater system at this location appearing to be inadequate, old and finally, the accumulation of debris inside inlets, manholes, and box culverts, which prevents the system from functioning properly.
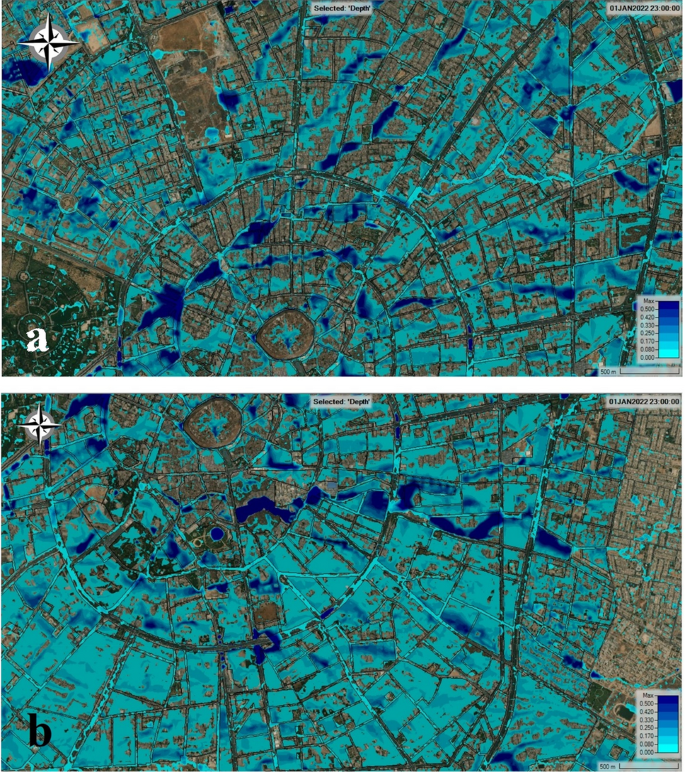
Results of the numerical simulation using BR technique with grid size 10 × 10 m: ( a ) upper part of the studied area. ( b ) the lower part of the studied area. Base imagery sources: Esri, DigitalGlobe, GeoEye, i-cubed, USDA FSA, USGS, AEX, Getmapping, Aerogrid, IGN, IGP, swisstopo, and the GIS User Community. These maps were processed and generated in HEC-RAS 6.1 software ( https://www.hec.usace.army.mil/software/default.aspx ).
The inundation depth (m) maps that were generated over the course of the simulation run period are used as criteria to describe the extent of the inundated areas. Although the area of flood inundation and its depth are the most critical parameters, particularly when mapping flood hazards 83 . We chose to focus on the extent and prone areas of flooding rather than the depth of inundation because the surface flow is all that is calculated during simulations. For the reason that some of the surface runoff will pass through the existing stormwater system. In addition, we performed three separate simulations based on a theoretical probability distribution of 10, 5, and 1%, which is equivalent to 71.16, 83.21, and 113.49 mm, respectively (the duration of the rainfall event was 24 h).
Here, in order to delineate flood extent in the studied area, we generated a vector layer using water depth maps at a value of 0.2 m. This means that the program will develop a vector layer for all areas where the water depth is equal to and/or greater than 0.2 m. This was done so that we could determine the extent of the flooding. After that, the vector layer was saved by being transformed into a shapefile. The vector layer was then sorted using the attribute tables, and areas smaller than 500 m 2 were ignored. As can be seen in Fig. 11 a and b, the amount of rainfall that occurs will cause the flooded area to expand, and this expansion will be proportional to the amount of rainfall that occurs. When the simulation was performed with a rainfall probability of 10%, an area of about 5.725 km 2 was affected by flooding. The size of this affected area increased by about 21.83%, which is equivalent to 1.25 km 2 , when the rainfall probability was increased to 5%. In addition to this, when we simulated this time with a rainfall probability of 1%, it resulted in a 71.37% increase in the magnitude of the flood extent (Fig. 12 ). In point of fact, the areas of the studied site that experience water levels that are higher than 0.2 m in the case of P 1% rainfall have an area that is approximately equivalent to 10% (9.811 km 2 ) of the total area (102.38 km 2 ). It is concerning that the majority of the areas that flood or accumulate water as a result of rainfall are public streets, commercial districts, and residential areas. As a direct consequence of this, both citizens and businesses experience a loss of life as well as property. It is a common observation in Erbil that rain that falls in a concentrated burst over a short period of time causes the water level in the streets to rise, which in turn has a significant effect on the flow of traffic. Other flood hazard parameters, such as velocity, could not be developed by us because of the quality of the data that was available and the level of uncertainty that we produced during the modelling process. It was mentioned that only 10.71% of the studied area is covered by vegetation, and the majority of this vegetation can be found in two large areas in the middle of the city. Because the permeable areas were not distributed well, there was less infiltration and more runoff as a result.
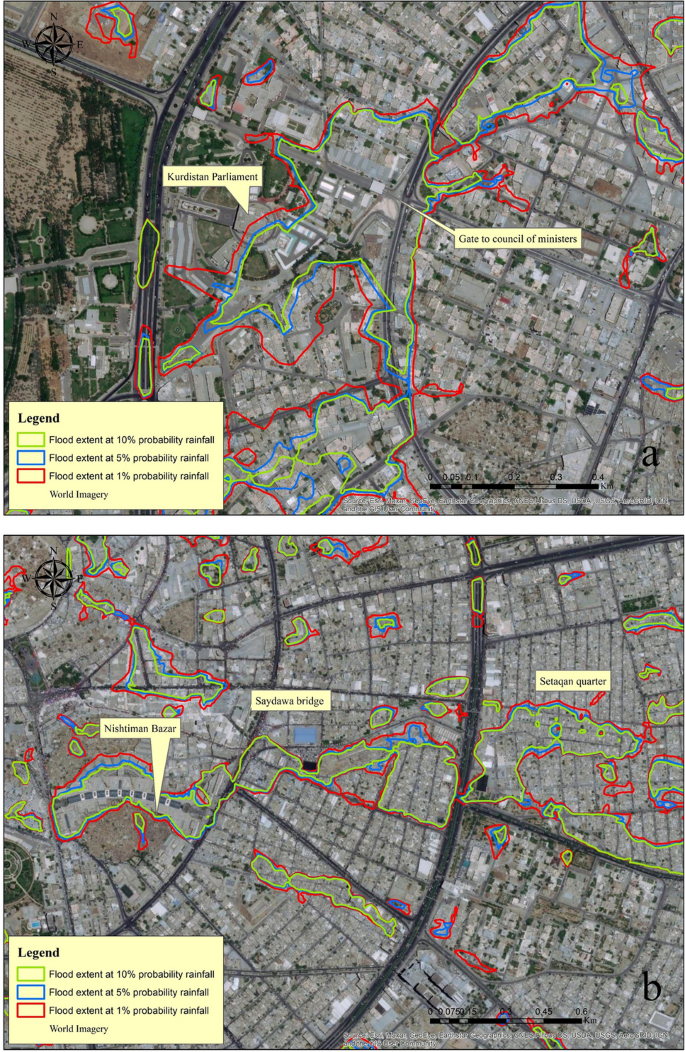
Comparison of the development of flooded areas based on a different rainfall simulation: ( a ) Gate to council of ministers. ( b ) Nishtman Bazar, Saydawa quarter and Setaqan quarter. Base imagery sources: Esri, DigitalGlobe, GeoEye, i-cubed, USDA FSA, USGS, AEX, Getmapping, Aerogrid, IGN, IGP, swisstopo, and the GIS User Community. These maps were processed and generated in ArcGIS 10.7 software (
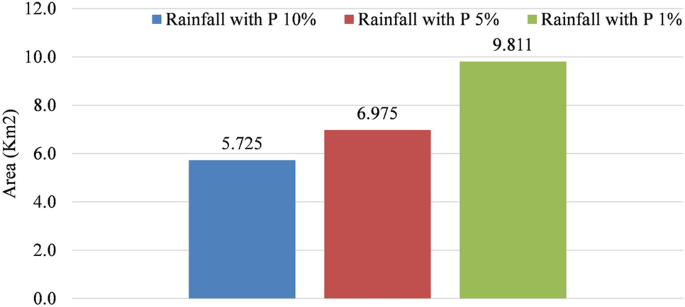
The evolution of inundated areas.
Observing two extraordinary events that occurred at the end of 2021, authorities in Erbil revealed that the flooded area is located on the route of natural and seasonal streams, which occurred outside the area considered in the HEC-RAS 2-D modeling in the current study. The expansion of the city has resulted in the construction of numerous residential and commercial buildings, as well as industrial and manufacturing facilities, along the path of natural flow routes. In this section, we evaluated the areas that were at risk of flooding outside of the 120 m ring road by using the DEM with a resolution of 30 m to assess flood basins that had been delineated. The route of the natural streams can clearly be seen in Fig. 13 , which shows that both Zerin city and Korean village are located along the route of the natural streams. According to a media statement issued by the governor of Erbil on October 30, 2021, an amount of 55 mm of rainfall was recorded in the upper part of the city in just two hours on that day. In the northeast, where there is higher topography known as the Tarin heights, crazy flow can flow to the prone areas.
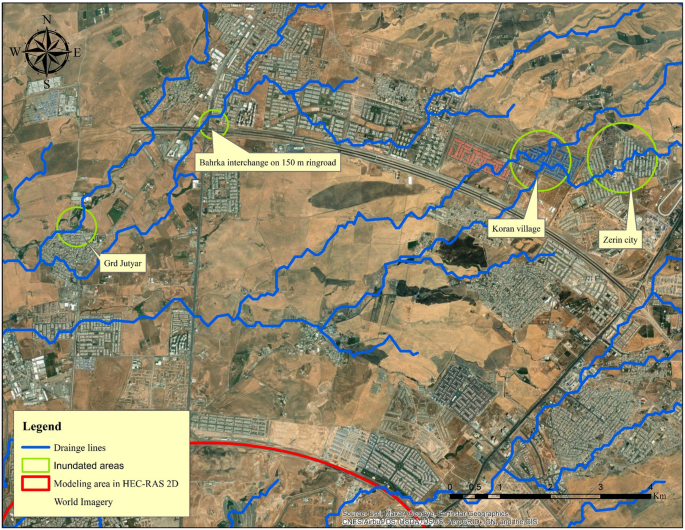
Some inundated areas in Erbil during the storm event of October 30 and December 17, 2021. Base imagery sources: Esri, DigitalGlobe, GeoEye, i-cubed, USDA FSA, USGS, AEX, Getmapping, Aerogrid, IGN, IGP, swisstopo, and the GIS User Community. This map is processed and generated in ArcGIS 10.7 software (
The same can be said for the village of Grd Jutiar, which is located directly adjacent to the natural canal. In fact, on that particular day, the canal was unable to handle the volume of water flowing through it. As a result of this, the overflow of canal banks occurred somewhere near the north side of the village, resulting in the catastrophic event. The first phase of a 150 m ring road connecting the main Shaqlawa road with the main Bahrka road was completed in 2021. In an interview with Rudaw TV 84 , the project manager for the 150 m ring road stated that the culverts and box culverts that cross the ring road and run along the ring road were only intended to handle stormwater from the surrounding areas and household waters, not the large amounts of water that flow from the natural basin. Erbil city has expanded toward mainly the Northeast and East areas, but detailed hydrologic studies are absent. Many stormwater and sewer pipes in local areas throughout Erbil have been built without taking the dimensions of water pathways and streams into consideration. It is now possible to experience flash flooding in areas where urban and infrastructure development has taken place.
Flooding in Erbil ranges from minor incidents, such as inundation of streets and then water entering some houses, to major incidents, such as large areas of the city being submerged for several hours at a time. The event, which took place on December 17, 2021, was primarily focused on the Southeast of the city, specifically the Bnaslawa sub-district and the Roshinbiri quarter (Fig. 14 ). In reality, this was a catastrophic event that was unprecedented in Erbil's history. Because 12 people died, one of them was a 10-month-old child whose body was found approximately 60 days after the event and more than 15 km away from his house. A large number of small-scale local problems are common in most developing cities around the world, primarily because their stormwater systems do not have enough capacity to handle heavy rainstorms 85 . Greater flooding risk exists in areas that are close to the main channel and accumulation path 86 . Poorly designed highways that do not take into account natural drainage patterns can lead to increased runoff and flooding during heavy rainfall events 87 , 88 . There were various contributing factors to this catastrophic event, the first of which was a reduction in the cross-section of streams, with their bed elevations approaching the elevation of the street in some places. The reason for this is that these seasonal streams have not been cleaned in a long time and have become clogged with river sediments and debris. In addition, a large number of quarries are operating on the river bed on the upstream side of the developed area (Bnaslawa sub-district) to extract sub-base or other materials. According to the locals' speech, those quarries created a dam-like barrier, which collected a large volume of water on that particular day before being breached, causing the flow to become increasingly erratic. As we can clearly see, a large number of residential areas have been constructed directly adjacent to stream routes, indicating that this type of event is predictable.
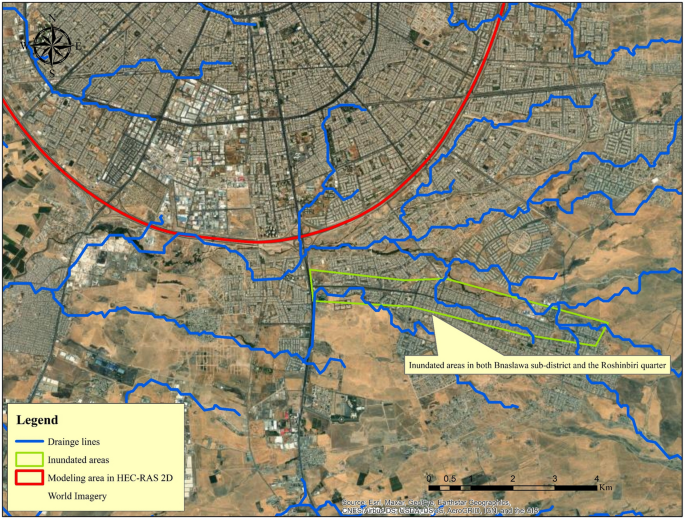
Bnaslawa sub-district and the Roshinbiri quarter were inundated in Erbil during the storm event of December 17, 2021. Base imagery sources: Esri, DigitalGlobe, GeoEye, i-cubed, USDA FSA, USGS, AEX, Getmapping, Aerogrid, IGN, IGP, swisstopo, and the GIS User Community. This map is processed and generated in ArcGIS 10.7 software (
The floods in Erbil cause broad damage on several levels (Fig. 15 ). On a human level, floods claim a number of lives. Economically, urban areas are the most frequently affected, and this is especially true in the most severe events. Environmental consequences are big, and we are seeing deterioration of the natural environment, as well as the production of debris and wreckage, which is being spread over drainage lines and residential areas. There are several different approaches that can be taken to mitigate the consequences of flooding and heavy rainfall. One of the strategies is to make individuals aware of locations that are at risk of flooding and to warn them accordingly. For example in Brazil, a Decision Support System (DSS) coupled with a flood alert web application was developed and evaluated in the Prosa Basin (Midwestern Brazil) to send early flood warning messages to ordinary people 89 . The basis of the flood alarm system was the Hydrologic Modeling System (HEC-HMS) and River Analysis System (HEC-RAS) of the Hydrologic Engineering Center.
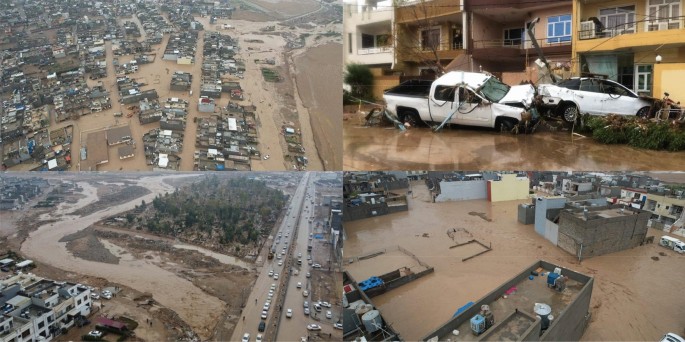
Flooding had devastating consequences in Erbil in two separate events at the end of 2021.
Recommendations, suggestions and the future scope
In accordance with the findings of this study, the recommendations made priorities the implementation of a plan for flood hazard prevention and the protection of the city and its infrastructure through the maintenance of existing floodwater drainage facilities. The result of this study highlights the necessity of establishing hydrodynamic facilities for the control, obstruction, and divergence of the floods of drainage lines, and the specific recommendations are as follows:
The adoption of a plan to harvest water in the northeast and east of the city would make a significant contribution to the provision of water for people and farms. It would also aid in the process of afforestation, which slows down floods and allows them to feed the underground reservoirs. At the same time, it would reduce a portion of the wasting floods that are directed towards the city center. Moreover, in order to reduce the craziness of the flow and delay the flow into the city.
Cleaning and maintaining the width of seasonal streams and drainage lines, which are located in various parts of the city, especially those located in the east, the northeast and the southeast. Also necessary is the refinement of the slope of the drainage channel in the area in order to increase the drainage velocity.
A reserve for each stream must be established to prevent construction in drainage lines and flood catchments. This reserve must remain in place even after the proposed man-made channels have been constructed. A mandatory study of flood risks, including their peaks and catchments, must also be provided with each project and submitted to the appropriate authorities for consideration of approving the project.
The stormwater management system should be changed/modified so that it can keep up with urban development, population growth, and climate change. Because there was no way to show that they were the same.
Green roofs, rainwater harvesting systems in the household, permeable surfaces, swales, channels and rills, infiltration trenches, detention and infiltration basins, rain gardens, and retention ponds are all examples of Nature-Based Solutions (NBS) that can be used to improve the capacity of stormwater systems. Certainly, this will be after evaluating the outcomes of each solution using high-resolution models and selecting the most suitable option for the research area.
The uneven distribution of the city's greenery has contributed to the problem of increased runoff. One way to lessen the impact of this is to reorganize the green spaces within the city layout. In this regard, precautions should be taken in areas that are prone to flooding.
Local and regional authorities should develop flood control and protection legislation and regulations in order to save lives and property from the effects of flooding. Having such types of national laws in place is extremely important for managing flood risk and increasing future flood resilience in floodplains.
The Storm Water Management Model (SWMM) or a coupled model can also be used to evaluate the efficiency of the current urban drainage system in Erbil city center, which will allow for a more accurate analysis of urban flooding. However, because there are currently no GIS-based stormwater lines in Erbil, a significant amount of effort may be required. The model can simulate storm events and the resulting flooding scenarios and shall help the authorities in the directorate of water and sewer/Erbil devise an efficient drainage system in the city. Filling in the gaps in the current study will result in a completely different understanding of the Erbil flooding process in the future, which will aid the authorities in developing a more comprehensive flood management and mitigation plan.
Conclusions
Climate change, which causes and amplifies large-scale natural disasters, poses a threat to the city of Erbil. These dangers can sometimes have a negative impact on people's daily lives and activities in the city. Our previous paper clearly illustrates the magnitude of the flooding phenomenon that has occurred in this area. Flash floods in urban areas have been and will continue to be a major problem in many cities throughout the world, particularly in developing countries. The threat of flooding can be countered by the opportunity it provides to bring in more water. As a consequence of this, flood management is a component of water management, and it should be given particular consideration in the Kurdistan Region of Iraq, particularly in the city center of Erbil, where solutions to the problem of water scarcity are required as quickly as possible. Due to the rapid growth of Erbil as a city in a developing country without the necessary funds to expand and renovate their existing drainage systems, the situation continues to deteriorate further. The findings of this study tell us that the effects of flash flooding in Erbil in the areas shown are due to their location that is located on the waterways, and even the areas that were flooded in the first month of 2022 in the city center were waterways in the past. Therefore, we believe that serious and practical steps must be taken in order to lessen the effects of floods, which will allow for runoff to be collected, utilized, and stored in order to increase groundwater levels. Currently, certain areas of the city are experiencing water shortages during the warm summer months, and the level of groundwater has decreased by a sizeable level.
In order to shed some light on the crucial role that data plays in the preparation of hydrological and hydrodynamic models, we must keep in mind that obtaining data in developing countries is not an easy task. There are difficulties and challenges to overcome at each stage. Even if the goal is to develop a simple flood model, we face obstacles and challenges. During the course of this work, we tested a number of different approaches to prepare the hydrodynamic model. In the end, we were successful in employing the BR technique to construct a model with cell size (10 * 10). In point of fact, we were able to draw the conclusion, on the basis of our observations, that the BR technique is adequate for modelling flash floods in regions where there is a scarcity of data.
Data availability
The datasets generated during and/or analysed during the current study are available from the corresponding author on reasonable request.
Ritchie, H. & Roser, M. Natural disasters. Our World in Data (2014).
Tanoue, M., Hirabayashi, Y. & Ikeuchi, H. Global-scale river flood vulnerability in the last 50 years. Sci. Rep. 6 , 36021. https://doi.org/10.1038/srep36021 (2016).
Article ADS CAS PubMed PubMed Central Google Scholar
Samanta, S., Koloa, C., Kumar Pal, D. & Palsamanta, B. Flood risk analysis in lower part of Markham river based on multi-criteria decision approach (MCDA). Hydrology 3 , 29. https://doi.org/10.3390/hydrology3030029 (2016).
Article Google Scholar
Cao, W. et al. Increasing global urban exposure to flooding: An analysis of long-term annual dynamics. Sci. Total Environ. 817 , 153012. https://doi.org/10.1016/j.scitotenv.2022.153012 (2022).
Article ADS CAS PubMed Google Scholar
Galasso, C. & Senarath, S. U. S. In Vulnerability, uncertainty, and risk 1415–1424 (2014).
Loudyi, D. & Kantoush, S. A. Flood risk management in the middle east and north Africa (MENA) region. Urban Water J. 17 , 379–380. https://doi.org/10.1080/1573062X.2020.1777754 (2020).
Mustafa, A. & Szydłowski, M. The impact of spatiotemporal changes in land development (1984–2019) on the increase in the runoff coefficient in Erbil Kurdistan Region of Iraq. Remote Sens. 12 , 1302. https://doi.org/10.3390/rs12081302 (2020).
Article ADS Google Scholar
Doocy, S., Daniels, A., Murray, S. & Kirsch, T. D. The human impact of floods: A historical review of events 1980–2009 and systematic literature review. PLoS Curr. https://doi.org/10.1371/currents.dis.f4deb457904936b07c09daa98ee8171a (2013).
Article PubMed PubMed Central Google Scholar
Abdelkarim, A., Gaber, A. F. D., Youssef, A. M. & Pradhan, B. Flood hazard assessment of the urban area of Tabuk City, Kingdom of Saudi Arabia by integrating spatial-based hydrologic and hydrodynamic modeling. Sensors 19 , 1024. https://doi.org/10.3390/s19051024 (2019).
Article ADS PubMed PubMed Central Google Scholar
Bouaida, J., Witam, O., Ibnoussina, M., Delmaki, A. E. F. & Benkirane, M. Contribution of remote sensing and GIS to analysis of the risk of flooding in the Zat basin (High Atlas-Morocco). Nat. Hazards 108 , 1835–1851. https://doi.org/10.1007/s11069-021-04758-x (2021).
Desalegn, H. & Mulu, A. Mapping flood inundation areas using GIS and HEC-RAS model at Fetam river, upper Abbay basin Ethiopia. Sci. Afr. 12 , e00834. https://doi.org/10.1016/j.sciaf.2021.e00834 (2021).
Article CAS Google Scholar
Hermas, E., Gaber, A. & El Bastawesy, M. Application of remote sensing and GIS for assessing and proposing mitigation measures in flood-affected urban areas Egypt. Egypt. J. Remote Sens. Space Sci. 24 , 119–130. https://doi.org/10.1016/j.ejrs.2020.03.002 (2021).
Portugués-Mollá, I., Bonache-Felici, X., Mateu-Bellés, J. F. & Marco-Segura, J. B. A GIS-based model for the analysis of an urban flash flood and its hydro-geomorphic response. The Valencia event of 1957. J. Hydrol. 541 , 582–596. https://doi.org/10.1016/j.jhydrol.2016.05.048 (2016).
Samela, C., Albano, R., Sole, A. & Manfreda, S. A GIS tool for cost-effective delineation of flood-prone areas. Comput. Environ. Urban Syst. 70 , 43–52. https://doi.org/10.1016/j.compenvurbsys.2018.01.013 (2018).
Mohamed, S. A. Application of satellite image processing and GIS-Spatial modeling for mapping urban areas prone to flash floods in Qena governorate. Egypt. J. Afric. Earth Sci. 158 , 103507. https://doi.org/10.1016/j.jafrearsci.2019.05.015 (2019).
Szydłowski, M. et al. In 14th International Symposium Water Management and Hydraulic Engineering.
Assaf, A. T., Sayl, K. N. & Adham, A. In Journal of Physics: Conference Series. 012149 (IOP Publishing).
Mohammed, O. & Sayl, K. In IOP Conference Series: Earth and Environmental Science. 012049 (IOP Publishing).
Courty, L. G., Rico-Ramirez, M. Á. & Pedrozo-Acuña, A. The significance of the spatial variability of rainfall on the numerical simulation of urban floods. Water 10 , 207 (2018).
Notaro, V., Fontanazza, C. M., Freni, G. & Puleo, V. Impact of rainfall data resolution in time and space on the urban flooding evaluation. Water Sci. Technol. 68 , 1984–1993. https://doi.org/10.2166/wst.2013.435 (2013).
Article PubMed Google Scholar
Rahmani, V., Hutchinson, S. L., Harrington, J. A. Jr. & Hutchinson, J. M. S. Analysis of frequency and magnitude of extreme rainfall events with potential impacts on flooding: A case study from the central United States. Int. J. Climatol. 36 , 3578–3587. https://doi.org/10.1002/joc.4577 (2016).
Faisal Koko, A., Yue, W., AbdullahiAbubakar, G., Hamed, R. & Noman Alabsi, A. A. Analyzing urban growth and land cover change scenario in Lagos, Nigeria using multi-temporal remote sensing data and GIS to mitigate flooding. Geomat. Nat. Hazards Risk 12 , 631–652. https://doi.org/10.1080/19475705.2021.1887940 (2021).
Hussein, K., Alkaabi, K., Ghebreyesus, D., Liaqat, M. U. & Sharif, H. O. Land use/land cover change along the eastern coast of the UAE and its impact on flooding risk. Geomat. Nat. Haz. Risk 11 , 112–130. https://doi.org/10.1080/19475705.2019.1707718 (2020).
Moniruzzaman, M. et al. Decadal Urban land use/land cover changes and its impact on surface runoff potential for the Dhaka city and surroundings using remote sensing. Remote Sens. 13 , 83 (2021).
Pabi, O., Egyir, S. & Attua, E. M. Flood hazard response to scenarios of rainfall dynamics and land use and land cover change in an urbanized river basin in Accra Ghana. City Environ. Interact. 12 , 100075. https://doi.org/10.1016/j.cacint.2021.100075 (2021).
Johnson, B. A. et al. High-resolution urban change modeling and flood exposure estimation at a national scale using open geospatial data: A case study of the Philippines. Comput. Environ. Urban Syst. 90 , 101704 (2021).
Tierolf, L., de Moel, H. & van Vliet, J. Modeling urban development and its exposure to river flood risk in Southeast Asia. Comput. Environ. Urban Syst. 87 , 101620 (2021).
Cabrera, J. S. & Lee, H. S. Flood risk assessment for Davao oriental in the Philippines using geographic information system-based multi-criteria analysis and the maximum entropy model. J. Flood Risk Manag. 13 , e12607. https://doi.org/10.1111/jfr3.12607 (2020).
Mahmoud, S. H. & Gan, T. Y. Urbanization and climate change implications in flood risk management: Developing an efficient decision support system for flood susceptibility mapping. Sci. Total Environ. 636 , 152–167. https://doi.org/10.1016/j.scitotenv.2018.04.282 (2018).
Oubennaceur, K., Chokmani, K., Nastev, M., Lhissou, R. & El Alem, A. Flood risk mapping for direct damage to residential buildings in Quebec, Canada. Int. J. Disaster Risk Reduct. 33 , 44–54. https://doi.org/10.1016/j.ijdrr.2018.09.007 (2019).
Rincón, D., Khan, U. T. & Armenakis, C. Flood risk mapping using GIS and multi-criteria analysis: A greater Toronto area case study. Geosciences 8 , 275. https://doi.org/10.3390/geosciences8080275 (2018).
Amen, M. et al. Mapping of flood-prone areas utilizing GIS techniques and remote sensing: A case study of Duhok Kurdistan Region of Iraq. Remote Sens. 15 , 1102 (2023).
Mohammed, S. S., Sayl, K. N. & Kamel, A. H. Ground water recharge mapping in Iraqi Western desert. Int. J. Des. Nat. Ecodyn. 17 , 913–920. https://doi.org/10.18280/ijdne.170612 (2022).
Muneer, A. S., Sayl, K. N. & Kamal, A. H. Modeling of spatially distributed infiltration in the Iraqi western desert. Appl. Geomat. 13 , 467–479. https://doi.org/10.1007/s12518-021-00363-6 (2021).
Szeląg, B. et al. Influence of urban catchment characteristics and rainfall origins on the phenomenon of stormwater flooding: Case study. Environ. Model. Softw. 150 , 105335. https://doi.org/10.1016/j.envsoft.2022.105335 (2022).
Sayl, K. N., Sulaiman, S. O., Kamel, A. H. & Al-Ansari, N. Towards the generation of a spatial hydrological soil group map based on the radial basis network model and spectral reflectance band recognition. Int. J. Des. Nat. Ecodyn. 17 , 761–766 (2022).
El-Saoud, W. A. & Othman, A. An integrated hydrological and hydraulic modelling approach for flash flood hazard assessment in eastern Makkah city, Saudi Arabia. J. King Saud. Univ. Sci. 34 , 102045. https://doi.org/10.1016/j.jksus.2022.102045 (2022).
Szydlowski, M. Transboundary floods: Reducing risks through flood management (Springer, 2006).
Google Scholar
Néelz, S. & Pender, G. Benchmarking the latest generation of 2D hydraulic modelling packages. Environment Agency, Horison House, Deanery Road, Bristol, BS1 9AH (2013).
Glenis, V., Kutija, V. & Kilsby, C. G. A fully hydrodynamic urban flood modelling system representing buildings, green space and interventions. Environ. Model. Softw. 109 , 272–292. https://doi.org/10.1016/j.envsoft.2018.07.018 (2018).
Macalalad, R. V. et al. Flash flood modeling in the data-poor basin: A case study in Matina river basin. Trop. Cyclone Res. Rev. 10 , 87–95. https://doi.org/10.1016/j.tcrr.2021.06.003 (2021).
Costabile, P., Costanzo, C., Ferraro, D., Macchione, F. & Petaccia, G. Performances of the new HEC-RAS version 5 for 2-D hydrodynamic-based rainfall-runoff simulations at basin scale: Comparison with a state-of-the art model. Water 12 , 2326. https://doi.org/10.3390/w12092326 (2020).
Mustafa, A. & Szydłowski, M. Application of different building representation techniques in HEC-RAS 2-D for urban flood modeling using the Toce River experimental case. PeerJ 9 , e11667. https://doi.org/10.7717/peerj.11667 (2021).
Alipour, A., Jafarzadegan, K. & Moradkhani, H. Global sensitivity analysis in hydrodynamic modeling and flood inundation mapping. Environ. Model. Softw. 152 , 105398. https://doi.org/10.1016/j.envsoft.2022.105398 (2022).
Ibrahim, R. I., Mushatat, S. A. & Abdelmonem, M. G. Erbil. Cities 49 , 14–25. https://doi.org/10.1016/j.cities.2015.07.001 (2015).
Gunes, C. Kurds in a new middle east (Springer, 2019).
Book Google Scholar
Mustafa, A. M., Muhammed, H. H. & Szydlowski, M. Extreme rainfalls as a cause of urban flash floods; A case study of the Erbil-Kurdistan region of IRAQ. Acta Scientiarum Polonorum. Formatio Circumiectus 18 , 113–132. https://doi.org/10.15576/asp.Fc/2019.18.3.113 (2019).
Löwe, R. et al. Impacts of urban development on urban water management–limits of predictability. Comput. Environ. Urban Syst. 84 , 101546. https://doi.org/10.1016/j.compenvurbsys.2020.101546 (2020).
Gizaw, M. S. & Gan, T. Y. Possible impact of climate change on future extreme precipitation of the oldman, bow and red deer river basins of Alberta. Int. J. Climatol. 36 , 208–224. https://doi.org/10.1002/joc.4338 (2016).
Jiang, R., Gan, T. Y., Xie, J., Wang, N. & Kuo, C.-C. Historical and potential changes of precipitation and temperature of Alberta subjected to climate change impact: 1900–2100. Theoret. Appl. Climatol. 127 , 725–739. https://doi.org/10.1007/s00704-015-1664-y (2017).
Courty, L. G., Wilby, R. L., Hillier, J. K. & Slater, L. J. Intensity-duration-frequency curves at the global scale. Environ. Res. Lett. 14 , 084045. https://doi.org/10.1088/1748-9326/ab370a (2019).
Noor, M., Ismail, T., Chung, E.-S., Shahid, S. & Sung, J. H. Uncertainty in rainfall intensity duration frequency curves of peninsular Malaysia under changing climate scenarios. Water 10 , 1750. https://doi.org/10.3390/w10121750 (2018).
ShahabulAlam, M. & Elshorbagy, A. Quantification of the climate change-induced variations in intensity–duration–frequency curves in the Canadian prairies. J. Hydrol. 527 , 990–1005. https://doi.org/10.1016/j.jhydrol.2015.05.059 (2015).
Kareem, D. A., Amen, A. R., Mustafa, A., Yüce, M. I. & Szydłowski, M. Comparative analysis of developed rainfall intensity–duration–frequency curves for Erbil with other Iraqi Urban areas. Water 14 , 419. https://doi.org/10.3390/w14030419 (2022).
Bouwer, L. M., Bubeck, P. & Aerts, J. C. J. H. Changes in future flood risk due to climate and development in a Dutch polder area. Glob. Environ. Chang. 20 , 463–471. https://doi.org/10.1016/j.gloenvcha.2010.04.002 (2010).
de Kok, J.-L. & Grossmann, M. Large-scale assessment of flood risk and the effects of mitigation measures along the Elbe river. Nat. Hazards 52 , 143–166. https://doi.org/10.1007/s11069-009-9363-6 (2010).
Gouldby, B. Uncertainty and sensitvity analysis method for flood risk analysis. T24–08–01 (2009).
Relifeweb. Iraq: Flash Floods - Dec 2021 , < https://reliefweb.int/disaster/fl-2021-000208-irq > (2021).
Erbil Govornorate. Media Statement , < https://www.hawlergov.org/app/ku/node/2146 > (2021).
Kurdistan Region Statics, O. Report of the expectation of Kurdistan Region Population from 2009–2020. (Erbil- Kurdistan Region of Iraq, 2014).
Al-Ansari, N. Management of water resources in Iraq: Perspectives and prognoses. Engineering 5 , 667–684 (2013).
Hameed, H. M. Estimating the effect of urban growth on annual runoff volume using GIS in the Erbil sub-basin of the Kurdistan region of Iraq. Hydrology 4 , 12. https://doi.org/10.3390/hydrology4010012 (2017).
Li, J. & Wong, D. W. Effects of DEM sources on hydrologic applications. Comput. Environ. Urban Syst. 34 , 251–261. https://doi.org/10.1016/j.compenvurbsys.2009.11.002 (2010).
NRCS, U. Chapter 7–Hydrologic soil groups in: NRCS–National engineering handbook (NEH), Part 630–hydrology. USDA NRCS, Washington, DC , 7.1–7.5 (2009).
Brunner, G. W. C.-H. HEC-RAS river analysis system 2D modeling user’s manual . (US Army Corps of Engineers—Hydrologic Engineering Center, 2021).
Brunner, G. W. (2021) HEC-RAS River Analysis System2D Hydraulic reference manual, Version 6.0 (US Army Corps of Engineers—Hydrologic Engineering Center, London).
Aronica, G. & Lanza, L. Drainage efficiency in urban areas: A case study. Hydrol. Process. Int. J. 19 , 1105–1119. https://doi.org/10.1002/hyp.5648 (2005).
Gallien, T., Schubert, J. & Sanders, B. Predicting tidal flooding of urbanized embayments: A modeling framework and data requirements. Coast. Eng. 58 , 567–577. https://doi.org/10.1016/j.coastaleng.2011.01.011 (2011).
Hunter, N. et al. In Proceedings of the Institution of Civil Engineers-Water Management. 13–30 (Thomas Telford Ltd).
Li, Z. et al. Comparative analysis of building representations in TELEMAC-2D for flood inundation in idealized Urban districts. Water 11 , 1840. https://doi.org/10.3390/w11091840 (2019).
Schubert, J. E. & Sanders, B. F. Building treatments for urban flood inundation models and implications for predictive skill and modeling efficiency. Adv. Water Resour. 41 , 49–64. https://doi.org/10.1016/j.advwatres.2012.02.012 (2012).
Chow, V. T. Open-channel hydraulics (McGraw-Hill civil engineering series, 1959).
Cronshey, R. Urban hydrology for small watersheds (US Department of Agriculture Soil Conservation Service, Engineering Division, 1986).
Huff, F. A. Time distribution of rainfall in heavy storms. Water Resour. Res. 3 , 1007–1019. https://doi.org/10.1029/WR003i004p01007 (1967).
Mark, O., Weesakul, S., Apirumanekul, C., Aroonnet, S. B. & Djordjević, S. Potential and limitations of 1D modelling of urban flooding. J. Hydrol. 299 , 284–299. https://doi.org/10.1016/j.jhydrol.2004.08.014 (2004).
Gharbi, M., Soualmia, A., Dartus, D. & Masbernat, L. Comparison of 1D and 2D hydraulic models for floods simulation on the Medjerda Riverin Tunisia. J. Mater. Environ. Sci 7 , 3017–3026 (2016).
Liu, Y. & Pender, G. Carlisle 2005 urban flood event simulation using cellular automata-based rapid flood spreading model. Soft. Comput. 17 , 29–37. https://doi.org/10.1007/s00500-012-0898-1 (2013).
Shen, D., Wang, J., Cheng, X., Rui, Y. & Ye, S. Integration of 2-D hydraulic model and high-resolution lidar-derived DEM for floodplain flow modeling. Hydrol. Earth Syst. Sci. 19 , 3605–3616. https://doi.org/10.5194/hess-19-3605-2015 (2015).
Dazzi, S., Shustikova, I., Domeneghetti, A., Castellarin, A. & Vacondio, R. Comparison of two modelling strategies for 2D large-scale flood simulations. Environ. Model. Softw. 146 , 105225. https://doi.org/10.1016/j.envsoft.2021.105225 (2021).
Yalcin, E. Assessing the impact of topography and land cover data resolutions on two-dimensional HEC-RAS hydrodynamic model simulations for urban flood hazard analysis. Nat. Hazards 101 , 995–1017. https://doi.org/10.1007/s11069-020-03906-z (2020).
Surwase, T. et al. In Proceedings of International Conference on Remote Sensing for Disaster Management. 851–863 (Springer).
Liu, Y., Zhang, W. & Cui, X. Flood emergency management using hydrodynamic modelling. Procedia Eng. 28 , 750–753. https://doi.org/10.1016/j.proeng.2012.01.802 (2012).
Campana, N. A. & Tucci, C. E. Predicting floods from urban development scenarios: Case study of the Dilúvio Basin, Porto Alegre Brazil. Urban Water 3 , 113–124. https://doi.org/10.1016/S1462-0758(01)00004-8 (2001).
Rudaw Meida Network. Daily NEWS < https://www.rudaw.net/ > (2021).
Sissakian, V. K., Al-Ansari, N., Adamo, N., Abdul Ahad, I. D. & Abed, S. A. Flood hazards in Erbil city Kurdistan region Iraq, 2021: A case study. Engineering 14 , 591–601. https://doi.org/10.4236/eng.2022.1412044 (2022).
Gigović, L., Pamučar, D., Bajić, Z. & Drobnjak, S. Application of GIS-interval rough AHP methodology for flood hazard mapping in urban areas. Water 9 , 360. https://doi.org/10.3390/w9060360 (2017).
Sameer, Y. M., Abed, A. N. & Sayl, K. N. In Journal of Physics: Conference Series. 012060 (IOP Publishing).
Sameer, Y. M., Abed, A. N. & Sayl, K. N. Geomatics-based approach for highway route selection. Appl. Geomat. 15 , 161–176. https://doi.org/10.1007/s12518-023-00495-x (2023).
Mattos, T. S. et al. Towards reducing flood risk disasters in a tropical urban basin by the development of flood alert web application. Environ. Model. Softw. 151 , 105367. https://doi.org/10.1016/j.envsoft.2022.105367 (2022).
Download references
This research did not receive any specific grant from funding agencies in the public, commercial, or not-for-profit sectors.
Author information
Authors and affiliations.
Faculty of Civil and Environmental Engineering, Gdańsk University of Technology, Narutowicza 11/12, 80-233, Gdańsk, Poland
Andam Mustafa & Michał Szydłowski
Department of Physical Geography and Ecosystem Science, Lund University, Lund, Sweden
Mozafar Veysipanah
College of Engineering, Geomatics (Surveying) Engineering, Salahaddin University-Erbil, Erbil, 44001, Iraq
Hasan Mohammed Hameed
You can also search for this author in PubMed Google Scholar
Contributions
Conceptualization, A. M. and M. S.; methodology, A. M. and M. V.; software, A. M. and M. V.; formal analysis, A. M. and M. V.; investigation, A. M. and H. M. H.; resources, A. M. and H. M. H.; writing—original draft preparation, A. M.; writing—review and editing, A. M., M. S., M. V. and H. M. H.; supervision, M. S. and H. M. H.. All authors have read and agreed to the published version of the manuscript.
Corresponding author
Correspondence to Andam Mustafa .
Ethics declarations
Competing interests.
The authors declare that they have no known competing financial interests or personal relationships that could have appeared to influence the work reported in this paper.
Additional information
Publisher's note.
Springer Nature remains neutral with regard to jurisdictional claims in published maps and institutional affiliations.
Rights and permissions
Open Access This article is licensed under a Creative Commons Attribution 4.0 International License, which permits use, sharing, adaptation, distribution and reproduction in any medium or format, as long as you give appropriate credit to the original author(s) and the source, provide a link to the Creative Commons licence, and indicate if changes were made. The images or other third party material in this article are included in the article's Creative Commons licence, unless indicated otherwise in a credit line to the material. If material is not included in the article's Creative Commons licence and your intended use is not permitted by statutory regulation or exceeds the permitted use, you will need to obtain permission directly from the copyright holder. To view a copy of this licence, visit http://creativecommons.org/licenses/by/4.0/ .
Reprints and permissions
About this article
Cite this article.
Mustafa, A., Szydłowski, M., Veysipanah, M. et al. GIS-based hydrodynamic modeling for urban flood mitigation in fast-growing regions: a case study of Erbil, Kurdistan Region of Iraq. Sci Rep 13 , 8935 (2023). https://doi.org/10.1038/s41598-023-36138-9
Download citation
Received : 24 March 2023
Accepted : 30 May 2023
Published : 01 June 2023
DOI : https://doi.org/10.1038/s41598-023-36138-9
Share this article
Anyone you share the following link with will be able to read this content:
Sorry, a shareable link is not currently available for this article.
Provided by the Springer Nature SharedIt content-sharing initiative
By submitting a comment you agree to abide by our Terms and Community Guidelines . If you find something abusive or that does not comply with our terms or guidelines please flag it as inappropriate.
Quick links
- Explore articles by subject
- Guide to authors
- Editorial policies
Sign up for the Nature Briefing: Anthropocene newsletter — what matters in anthropocene research, free to your inbox weekly.

share this!
May 30, 2024
This article has been reviewed according to Science X's editorial process and policies . Editors have highlighted the following attributes while ensuring the content's credibility:
fact-checked
peer-reviewed publication
trusted source
Model simulates urban flood risk with an eye to equity
by Tara Roberts, Stanford University
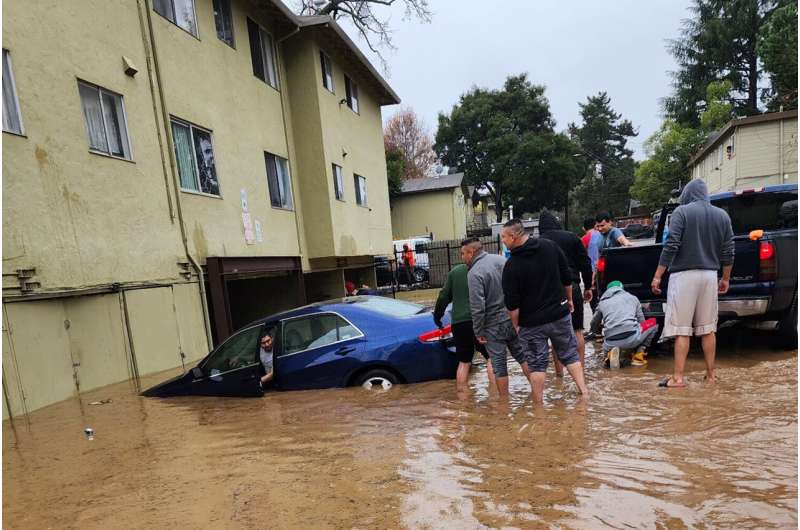
Plans for flood mitigation along urban rivers often benefit some neighborhoods more than others. Researchers and collaborators in a densely populated California floodplain developed a way to help planners see how infrastructure designs, sea-level rise, and severe storms fueled by climate change will affect flood risk at the local level.
Rising seas and extreme storms fueled by climate change are combining to generate more frequent and severe floods in cities along rivers and coasts, and aging infrastructure is poorly equipped for the new reality. But when governments and planners try to prepare communities for worsening flood risks by improving infrastructure, the benefits are often unfairly distributed.
A new modeling approach from Stanford University and University of Florida researchers offers a solution: an easy way for planners to simulate future flood risks at the neighborhood level under conditions expected to become commonplace with climate change, such as extreme rainstorms that coincide with high tides elevated by rising sea levels.
The approach, published May 28 in Environmental Research Letters , reveals places where elevated risk is invisible with conventional modeling methods designed to assess future risks based on data from a single past flood event.
"Asking these models to quantify the distribution of risk along a river for different climate scenarios is kind of like asking a microwave to cook a sophisticated souffle. It's just not going to go well," said senior study author Jenny Suckale, an associate professor of geophysics at the Stanford Doerr School of Sustainability. "We don't know how the risk is distributed, and we don't look at who benefits, to which degree."
A history of destructive floods
The new study came about through collaboration with regional planners and residents in bayside cities including East Palo Alto, which faces rising flood risks from the San Francisco Bay and from an urban river that snakes along its southeastern border.
The river, known as the San Francisquito Creek, meanders from the foothills above Stanford's campus down through engineered channels to the bay—its historic floodplains long ago developed into densely populated cities. "We live around it, we drive around it, we drive over it on the bridges," said lead study author Katy Serafin, a former postdoctoral scholar in Suckale's research group.
The river has a history of destructive floods. The biggest one, in 1998, inundated 1,700 properties, caused more than $40 million in damages, and led to the creation of a regional agency tasked with mitigating future flood risk.
Nearly 20 years after that historic flood, Suckale started thinking about how science could inform future flood mitigation efforts around urban rivers like the San Francisquito when she was teaching a course in East Palo Alto focused on equity, resilience, and sustainability in urban areas. Designated as a Cardinal Course for its strong public service component, the course was offered most recently under the title Shaping the Future of the Bay Area.
Around the time Suckale started teaching the course, the regional agency—known as the San Francisquito Creek Joint Powers Authority—had developed plans to redesign a bridge to allow more water to flow underneath it and prevent flooding in creekside cities. But East Palo Alto city officials told Suckale and her students that they worried the plan could worsen flood risks in some neighborhoods downstream of the bridge.
Suckale realized that if the students and scientists could determine how the proposed design would affect the distribution of flood risks along the creek, while collaborating with the agency to understand its constraints, then their findings could guide decisions about how to protect all neighborhoods. "It's actionable science, not just science for science's sake," she said.
Science that leads to action
The Joint Powers Authority had developed the plan using a flood-risk model commonly used by hydrologists around the world. The agency had considered the concerns raised by East Palo Alto city staff about downstream flood risks, but found that the standard model couldn't substantiate them.
"We wanted to model a wider range of factors that will contribute to flood risk over the next few decades as our climate changes," said Serafin, who served as a mentor to students in the Cardinal Course and is now an assistant professor at University of Florida.
Serafin created an algorithm to simulate millions of combinations of flood factors, including sea-level rise and more frequent episodes of extreme rainfall—a consequence of global warming that is already being felt in East Palo Alto and across California .
Serafin and Suckale incorporated their new algorithm into the widely used model to compute the statistical likelihood that the San Francisquito Creek would flood at different locations along the river. They then overlaid these results with aggregated household income and demographic data and a federal index of social vulnerability .
They found that the redesign of the upstream bridge would provide adequate protection against a repeat of the 1998 flood, which was once considered a 75-year flood event. But the modeling revealed that the planned design would leave hundreds of low-income households in East Palo Alto exposed to increased flood risk as climate change makes once-rare severe weather and flood events more common.
A beneficial collaboration
When the scientists shared their findings with the city of East Palo Alto, the Joint Powers Authority, and other community collaborators in conversations over several years, they emphasized that the conventional model wasn't wrong—it just wasn't designed to answer questions about equity.
The results provided scientific evidence to guide the Joint Powers Authority's infrastructure plans, which expanded to include construction of a permanent floodwall beside the creek in East Palo Alto. The agency also adopted a plan to build up the creek's bank in a particularly low area to better protect neighboring homes and streets.
Ruben Abrica, East Palo Alto's elected representative to the Joint Powers Authority board, said researchers, planners, city staff, and policymakers have a responsibility to work together to "carry out projects that don't put some people in more danger than others."
The results of the Stanford research demonstrated how seemingly neutral models that ignore equity can lead to uneven distributions of risks and benefits. "Scientists have to become more aware of the impact of the research, because the people who read the research or the people who then do the planning are relying on them," he said.
Serafin and Suckale said their work with San Francisquito Creek demonstrates the importance of mutual respect and trust among researchers and communities positioned not as subjects of study, but active contributors to the creation of knowledge.
"Our community collaborators made sure we, as scientists, understood the realities of these different communities," Suckale said. "We're not training them to be hydrological modelers. We are working with them to make sure that the decisions they're making are transparent and fair to the different communities involved."
The new approach to modeling flood risk can help city and regional planners create better flood risk assessments and avoid creating new inequities, Suckale said. The algorithm is publicly available for other researchers to adapt to their location.
Journal information: Environmental Research Letters
Provided by Stanford University
Explore further
Feedback to editors

New imager acquires amplitude and phase information without digital processing
23 minutes ago

AI helps scientists understand cosmic explosions
36 minutes ago

'Forever chemical' discovery can aid drinking water treatment
42 minutes ago

Mountain building linked to major extinction event half a billion years ago
46 minutes ago

News from 'El Gordo': Study suggests dark matter may have collisional properties after all
54 minutes ago

Clues to mysterious disappearance of North America's large mammals 50,000 years ago found within ancient bone collagen

Researchers discover 'Trojan Horse' virus hiding in human parasite

An outlandish molecule may be lurking inside Uranus and Neptune, affecting their magnetic fields

A new way of designing auxetic materials

Glimpses of a volcanic world: New telescope images of Jupiter's moon Io rival those from spacecraft
Relevant physicsforums posts, iceland warming up again - quakes swarming.
May 29, 2024

Mount Ibu, Indonesia erupts
Adirondack mountains and earthquakes.
May 23, 2024
The Secrets of Prof. Verschure's Rosetta Stones
Mt. vesuvius 1944 eruption light show -- static electricity.
May 22, 2024
Can a glass of water be filled to its edge?
May 21, 2024
More from Earth Sciences
Related Stories

Sea-level rise may worsen existing Bay Area inequities
Jul 12, 2021

Bay Area coastal flooding triggers regionwide commute disruptions
Aug 5, 2020

San Francisco Bay study highlights value of salt marsh restoration for flood risk reduction and climate resilience
Apr 11, 2024

Communities must get prepared for increased flooding due to climate change, expert warns
Feb 18, 2024

Mapping floods of the future reveals communities at risk due to climate change
Apr 25, 2023

Extreme UK flood levels are happening much more often than they used to, analysis shows
Jan 11, 2024
Recommended for you

No new fossil fuel projects needed in the transition to Net Zero, researchers say
2 hours ago

Study of radioiodine sorption and transport could help protect groundwater

Novel mobile air monitoring technology yields greater insight into post-disaster pollution levels
19 hours ago

Historic iceberg surges offer insights on modern climate change
23 hours ago

Study suggests faster decomposition rates in waterways could exacerbate greenhouse gas emissions, threaten biodiversity

Reduced sulfur content in shipping fuel associated with increased maritime atmospheric warming
Let us know if there is a problem with our content.
Use this form if you have come across a typo, inaccuracy or would like to send an edit request for the content on this page. For general inquiries, please use our contact form . For general feedback, use the public comments section below (please adhere to guidelines ).
Please select the most appropriate category to facilitate processing of your request
Thank you for taking time to provide your feedback to the editors.
Your feedback is important to us. However, we do not guarantee individual replies due to the high volume of messages.
E-mail the story
Your email address is used only to let the recipient know who sent the email. Neither your address nor the recipient's address will be used for any other purpose. The information you enter will appear in your e-mail message and is not retained by Phys.org in any form.
Newsletter sign up
Get weekly and/or daily updates delivered to your inbox. You can unsubscribe at any time and we'll never share your details to third parties.
More information Privacy policy
Donate and enjoy an ad-free experience
We keep our content available to everyone. Consider supporting Science X's mission by getting a premium account.
E-mail newsletter
INTRODUCTION
Methodology, case studies and results, conclusions, data availability statement, conflict of interest, urban flood modeling with a novel coupling method of surface and sewer hydrodynamic processes.

- Article contents
- Figures & tables
- Supplementary Data
- Open the PDF for in another window
- Guest Access
- Cite Icon Cite
- Permissions
- Search Site
Siyi Wang , Jiaying Wang , Kunlun Xin , Hexiang Yan , Shuping Li , Tao Tao; Urban flood modeling with a novel coupling method of surface and sewer hydrodynamic processes. Water Sci Technol 2024; wst2024172. doi: https://doi.org/10.2166/wst.2024.172
Download citation file:
- Ris (Zotero)
- Reference Manager
Drainage modeling that accurately captures urban storm inundation serves as the foundation for flood warning and drainage scheduling. In this paper, we proposed a novel coupling ideology that, by integrating 2D-1D and 1D-2D unidirectional processes, overcomes the drawback of the conventional unidirectional coupling approach that fails to properly represent the rainfall surface catchment dynamics, and provides more coherent hydrological implications compared to the bidirectional coupling concept. This paper first referred to a laboratory experimental case from the literature, applied and analyzed the coupling scheme proposed in this paper and the bidirectional coupling scheme that has been widely studied in recent years, compared the two coupling solutions in terms of the resulting accuracy and applicability, and discussed their respective strengths and weaknesses to validate the reliability of the proposed method. The verified proposed coupling scheme was then applied to the modeling of a real drainage system in a region of Nanjing, China, and the results proved that the coupling mechanism proposed in this study is of practical application value.
A novel coupling approach by combining two unidirectional processes.
Comparison with bidirectional tight coupling scheme in terms of simulation accuracy and applicability.
The proposed coupling idea provides reliable outcomes with enhanced applicability.
The global climate change and urbanization process have contributed to an increased frequency of flooding, which has become the most frequent weather-related hazard in the last two decades, affecting 2.3 billion people worldwide, more than all other weather-related hazards combined ( Wallemacq et al. 2015 ). At the same time, flooding is one of the world's most destructive natural disasters, not only endangering lives and property but also having a serious negative impact on society and the environment ( Han & He 2021 ; Dong et al. 2022 ). It is essential to develop an efficient and trustworthy urban drainage model appropriate for various rainfall scenarios and diverse geographical regions to comprehend the development progress of urban flooding, which is the foundation for mitigating flood damage.
The sewer network flow and the overland surface flow constitute two distinct subsystems that jointly make up the urban drainage system. The simulation of overland surface flow is typically carried out with either simplified conceptual model-based hydrological methods or numerical model-based hydrodynamic methods (including one- and two-dimensional hydraulic models). Conceptual model-based hydrological and 1D hydrodynamic approaches for solving the shallow water equations (SWEs), while computationally efficient, are not as accurate as physically based 2D hydrodynamic methods ( Djordjević et al. 1999 ; Teng et al. 2017 ; Sañudo et al. 2020 ). Modeling sewer flow is usually accomplished by solving the 1D Saint-Venant equations to examine the ability of a sewer system to cope with intense rainfall ( Djordjević et al. 1999 ; Rossman & Simon 2022 ). The 1D model simulates the water hydrodynamics in the sewer network with high precision and rapid calculation, but has evident flaws when simulating urban structures (such as buildings) with obvious 2D and 3D characteristics. The 2D model deals primarily with surface water movement, but it is unable to simulate the water flow dynamics in the drainage network as effectively as the 1D model ( Dong et al. 2021 ). Therefore, coupled 1D sewer flow and 2D overland flow models for urban inundation modeling have been extensively investigated over the last few years ( Leandro & Martins 2016 ; Noh et al. 2016 ; Dong et al. 2022 ).
The first 1D/2D coupling was developed by Hsu et al. (2000) who used Stormwater Management Model (SWMM) to analyze the flow dynamics in a drainage network and treated its overflow as inputs to a 2D overland flow model. The process of returning overflow to the network is not accounted for with this coupling technique, and therefore, the extent and depth of flooding in the downstream area are often overestimated. In an attempt to overcome this limitation, some academic scholars incorporated the consideration of the reflux process to define the 1D/2D bidirectional interactions ( Hsu et al. 2002 ; Seyoum et al. 2012 ). These studies can be further categorized into two classes according to whether or not the surface convergence process is represented in 2D. The first category applies rainfall to the 1D hydrologic model, calculating sewer flow first and then interfacing the overload with the 2D surface flow model. The second category directly adds rainfall to the 2D model, where both surface catchment and network overflow are computed from the 2D model. Flood estimation may be erroneous if the rainfall intensity exceeds the intended capacity of the sewer inlets because the hydrologic model's catchment process assumes that runoff from sub-catchments is fully collected and discharged into the sewer network. In contrast, it is a fact that in urban areas, rainfall turns into runoff immediately after it drops to the ground surface and travels across the landscape for a certain distance before it reaches a drainage inlet or manhole. A simplified hydrologic model is unable to adequately clarify this phenomenon. The findings of Chang et al. (2015) further demonstrated that the 1D/2D coupling should apply rainfall directly to the 2D model to describe the precipitation catchment process rather than employing hydrologic routing.
The previously described bidirectional coupling strategy expresses two-way interactions in terms of water exchanges between the sewer system and the surface water system, where the interaction flow is commonly a simplified calculation based on the weir or orifice equations applied to the point-source head gradient. The coefficients within these manhole flow exchange equations bear stochastic properties that inject a degree of uncertainty and potential error into the estimated flow rates ( Zhang et al. 2023 ). And achieving coherence in simulation results necessitates the use of exceedingly small time step, invariably sacrificing the temporal smoothness of the model. Furthermore, the development of the closely coupled model involves a heightened research threshold that not only demands a profound comprehension of the 1D and 2D system dynamics but also requires substantial programming expertise. Although there exists some commercial software with strong generalization and stable performance, such as InfoWorks ICM, MIKE, and TUFLOW ( Syme 1992 ; Innovyze 2019 ; DHI 2020 ), they are not open-source and costly to utilize. The current unidirectional coupling methods, which are relatively convenient to implement, tend to only consider the transformation of the overflow from the 1D pipe network to the 2D model, making the results comparatively imprecise. By combining two unidirectional processes – feeding the 2D confluence into the 1D sewer network, and vice versa, channeling the overflow from the 1D network back into the 2D model – we can adopt a straightforward and manageable approach that addresses the conventional unidirectional process's inability to realistically depict the rainfall-runoff process. However, to our knowledge, no studies have yet attempted to compare the effectiveness of this integrated unidirectional method with that of the bidirectional coupling strategies.
To address the above issues, this paper investigated the coupling technique to merge the outcomes of two unidirectional processes and contrasted it with the bidirectional coupling method in terms of the result correctness, calculated stability, and implementation requirements. The 2D model was applied to mimic the rainfall catchment and surface diffusion process, whereas the 1D model solely analyzes the internal flow of the sewer network. The 2D model adopted Telemac-2D and the 1D model employed SWMM ( Moulinec et al. 2011 ; McDonnell et al. 2021 ), both of which are open source and hence free and accessible. The details of the two coupling approaches are covered in the section that follows.
The paper is organized as follows: first, a detailed procedural description is presented for both the new coupling scheme proposed in this study and the existing bidirectional coupling scheme; then, two case discussions are introduced to evaluate the effectiveness of the proposed methodology, where the first one cites a large-scale laboratory experiment from the literature to validate the correctness and reasonableness of the proposed model ( Naves et al. 2020 ), and the second one applies the method to simulate a real drainage system in a specific area of Nanjing, China, to validate the applicability of the methodology to large-scale drainage systems; and finally, the major conclusions are highlighted.
Hydraulic models
2d surface model.
Among several numerical solvers, Telemac-2D is preferred in this paper since it simulates the moving boundaries of flows on a horizontal plane by the finite element method, which offers the strength of faster speed, lower memory requirements, better robustness to absorb the drastic variation in the transition between wet and dry regions, and especially the availability of the open source ( Heniche et al. 2000 ). Hierarchical mesh resolution is possible with Telemac-2D finite element grids ( Hervouet 2007 ). The average vertical water depth and velocity at each point in the resolution grid are obtained by solving the SWEs on triangular or quadrilateral elements.
1D Sewer network model

Node-link representation of a conveyance network in SWMM.
The equations are discretized in finite difference form with spatial dispersion equal to the conduit length and are solved implicitly and iteratively using Picard's method over a given time step.
Linking methodology
The majority of surface water that contributes to urban flooding is collected in the subterranean sewer system through manholes and artificial outlets ( Dong et al. 2022 ). When the precipitation intensity surpasses the drainage capacity of the network, it causes rainwater overflow and the emergence of surface flooding.
Combination of two unidirectional couplings (CTUC)

Schematic diagram of the combination of two unidirectional coupled processes.
Eventually, the 2D model results of the two processes can be aggregated with the water depths at identical spatial locations within the same time step to yield a comprehensive simulation of the entire rainfall-runoff overflow flooding procedure. The fact is that if variable time steps are adopted, it is impossible for two different 2D models to be synchronized in time, and the grid vertex locality cannot correspond accurately. Therefore, in this study, the 2D model results of the 2D-1D process are taken as the base results, with the 2D model results of the 1D-2D process as the additional results. The bathymetry values of the additional results are linearly interpolated on the time scale to correspond to the time steps of the base results, and on the spatial scale the KD-Tree algorithm ( Bentley 1975 ) is employed to search for additional result nodes adjacent to the base result nodes at a certain distance (the mesh default size in this paper), and their average water depths are computed to be appended to the base result nodes' water depths. The treatment can be parallelized by partitioning the time series to improve computational efficiency.
The two straightforward and simple unidirectional coupling processes that make up the CTUC method's implementation eliminate the need to develop a customized 2D computational engine and coupling module and enable the direct adoption of pre-existing mature software (whether open source or not, e.g., LISFLOOD and HEC-RAS ( Shustikova et al. 2019 )) that focuses primarily on 2D simulation capabilities, offering a broader spectrum of application.
Bidirectional coupling (BC)
A precise characterization of the flow interaction between surface and sewer systems is important in the bidirectional coupling method. It synchronizes the simulation process of 1D and 2D models, and the water level difference between the node of the sewer network and the corresponding land surface is assessed at each time step to determine whether it is a nodal overflow or an inflow. Nodal inflow can be subdivided into free and submerged inflow according to the relation between the nodal water level and the adjacent surface elevation. Therefore, bidirectional coupling can be divided into the following three scenarios ( Chen et al. 2007 ).
Free weir linkage

Three scenarios of flow exchange through manholes: (a) free weir linkage; (b) submerged weir linkage; and (c) orifice linkage.
Submerged weir linkage
Orifice linkage.
A full-scale dual-drainage laboratory experimental case from the literature ( Naves et al. 2020 ), which emulated the hydraulic and sediment transport process using a realistic rainfall simulator, was adopted in this work and the differences between the two coupling schemes concerning accuracy and implementation were analyzed and compared as the first step in verifying the dependability of the CTUC method. Then, the CTUC method was utilized to simulate the performance of a real large-scale drainage system in NJ, China during a typical local rainfall circumstance.
Model validation using a laboratory experiment
Case study description.

Schematic of the laboratory physical model: (a) physical facility and (b) street, drainage network, and measuring points for results validation.
Rainfall simulators are capable of producing rainfall with intensities of 30, 50, and 80 mm/h. The simulation employed measured rainfall intensity maps to approximate the spatial distribution of actual rainfall. To compare the results of the CTUC and BC methods with the experimental data, the discharges from the two gully pots were selected as flow indicators, the measuring point S1 as surface water depth indicator, and the measuring points S2 and S3 as inside-pipe water depth indicators ( Figure 4 ) for reference.
Discretizing the surface domain with an unstructured triangular mesh of default size 0.05 m, the CTUC method generated a total of 29,508 elements, while the BC method created more elements irrespective of the boundary geometry, with a total of 30,186. The two gully pots and the outlet channel are set up as open boundaries for free outflow. Following previous studies conducted in this laboratory facility ( Naves et al. 2019a ), the Manning roughness coefficients for the surface and pipes were set at 0.016 and 0.008 s.m −1/3 , respectively. For the flow exchange formula of the BC method, the weir and orifice coefficients for the inlets were set at 0.52 and 0.62 after model testing, respectively.
Results and discussions
Comparison of CTUC and BC methods for the steady water discharges drained by the two gully pots

Comparisons between the simulated and experimental data of the CTUC and BC methods: (a) GP1; (b) GP2; (c) S1; (d) S2; (e) S3, and (f) the NSE values of all simulation scenarios.
Since the BC idea intervenes in the variable states of the two subsystems at each time step, it is critical to restrict the magnitude of the interactive fluxes to ensure the stability of their results. In this paper, the tuning of this limitation is manifested mostly in the coefficient α of Equation ( 13 ), where α is too large for the results to oscillate significantly, and α is too small to describe the exact amount of the interaction flow. The determination of α value is associated with the overall runoff quantity size and time step size, which is an arithmetic and time-consuming task. In addition, the BC concept cannot be implemented in a mature calculating engine without open source, which necessitates the developers to possess a profound mastery of both hydraulic mechanisms and computer programming. The CTUC approach, on the other hand, integrates two continuous hydrological processes and eliminates the necessity for additional stabilization safeguards. Moreover, the CTUC approach can be realized by utilizing mature commercial software for 2D and 1D computing that is widely available in the market, which allows for wider applicability.
Application of a real urban drainage system

Topography and drainage network layout of the study area.
The unstructured triangular mesh with a default resolution of 6 m was generated, and the CTUC method sets each manhole as a square open boundary. A finer grid resolution of 1 m was implemented at the boundaries, and the square sides were 2 m long. The total number of grids for the area was 1,192,979. A precipitation hydrograph with a duration of 2 h is shown in Figure 6 . The rainfall distribution is spatially homogeneous. The overall simulation duration was 4 h, and the time step was set to 5 s, satisfying the Courant–Friedrichs–Lewy (CFL) stability constraint ( Courant et al. 1967 ).

Spatial and temporal variations of the flood inundation process.

Temporal variations in accumulated volumes of rain, surface, entering the network, and outflow.

Spatial distribution of flooding water depth at the highest water volume.

Temporal distribution of water depth at checkpoints P1–P4.
Among the 1D/2D coupled models that are extensively employed to simulate urban stormwater inundation developments, one generally acknowledged viewpoint is that the unidirectional coupled model from 1D network overflow to 2D surface diffusion cannot accurately characterize the rainfall surface confluence process, and hence is less dependable than the bidirectional coupled model. However, this paper proposed a new coupling scheme CTUC that combines the two unidirectional coupling processes of 2D surface confluence to 1D network and network overflow to surface flooding, and compared it with the contemporary bidirectional coupling idea BC in terms of the result accuracy and implementation requirements. This study adopted the 1D engine SWMM and the 2D engine TELEMAC, both of which are proven software that are open source and stable in operation and maintenance.
Through a laboratory experiment case designed to mimic urban street flooding, we first verified the reliability of the proposed coupling approach. The simulation results obtained by the CTUC and BC methods were compared with the experimental findings, separately, indicating that the CTUC and BC methods produced comparable results in terms of accuracy, both of which can satisfy the engineering requirements. The CTUC solution outperformed the BC marginally in the surface water depth, while it was slightly inferior to the BC strategy in the pipe water depth. The CTUC concept has a better calculation stability and applicability range than the BC method. Afterward, we applied the CTUC method to establish an actual urban drainage system model in a region of Nanjing, China, to synchronize the spatial and temporal variation of waterlogging under a 3-year rainfall condition, and the results were consistent with the physical topography and engineering characteristics of the urban area, which provided an efficient theoretical basis for the urban flood control design and renovation.
To summarize, the CTUC method proposed in this paper can generate relatively satisfactory and accurate simulation results for large-scale drainage systems, with improved computational stability and lower implementation threshold.
This work was financially supported by the National Natural Science Foundation of China (NSFC) [Grant 51978493] and [Grant 51778452].
Data cannot be made publicly available; readers should contact the corresponding author for details.
The authors declare there is no conflict.
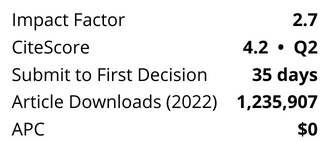
Affiliations
- ISSN 0273-1223 EISSN 1996-9732
- Open Access
- Collections
- Subscriptions
- Subscribe to Open
- Editorial Services
- Rights and Permissions
- Sign Up for Our Mailing List
- IWA Publishing
- Republic – Export Building, Units 1.04 & 1.05
- 1 Clove Crescent
- London, E14 2BA, UK
- Telephone: +44 208 054 8208
- Fax: +44 207 654 5555
- IWAPublishing.com
- IWA-network.org
- IWA-connect.org
- Cookie Policy
- Terms & Conditions
- Get Adobe Acrobat Reader
- ©Copyright 2024 IWA Publishing
This Feature Is Available To Subscribers Only
Sign In or Create an Account
Advertisement
Analysis of flood damage and influencing factors in urban catchments: case studies in Manila, Philippines, and Jakarta, Indonesia
- Original Paper
- Published: 10 September 2020
- Volume 104 , pages 2461–2487, ( 2020 )
Cite this article
- Mohamed Kefi 1 , 5 ,
- Binaya Kumar Mishra 2 , 5 ,
- Yoshifumi Masago 3 , 5 &
- Kensuke Fukushi 4 , 5
2155 Accesses
10 Citations
Explore all metrics
The sustainability and efficiency of flood risk management depends on the assessment of flood hazards and on the quantification of flood damage. Under the conditions of climate change and rapid urbanization, the evaluation of flood risk can lead to the success of adaptation strategies. The main objectives of this study are the estimation of future direct flood damage in two urban watersheds: The Pasig–Marikina–San Juan River in Metro Manila, Philippines, and the Ciliwung River in Jakarta, Indonesia, as well as the determination of the relation between factors that drive floods and flood damage. A spatial analysis approach based on the integration of several parameters, such as flood hazard, climate, and property value, was applied using a Geographic Information System (GIS). The flood depth-damage function generated from the field surveys was employed for the analysis to identify the spatial distribution of flood loss. The findings showed that, under future scenarios (target year: 2030), the total flood damage will increase by 212% and 80% in the target areas of Manila and Jakarta, respectively, compared to the current scenarios. This growth is due to the higher level of extreme rainfall events and to the degree of urbanization in the future. A comparative analysis of the two study areas highlighted the significant effects of the level of water depth and the inundated areas on flood damage, depending on the sites. This study is useful for local decision makers to implement suitable strategies for urban planning and flood control.
This is a preview of subscription content, log in via an institution to check access.
Access this article
Price includes VAT (Russian Federation)
Instant access to the full article PDF.
Rent this article via DeepDyve
Institutional subscriptions
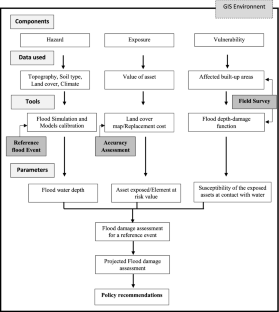
Similar content being viewed by others
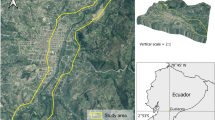
Assessment of microscale economic flood losses in urban and agricultural areas: case study of the Santa Bárbara River, Ecuador
Urban flood hazard assessment in the basin of athens metropolitan city, greece, assessment and mapping of flood potential in the slănic catchment in romania, data availability.
The data collected and used in this study are strictly anonymous and are used for research purposes only.
ADB (Asian Development Bank) (2013) The rise of natural disasters in Asia and the Pacific: learning from ADB’s experience. Independent Evaluation at ADB. ADB (Asian Development Bank), Mandaluyong
Google Scholar
ADB (Asian Development Bank) (2015) Global increase in climate-related disasters, working paper November 2015. Independent evaluation at ADB. ADB (Asian Development Bank), Metro Manila
Afifi Z, Chu HJ, Kuo YL, Hsu YC, Wong HK, Zeeshan Ali M (2019) Residential flood loss assessment and risk mapping from high-resolution simulation. Water 11:751. https://doi.org/10.3390/w11040751
Article Google Scholar
Albano R, Mancusi L, Sole A, Adamowski J (2015) Collaborative strategies for sustainable EU flood risk management: FOSS and geospatial tools—challenges and opportunities for operative risk analysis. ISPRS Int J Geo-Inf 4:2704–2727. https://doi.org/10.3390/ijgi4042704
Asdak C, Supian S, Subiyanto (2018) Watershed management strategies for flood mitigation: a case study of Jakarta’s flooding. Weather Clim Extrem 21:117–122. https://doi.org/10.1016/j.wace.2018.08.002
Bathrellos GD, Karymbalis E, Skilodimou HD, Gaki-Papanastassiou K, Baltas EA (2016) Urban flood hazard assessment in the basin of Athens Metropolitan city, Greece. Environ Earth Sci 75:319. https://doi.org/10.1007/s12665-015-5157-1
Campbell JB (2007) Introduction to remote sensing, 4th edn. The Guilford press, New York
Centre for Research on the Epidemiology of Disasters (2018) EM-DAT: the emergency events database. https://www.emdat.be . Accessed 03 May 2018
Chiba Y, Shaw R, Prabhakar S (2017) Climate change related non-economic loss and damage in Bangladesh and Japan. Int J Clim Change Strateg 9(2):166–183. https://doi.org/10.1108/IJCCSM-05-2016-0065
CTI Engineering International Co., Ltd and WCI, Woodfields Consultants, Inc. (2013) Master plan for flood management in Metro Manila and surrounding areas. Department of Public Works and Highways, the World Bank and AusAID, Tokyo
Dang NM, Babel MS, Luong HT (2011) Evaluation of flood risk parameters in the day river flood diversion area, Red River Delta, Vietnam. Nat Hazards 56(1):169–194. https://doi.org/10.1007/s11069-010-9558-x
De Moel H, Aerts JCJH (2011) Effect of uncertainty in land use, damage models and inundation depth on flood damage estimates. Nat Hazards 58(1):407–425. https://doi.org/10.1007/s11069-010-9675-6
Dutta D, Herath S, Musiake K (2003) A mathematic model for loss estimation. J Hydrol 227(1–2):24–49. https://doi.org/10.1016/S0022-1694(03)00084-2
Dutta D, Wright W, Rayment P (2011) Synthetic impact response functions for flood vulnerability analysis and adaptation measures in coastal zones under changing climatic conditions: a case study in Gippsland coastal region, Australia. Nat Hazards 59(2):967–986. https://doi.org/10.1007/s11069-011-9812-x
Fankhauser S, Dietz S, Gradwell P (2014) Non-economic losses in the context of the UNFCCC work programme on loss and damage (policy paper). Centre for Climate Change Economics and Policy, Grantham Research Institute on Climate Change and the Environment, London
Feldman AD (2000) Hydrologic modeling system HEC-HMS technical reference manual, U.S. Army Corps of Engineers, Hydrologic Engineering Center, HEC Davis, CA, USA
Fohrer N, Haverkamp S, Eckhardt K, Frede HG (2001) Hydrologic response to land use changes on the catchment scale. Phys Chem Earth Part B Hydrol Oceans Atmos 26(7–8):577–582. https://doi.org/10.1016/S1464-1909(01)00052-1
Foudi S, Oses-Eraso N, Tamayo I (2015) Integrated spatial flood risk assessment: the case of Zaragoza. Land Use Policy 42:278–292. https://doi.org/10.1016/j.landusepol.2014.08.002
Glas H, Jonckheere M, Mandal A, James-Williamson S, De Maeyer P, Deruyter G (2017) A GIS-based tool for flood damage assessment and delineation of a methodology for future risk assessment: case study for Annotto Bay, Jamaica. Nat Hazards 88:1867–1891. https://doi.org/10.1007/s11069-017-2920-5
Guha-Sapir D, Hoyois Ph, Wallemacq P, Below R (2016) Annual disaster statistical review 2016, the numbers and trends. CRED, Brussels
Handmer J (2002) The chimera of precision: inherent uncertainties in disaster loss assessment. Int J Mass Emerg Disaster 20(2):325–346
Huizinga J, De Moel H, Szewczyk W (2017). Global flood depth-damage functions. Methodology and the database with guidelines. EUR 28552 EN. https://doi.org/10.2760/16510
IPCC (Intergovernmental Panel on Climate Change) (2012) Managing the risks of extreme events and disasters to advance climate change adaptation. In: Field CBV, Barros TF, Stocker D, Qin DJ, Dokken KL, Ebi MD, Mastrandrea KJ, Mach GK, Plattner SK, Allen M et al (eds) A special report of Working Groups I and II of the Intergovernmental Panel on Climate Change. Cambridge University Press, Cambridge
Jalilov SM, Kefi M, Kumar P, Masago Y, Mishra BK (2018) Sustainable urban water management: application for integrated assessment in Southeast Asia. Sustainability 10(1):122. https://doi.org/10.3390/su10010122
Jongman B, Kreibich H, Barredo JI, Bates PD, Feyen L, Gericke A, Neal J, Aerts JCJH, Ward PJ (2012) Comparative flood damage model assessment: towards a European approach. Nat Hazard Earth Syst 12:3733–3752. https://doi.org/10.5194/nhess-12-3733-2012
Jonkman SN, Bockarjova M, Kok M, Bernardini P (2008) Integrated hydrodynamic and economic modelling of flood damage in the Netherlands. Ecol Econ 66(1):77–90. https://doi.org/10.1016/j.ecolecon.2007.12.022
Kefi M, Mishra BK, Kumar P, Masago Y, Fukushi K (2018) Assessment of tangible direct flood damage using a spatial analysis approach under the effects of climate change: case study in an urban watershed in Hanoi, Vietnam. ISPRS Int J Geo-Inf 7(1):29. https://doi.org/10.3390/ijgi7010029
Komolafe AA, Herath S, Avtar R (2015) Sensitivity of flood damage estimation to spatial resolution. J Flood Risk Manag. https://doi.org/10.1111/jfr3.12224
Komolafe AA, Herath S, Avtar R (2018) Development of generalized loss functions for rapid estimation of flood damages: a case study in Kelani River basin, Sri Lanka. Appl Geom 10(1):13–30. https://doi.org/10.1007/s12518-017-0200-4
Komori D, Nakamura S, Kiguchi M, Nishijima A, Yamazaki D, Suzuki S, Kawasaki A, Oki K, Oki T (2012) Characteristics of the 2011 Chao Phraya River flood in Central Thailand. Hydrol Res Lett 6:41–46. https://doi.org/10.3178/hrl.6.41
Kreibich H, Thieken AH (2008) Assessment of damage caused by high groundwater inundation. Water Resour Res 44:W09409. https://doi.org/10.1029/2007WR006621
Kundzewicz ZW, Kanae S, Seneviratne SI, Handmer J, Nicholls N, Peduzzi P, Mechler R, Bouwer LM, Arnell N, Mach K, Muir-Wood R, Brakenridge GR, Kron W, Benito G, Honda Y, Takahashi K, Sherstyukov B (2014) Flood risk and climate change: global and regional perspectives. Hydrol Sci J 59(1):1–28. https://doi.org/10.1080/02626667.2013.857411
Lagmay AM, Mendoza J, Cipriano F, Delmendo PA, Lacsamana MN, Moises MA, Pellejera NIII, Punay KN, Sabio G, Santos L, Serrano J, Taniza HJ, Tingin NE (2017) Street floods in Metro Manila and possible solutions. J Environ Sci 59:39–47. https://doi.org/10.1016/j.jes.2017.03.004
Lechowska E (2018) What determines flood risk perception? A review of factors of flood risk perception and relations between its basic elements. Nat Hazards 94:1341–1366. https://doi.org/10.1007/s11069-018-3480-z
Mall RK, Srivastava RK (2012) Sustainable flood management in changing climate. Mishra OP, Ghatak M, Kamal A (eds) SAARC workshop on flood risk management in South Asia FLOOD, 9–10 October 2012, Islamabad, Pakistan. Published by the SAARC Disaster Management Centre, New Delhi
Mardiah ANR, Lovett JC, Evanty N (2017) Toward integrated and inclusive disaster risk reduction in Indonesia: review of regulatory frameworks and institutional networks. In: Djalante R, Garschagen M, Thomalla F, Shaw R (eds) Disaster risk reduction in Indonesia: progress, challenges, and issues. Springer, Berlin
Merz B, Kreibich H, Schwarze R, Thieken A (2010) Assessment of economic flood damage. Nat Hazard Earth Syst 10:1697–1724. https://doi.org/10.5194/nhess-10-1697-2010
Messner F, Penning Rowsell E, Green C, Meyer V, Tunstall S, Vander Veen A (2007) Evaluating flood damages : guidance and recommendations on principles and methods; FLOOD site integrated flood risk analysis and management methodologies report T09-06-01; HR Wallingford: Oxfordshire, UK
Mishra B, Herath S (2011) Climate projections downscaling and impact assessment on precipitation over upper Bagmati River Basin. In: Proceedings of third international conference on addressing climate change for sustainable development through up-scaling renewable energy technologies. Tribhuvan University, Kathmandu, Nepal, 2011, pp 275–281
Mishra BK, Rafiei Emam A, Masago Y, Kumar P, Regmi RK, Fukushi K (2017) Assessment of future flood inundations under climate and land use change scenarios in the Ciliwung River Basin. J Flood Risk Manag, Jakarta. https://doi.org/10.1111/jfr3.12311
Book Google Scholar
Moss RH, Edmonds JA, Hibbard KA, Manning MR, Rose SK, Van Vuuren DP, Carter TR, Emori S, Kainuma M, Kram T, Meehl GA, Mitchell JF, Nakicenovic N, Riahi K, Smith SJ, Stouffer RJ, Thomson AM, Weyant JP, Wilbanks TJ (2010) The next generation of scenarios for climate change research and assessment. Nature 463(7282):747–756. https://doi.org/10.1038/nature08823
Nash JE, Sutcliffe JV (1970) River flow forecasting through conceptual models, part I: a discussion of principles. J Hydrol 10:282–290. https://doi.org/10.1016/0022-1694(70)90255-6
Neal JC, Bates PD, Fewtrell TJ, Hunter NM, Wilson MD, Horritt MS (2009) Distributed whole city water level measurements from the Carlisle 2005 urban flood event and comparison with hydraulic model simulations. J Hydrol 368:42–55. https://doi.org/10.1016/j.jhydrol.2009.01.026
Pathirage C, Seneviratne K, Amaratunga D, Haigh R (2014) Knowledge factors and associated challenges for successful disaster knowledge sharing. Input paper Prepared for the global assessment report on disaster risk reduction 2015. The United Nations Office for Disaster Risk Reduction (UNISDR), Global Assessment Report on Disaster Risk Reduction (GAR)
Pistrika A, Tsakiris G, Nalbantis I (2014) Flood depth-damage functions for built environment. Environ Process 1(4):553–572. https://doi.org/10.1007/s40710-014-0038-2
PSA (Philippine Statistics Authority) (2010) Census of population and housing Philippines, 2010. https://psa.gov.ph/sites/default/files/attachments/hsd/article/Table%201_2.pdf . Accessed 03 May 2018
PSA (Philippine Statistics Authority) (2015) Census of population. Republic of the Philippines, 2015. Accessed 03 May 2018
Rafiei Emam A, Mishra BK, Kumar P, Masago Y, Fukushi K (2016) Impact assessment of climate and land-use changes on flooding behavior in the Upper Ciliwung River, Jakarta, Indonesia. Water 8(12):559. https://doi.org/10.3390/w8120559
Richards JA, Jia X (2006) Remote sensing digital image analysis. An introduction fourth edition. Springer, Berlin
Serdeczny OM, Bauer S, Huq S (2018) Non-economic losses from climate change: opportunities for policy-oriented research. Climate Dev 10(2):97–101. https://doi.org/10.1080/17565529.2017.1372268
Smith DI (1994) Flood damage estimation—a review of urban stage-damage curves and loss functions. Water SA 20:231–238
Srinivasa Raju K, Nagesh Kumar D (2018) Impact of climate change on water resources with modeling techniques and case studies. Springer, Singapore
Stabinsky D, Singh H, Vaughan K, Champling R, Phillips J (2012) Tackling the limits to adaptation: an international framework to address “loss and damage” from climate change impacts. ActionAid. CARE International and WWF, Geneva
Te Linde AH, Bubeck P, Dekkers JEC, De Moel H, Aerts JCJH (2011) Future flood risk estimates along the river Rhine. Nat Hazard Earth Syst 11:459–473. https://doi.org/10.5194/nhess-11-459-2011
Thieken AH, Muller M, Kreibich H, Merz B (2005) Flood damage and influencing factors: new insights from the August 2002 flood in Germany. Water Resour Res 41:W12430. https://doi.org/10.1029/2005WR004177
UNDESA (United Nations, Department of Economic and Social Affairs) (2015) Population division world urbanization prospects: the 2014 revision (ST/ESA/SER.A/366)
Win S, Zin W, Kawasaki W, San Z (2018) Establishment of flood damage function MODELS: a case study in the Bago River Basin, Myanmar. Int J Disast Risk Reduct 28:688–700. https://doi.org/10.1016/j.ijdrr.2018.01.030
Zischg AP, Hofer P, Mosimann M, Röthlisberger V, Ramirez JA, Keiler M, Weingartner R (2018) Flood risk (d)evolution: disentangling key drivers of flood risk change with a retro-model experiment. Sci Total Environ 639:195–207. https://doi.org/10.1016/j.scitotenv.2018.05.056
Download references
Acknowledgements
The authors are grateful to the staff of Center of Environmental Research, Research and Community Services Institute, Bogor Agricultural University, Indonesia (PPLH –IPB), and local residents and local NGO in Metro Manila, Philippines, for their cooperation during the field survey.
This research was supported by the Japan Society for the Promotion of Science as Overseas researcher under Postdoctoral Fellowship of JSPS (Fellowship P16790). This work was also supported by the Water and Urban Initiative project of the United Nations University Institute for the Advanced Study of Sustainability (UNU-IAS), Tokyo, Japan.
Author information
Authors and affiliations.
Laboratory of Desalination and Natural Water Valorisation, Water Research and Technologies Center CERTE-Technopark of Borj Cedria, BP 273, 8020, Soliman, Tunisia
Mohamed Kefi
School of Engineering, Faculty of Science and Technology, Pokhara University, Pokhara-30, Kaski, Nepal
Binaya Kumar Mishra
Center for Climate Change Adaptation, National Institute for Environmental Studies, Tsukuba, Ibaraki, 305-8506, Japan
Yoshifumi Masago
Institute for Future Initiatives, The University of Tokyo, 7-3-1 Hongo, Bunkyo-ku, Tokyo, 113-0033, Japan
Kensuke Fukushi
United Nations University Institute for the Advanced Study of Sustainability, 5-53-70 Jingumae, Shibuya-ku, Tokyo, 150-8925, Japan
Mohamed Kefi, Binaya Kumar Mishra, Yoshifumi Masago & Kensuke Fukushi
You can also search for this author in PubMed Google Scholar
Corresponding author
Correspondence to Mohamed Kefi .
Ethics declarations
Conflict of interest.
The authors declare that they have no conflict of interest.
Additional information
Publisher's note.
Springer Nature remains neutral with regard to jurisdictional claims in published maps and institutional affiliations.
Electronic supplementary material
Below is the link to the electronic supplementary material.
Questionnaire about flood event in Jakarta
Rights and permissions.
Reprints and permissions
About this article
Kefi, M., Mishra, B.K., Masago, Y. et al. Analysis of flood damage and influencing factors in urban catchments: case studies in Manila, Philippines, and Jakarta, Indonesia. Nat Hazards 104 , 2461–2487 (2020). https://doi.org/10.1007/s11069-020-04281-5
Download citation
Received : 18 January 2020
Accepted : 29 August 2020
Published : 10 September 2020
Issue Date : December 2020
DOI : https://doi.org/10.1007/s11069-020-04281-5
Share this article
Anyone you share the following link with will be able to read this content:
Sorry, a shareable link is not currently available for this article.
Provided by the Springer Nature SharedIt content-sharing initiative
- Flood damage
- Flood depth-damage function
- Climate change
- Urbanization
- Find a journal
- Publish with us
- Track your research

IMAGES
COMMENTS
The study area consists of three inland US cities—Atlanta, Phoenix, and Portland—that are prone to pluvial flooding. These cities are diverse in terms of physical and social characteristics (Table 1 and Fig. 2), providing scope for comparative analysis.All cities in this study are growing in population (U.S. Census Bureau, 2020) and have long histories of pluvial flooding, with major ...
Based on information gathered from the case study cities, the committee will produce a consensus report that: 1. Identifies any commonalities and variances among the case study metropolitan areas in terms of causes, adverse impacts, unexpected problems in recovery, or effective mitigation strategies, as well as key themes of urban flooding. 2.
This floodplain analysis provides a portal for assessing a city's performance in terms of flood resiliency in transportation, and provides urban planners, engineers, and political decision makers with insights into interrelationships between infrastructure and institutional resilience. 5. Institution and policy review.
Urban flooding occurred even in some mega cities and appeared to be transitioning into a chronic problem. In the capital city of Beijing, for example, urban flooding with casualties and/or injuries had occurred at least seven times over the past two decades: in 2004 (twice), 2007, 2011 (twice), 2012, and most recently on August 16, 2021 (Table 1).
data on urban flood hazard, flooding at local scales, or the economic costs and social impacts of flooding. Without better ... risk assessments for the case study areas found that 2.8 million people are exposed to flooding, more than triple the number estimated by FEMA. In addition, studies estimate that average annual losses are $3.3 billion ...
These flood data have been validated in detailed case studies, including for the USA, Canada and the UK 34,37, as well as a validation study for Nigeria and Mozambique 38, which finds accuracy ...
Urban flooding has become a national challenge in recent years due to a variety of socio-economic and environmental changes alongside rapid land use change in flood-prone areas. Losses from acute and chronic floods have become especially problematic in low-lying urban areas, where stormwater infrastructure deterioration, population growth, and ...
1. Introduction. Urban flooding is mostly pluvial flooding caused by High-Intensity Short Duration (HI-SD) rainfall. when ground infiltration and storm drainage are not capable to take away the ...
Indeed, the United States (U.S.) Department of Energy has dedicated $66 million towards combating climate change-induced extreme events, such as urban flooding [198], and the National Academy of Sciences organized an ad hoc committee of scientists to conduct case studies and explore urban flood occurrences [128]. The urgency of research is ...
This study focuses on urban floods occurring independently and/or in conjunction with flash and river floods. As at the year 2000, global urban land susceptible to floods was estimated to be around 195,493 km 2 (Güneralp et al. 2015). Africa is one of the continents highly affected by flood-induced disasters, particularly in urban areas.
This study has examined aspects related to urban flooding in the study area. The main aspects are daily life challenges, vulnerability from the flooding, and the local future resilience towards floods. ... Rufat S, Tate E, Burton CG, Maroof Abu Sayeed (2015) Social vulnerability to floods: Review of case studies and implications for measurement ...
For the Chicago case study, the committee convened a workshop in Chicago, Illinois, followed by site visits to three Chicago locations. Additional information was collected from some participants via telephone interviews. The workshop was structured to gather information from local, state, regional, and federal stakeholders.
Community resilience is a key index for describing the response of human habitat systems to hazards. Evaluating and enhancing the community resilience requires indicators, identification, and quantitative measurements, especially for urban flooding management. In this study, an advanced index framework for measuring community resilience to urban flooding is proposed, integrating the fuzzy ...
A history of destructive floods. The new study came about through collaboration with regional planners and residents in bayside cities including East Palo Alto, which faces rising flood risks from ...
A Research through Designing approach was used to explore nature-based solutions (NbS) for flood management at the fluvial (regional) and pluvial (local) scales as part of a Smart District visioning study in a peri-urban area north of Bangkok, Thailand. The NbS visions were informed by community surveys (total n = 770) as well as in-depth, semi-structured interviews with community leaders and ...
Johnson, B. A. et al. High-resolution urban change modeling and flood exposure estimation at a national scale using open geospatial data: A case study of the Philippines. Comput. Environ.
Urban flooding events are becoming increasingly frequent and serious in urban coastal areas (Arnell & Gosling, 2016; Cannaby, Fach, Arkin, ... This study uses the case of Shenzhen City to explore the spatial patterns between vulnerability and resilience from the perspective of spatial balance. Results reveal that the spatial inequality of ...
Using hydraulic models for two South Asian cities, Sylhet (in Bangladesh) and Bharatpur (in Nepal), we find that 22.3% of the land area in Sylhet and 12.7% in Bharatpur is under flooding risk, under the current scenario. The flood risk area can be reduced to 3.6% in Sylhet and 5.5% in Bharatpur with structural interventions in the drainage system.
Abstract and Figures. Urbanization in developing countries doubled from less than 25% in 1970 to more than 50% in 2006. It is widely accepted that proactive disaster management is a necessity for ...
Urban floods often affect certain parts of the city more adversely. As such, a local area level perspective is needed in the study of urban floods and mobility disruptions. The mobility of individuals and groups in a space-time framework have been the subject of interest of urban planners (Song et al. 2010; Simini et al. 2012).
Bangkok flood is an obstacle blocking the development of the city and its citizen's well-being as Bangkok. is situated on flood-sensitive area; therefore, Bangkok is flooded repeatedly. Measures ...
Model simulates urban flood risk with an eye to equity. by Tara Roberts, Stanford University. Flood waters from the San Francisquito Creek reached apartment complexes in East Palo Alto, California ...
Urban flooding is the inundation of land or property in cities or other ... Several studies have looked into the flow patterns and redistribution in streets during storm events and the implication on flood modelling. ... Dry soils can also become harder. In this case heavy rainfall runs off into rivers and lakes. This increases risks of ...
Therefore, in this study, the 7.20 Henan Extreme Flood event was selected as a typical case of sudden urban flooding. Using the 'Weibo' platform, which is widely used in China, we extracted a dataset of highly relevant microblogs. The study period was divided according to the response relationship between rainfall and the number of microblogs.
URBAN FLOODING - CASE STUDY OF HYDERABAD. Urban flooding is significantly different from rural flooding as urbanisation leads to developed catchments which increases the flood peaks from 1.8 to 8 times and flood volumes by up to 6 times. Consequently, flooding occurs very quickly due to faster flow times, sometimes in a matter of minutes.
Drainage modeling that accurately captures urban storm inundation serves as the foundation for flood warning and drainage scheduling. In this paper, we proposed a novel coupling ideology that, by integrating 2D-1D and 1D-2D unidirectional processes, overcomes the drawback of the conventional unidirectional coupling approach that fails to properly represent the rainfall surface catchment ...
In this study, urban flood hazard and flood damages were performed in GIS environment. A flood depth-damage function was established to evaluate flood damage through data collected from the field survey. ... For both case studies, the flood depth-damage function was generated based on the data collected through field survey in both areas. 2.3.3 ...
Urban pluvial flooding: A qualitative case study of cause, effect and nonstructural mitigation J. Flood Risk Manag. , 3 ( 2010 ) , pp. 112 - 125 , 10.1111/j.1753-318X.2010.01061.x View in Scopus Google Scholar
Flood vulnerability assessment (FVA) informs the disaster risk reduction and preparedness process in both rural and urban areas. However, many flood-vulnerable regions like Malawi still lack FVA supporting frameworks in all phases (pre-trans-post disaster). Partly, this is attributed to lack of the evidence-based studies to inform the processes. This study was therefore aimed at assessing ...