Towards Sustainable Energy: A Systematic Review of Renewable Energy Sources, Technologies, and Public Opinions
Ieee account.
- Change Username/Password
- Update Address

Purchase Details
- Payment Options
- Order History
- View Purchased Documents
Profile Information
- Communications Preferences
- Profession and Education
- Technical Interests
- US & Canada: +1 800 678 4333
- Worldwide: +1 732 981 0060
- Contact & Support
- About IEEE Xplore
- Accessibility
- Terms of Use
- Nondiscrimination Policy
- Privacy & Opting Out of Cookies
A not-for-profit organization, IEEE is the world's largest technical professional organization dedicated to advancing technology for the benefit of humanity. © Copyright 2024 IEEE - All rights reserved. Use of this web site signifies your agreement to the terms and conditions.
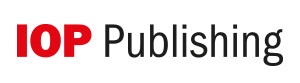
We apologize for the inconvenience...
To ensure we keep this website safe, please can you confirm you are a human by ticking the box below.
If you are unable to complete the above request please contact us using the below link, providing a screenshot of your experience.
https://ioppublishing.org/contacts/
Thank you for visiting nature.com. You are using a browser version with limited support for CSS. To obtain the best experience, we recommend you use a more up to date browser (or turn off compatibility mode in Internet Explorer). In the meantime, to ensure continued support, we are displaying the site without styles and JavaScript.
- View all journals
- Explore content
- About the journal
- Publish with us
- Sign up for alerts
- Published: 03 August 2020
Impacts of climate change on energy systems in global and regional scenarios
- Seleshi G. Yalew ORCID: orcid.org/0000-0002-7304-6750 1 , 2 , 3 ,
- Michelle T. H. van Vliet 2 , 4 ,
- David E. H. J. Gernaat ORCID: orcid.org/0000-0003-4994-1453 1 , 5 ,
- Fulco Ludwig 2 ,
- Ariel Miara ORCID: orcid.org/0000-0001-7089-4765 6 , 7 ,
- Chan Park ORCID: orcid.org/0000-0002-4994-6855 8 ,
- Edward Byers ORCID: orcid.org/0000-0003-0349-5742 9 ,
- Enrica De Cian 10 , 11 ,
- Franziska Piontek 12 ,
- Gokul Iyer ORCID: orcid.org/0000-0002-3565-7526 13 ,
- Ioanna Mouratiadou ORCID: orcid.org/0000-0002-3541-6271 1 ,
- James Glynn ORCID: orcid.org/0000-0001-7004-0153 14 ,
- Mohamad Hejazi 13 ,
- Olivier Dessens 15 ,
- Pedro Rochedo ORCID: orcid.org/0000-0001-5151-0893 16 ,
- Robert Pietzcker ORCID: orcid.org/0000-0002-9403-6711 12 ,
- Roberto Schaeffer ORCID: orcid.org/0000-0002-3709-7323 16 ,
- Shinichiro Fujimori ORCID: orcid.org/0000-0001-7897-1796 17 , 18 ,
- Shouro Dasgupta ORCID: orcid.org/0000-0003-4080-8066 10 , 11 ,
- Silvana Mima 19 ,
- Silvia R. Santos da Silva ORCID: orcid.org/0000-0002-6493-1475 13 , 20 ,
- Vaibhav Chaturvedi 21 ,
- Robert Vautard ORCID: orcid.org/0000-0001-5544-9903 22 &
- Detlef P. van Vuuren ORCID: orcid.org/0000-0003-0398-2831 1 , 5
Nature Energy volume 5 , pages 794–802 ( 2020 ) Cite this article
12k Accesses
186 Citations
128 Altmetric
Metrics details
- Projection and prediction
Although our knowledge of climate change impacts on energy systems has increased substantially over the past few decades, there remains a lack of comprehensive overview of impacts across spatial scales. Here, we analyse results of 220 studies projecting climate impacts on energy systems globally and at the regional scale. Globally, a potential increase in cooling demand and decrease in heating demand can be anticipated, in contrast to slight decreases in hydropower and thermal energy capacity. Impacts at the regional scale are more mixed and relatively uncertain across regions, but strongest impacts are reported for South Asia and Latin America. Our assessment shows that climate impacts on energy systems at regional and global scales are uncertain due partly to the wide range of methods and non-harmonized datasets used. For a comprehensive assessment of climate impacts on energy, we propose a consistent multi-model assessment framework to support regional-to-global-scale energy planning.
This is a preview of subscription content, access via your institution
Access options
Access Nature and 54 other Nature Portfolio journals
Get Nature+, our best-value online-access subscription
24,99 € / 30 days
cancel any time
Subscribe to this journal
Receive 12 digital issues and online access to articles
111,21 € per year
only 9,27 € per issue
Buy this article
- Purchase on Springer Link
- Instant access to full article PDF
Prices may be subject to local taxes which are calculated during checkout
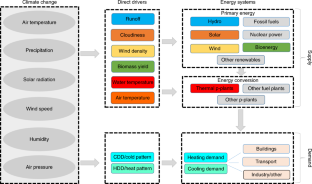
Similar content being viewed by others
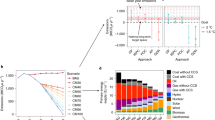
A framework for national scenarios with varying emission reductions
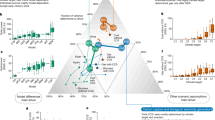
Spread in climate policy scenarios unravelled
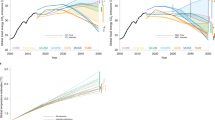
A multi-model analysis of long-term emissions and warming implications of current mitigation efforts
Data availability.
All data that support the findings of this study presented in the figures are provided in the Source Data section associated with this manuscript. Source data are provided with this paper.
Bruckner, T. et al. in Climate Change 2014: Mitigation of Climate Change (eds Edenhofer, O. et al.) (Cambridge Univ. Press, 2014).
Schaeffer, R. et al. Energy sector vulnerability to climate change: a review. Energy 38 , 1–12 (2012).
Google Scholar
Ebinger, J. & Vergara, W. Climate Impacts on Energy Systems: Key Issues for Energy Sector Adaptation (The World Bank, 2011).
Crook, J. A., Jones, L. A., Forster, P. M. & Crook, R. Climate change impacts on future photovoltaic and concentrated solar power energy output. Energ. Environ, Sci. 4 , 3101–3109 (2011).
Owusu, P. A. & Asumadu-Sarkodie, S. A review of renewable energy sources, sustainability issues and climate change mitigation. Cogent. Eng. 3 , 1167990 (2016).
Bartos, M. D. & Chester, M. V. Impacts of climate change on electric power supply in the Western United States. Nat. Clim. Change 5 , 748–752 (2015).
Karnauskas, K. B., Lundquist, J. K. & Zhang, L. Southward shift of the global wind energy resource under high carbon dioxide emissions. Nar. Geosci. 11 , 38–43 (2018).
Craig, M. T. et al. A review of the potential impacts of climate change on bulk power system planning and operations in the United States. Renew. Sust. Energ. Rev. 98 , 255–267 (2018).
Van Vliet, M. T. et al. Vulnerability of US and European electricity supply to climate change. Nat. Clim. Change 2 , 676 (2012).
Liu, L., Hejazi, M., Li, H., Forman, B. & Zhang, X. Vulnerability of US thermoelectric power generation to climate change when incorporating state-level environmental regulations. Nat. Ener. 2 , 17109 (2017).
Ciscar, J.-C. & Dowling, P. Integrated assessment of climate impacts and adaptation in the energy sector. Energy Econ. 46 , 531–538 (2014).
Ravestein, P., van der Schrier, G., Haarsma, R., Scheele, R. & van den Broek, M. Vulnerability of European intermittent renewable energy supply to climate change and climate variability. Renew. Sust. Ener. Rev. 97 , 497–508 (2018).
Perera, A., Nik, V. M., Chen, D., Scartezzini, J. L. & Hong, T. Quantifying the impacts of climate change and extreme climate events on energy systems. Nat. Energy 5 , 150–159 (2020).
IPCC Climate Change 2014: Synthesis Report (eds Core Writing Team, Pachauri, R. K. & Meyer L. A.) (IPCC, 2014).
Isaac, M. & van Vuuren, D. P. Modeling global residential sector energy demand for heating and air conditioning in the context of climate change. Energy Policy 37 , 507–521 (2009).
IPCC Climate Change 2014: Impacts, Adaptation, and Vulnerability (eds Field, C. B. et al.) (Cambridge Univ. Press, 2014).
Cinner, J. E. et al. Building adaptive capacity to climate change in tropical coastal communities. Nat. Clim. Change 8 , 177–123 (2018).
Lumbroso, D., Woolhouse, G. & Jones, L. A review of the consideration of climate change in the planning of hydropower schemes in sub-Saharan Africa. Clim. Change 133 , 621–633 (2015).
Kabir, E., Kumar, P., Kumar, S., Adelodun, A. A. & Kim, K.-H. Solar energy: potential and future prospects. Renew. Sust. Energ. Rev. 82 , 894–900 (2018).
Pryor, S. & Barthelmie, R. Climate change impacts on wind energy: a review. Renew. Sust. Energ. Rev. 14 , 430–437 (2010).
Berndes, G., Hoogwijk, M. & Van den Broek, R. The contribution of biomass in the future global energy supply: a review of 17 studies. Biomass Bioenerg. 25 , 1–28 (2003).
Li, D. H., Yang, L. & Lam, J. C. Impact of climate change on energy use in the built environment in different climate zones–a review. Energy 42 , 103–112 (2012).
Auffhammer, M. & Mansur, E. T. Measuring climatic impacts on energy consumption: a review of the empirical literature. Energy Econ. 46 , 522–530 (2014).
Mideksa, T. K. & Kallbekken, S. The impact of climate change on the electricity market: a review. Energy Policy 38 , 3579–3585 (2010).
Chandramowli, S. N. & Felder, F. A. Impact of climate change on electricity systems and markets–a review of models and forecasts. Sust. Energ. Technol. Assess. 5 , 62–74 (2014).
Mikellidou, C. V., Shakou, L. M., Boustras, G. & Dimopoulos, C. Energy critical infrastructures at risk from climate change: a state of the art review. Safety Science 110 , https://doi.org/10.1016/j.ssci.2017.12.022 (2017).
Stanton, M. C. B., Dessai, S. & Paavola, J. A systematic review of the impacts of climate variability and change on electricity systems in Europe. Energy 109 , 1148–1159 (2016).
Cronin, J., Anandarajah, G. & Dessens, O. Climate change impacts on the energy system: a review of trends and gaps. Clim. Change 151 , 79–93 (2018).
Barnett, T. P., Adam, J. C. & Lettenmaier, D. P. Potential impacts of a warming climate on water availability in snow-dominated regions. Nature 438 , 303 (2005).
Chilkoti, V., Bolisetti, T. & Balachandar, R. Climate change impact assessment on hydropower generation using multi-model climate ensemble. Renew. Energ. 109 , 510–517 (2017).
Fan, J.-L. Impacts of climate change on hydropower generation in China. Comput. Simulat. 167 , 4–18 (2018).
MathSciNet Google Scholar
Teotónio, C., Fortes, P., Roebeling, P., Rodriguez, M. & Robaina-Alves, M. Assessing the impacts of climate change on hydropower generation and the power sector in Portugal: a partial equilibrium approach. Renew. Sust. Energ. Rev. 74 , 788–799 (2017).
Hamududu, B. & Killingtveit, A. Assessing climate change impacts on global hydropower. Energies 5 , 305–322 (2012).
Van Vliet, M. T., Wiberg, D., Leduc, S. & Riahi, K. Power-generation system vulnerability and adaptation to changes in climate and water resources. Nat. Clim. Change 6 , 375–380 (2016).
Van Vliet, M. et al. Multi-model assessment of global hydropower and cooling water discharge potential under climate change. Global Environ. Change 40 , 156–170 (2016).
Turner, S. W., Ng, J. Y. & Galelli, S. Examining global electricity supply vulnerability to climate change using a high-fidelity hydropower dam model. Sci. Total Environ. 590 , 663–675 (2017).
Zhou, Y. et al. A comprehensive view of global potential for hydro-generated electricity. Energ. Environ. Sci. 8 , 2622–2633 (2015).
Raje, D. & Mujumdar, P. Reservoir performance under uncertainty in hydrologic impacts of climate change. Adv. Water Resour. 33 , 312–326 (2010).
Gaudard, L., Gilli, M. & Romerio, F. Climate change impacts on hydropower management. Water Resource. Manag. 27 , 5143–5156 (2013).
Mohor, G. S., Rodriguez, D. A., Tomasella, J. & Júnior, J. L. S. Exploratory analyses for the assessment of climate change impacts on the energy production in an Amazon run-of-river hydropower plant. J. Hydrol. Reg. Studies 4 , 41–59 (2015).
Fant, C., Schlosser, C. A. & Strzepek, K. The impact of climate change on wind and solar resources in southern Africa. Appl. Energy 161 , 556–564 (2016).
Wachsmuth, J. et al. How will renewable power generation be affected by climate change? The case of a Metropolitan Region in Northwest Germany. Energy 58 , 192–201 (2013).
Jerez, S. et al. The impact of climate change on photovoltaic power generation in Europe. Nat. Commun. 6 , 10014 (2015).
Bartók, B. et al. Projected changes in surface solar radiation in CMIP5 global climate models and in EURO-CORDEX regional climate models for Europe. Climate Dynam. 49 , 2665–2683 (2017).
Davy, R., Gnatiuk, N., Pettersson, L. & Bobylev, L. Climate change impacts on wind energy potential in the European domain with a focus on the Black Sea. Renew. Sust. Energ. Rev. 81 , 1652–1659 (2017).
Carvalho, D., Rocha, A., Gómez-Gesteira, M. & Santos, C. S. Potential impacts of climate change on European wind energy resource under the CMIP5 future climate projections. Renew. Energ. 101 , 29–40 (2017).
Hueging, H., Haas, R., Born, K., Jacob, D. & Pinto, J. G. Regional changes in wind energy potential over Europe using regional climate model ensemble projections. J. Appl. Meteorol. Climatol. 52 , 903–917 (2013).
Tobin, I. et al. Vulnerabilities and resilience of European power generation to 1.5 °C, 2 °C and 3 °C warming. Environ. Res. Lett. 13 , 044024 (2018).
Vautard, R. et al. Regional climate model simulations indicate limited climatic impacts by operational and planned European wind farms. Nat. Commun. 5 , 3196 (2014).
Jerez, S. et al. Future changes, or lack thereof, in the temporal variability of the combined wind-plus-solar power production in Europe. Renew. Energy 139 , 251–260 (2019).
De Lucena, A. F. P., Szklo, A. S., Schaeffer, R. & Dutra, R. M. The vulnerability of wind power to climate change in Brazil. Renew. Energy 35 , 904–912 (2010).
Pereira, E. B., Martins, F. R., Pes, M. P., da Cruz Segundo, E. I. & Lyra, Ad. A. The impacts of global climate changes on the wind power density in Brazil. Renew. Energy 49 , 107–110 (2013).
Breslow, P. B. & Sailor, D. J. Vulnerability of wind power resources to climate change in the continental United States. Renew. Energy 27 , 585–598 (2002).
Sailor, D. J., Smith, M. & Hart, M. Climate change implications for wind power resources in the Northwest United States. Renew. Energy 33 , 2393–2406 (2008).
De Jong, P. et al. Estimating the impact of climate change on wind and solar energy in Brazil using a South American regional climate model. Renew. Energy 141 , 390–401 (2019).
Tuck, G., Glendining, M. J., Smith, P., House, J. I. & Wattenbach, M. The potential distribution of bioenergy crops in Europe under present and future climate. Biomass Bioenerg. 30 , 183–197 (2006).
Bellarby, J., Wattenbach, M., Tuck, G., Glendining, M. J. & Smith, P. The potential distribution of bioenergy crops in the UK under present and future climate. Biomass Bioenerg. 34 , 1935–1945 (2010).
Harvey, M. & Pilgrim, S. The new competition for land: food, energy, and climate change. Food Policy 36 , S40–S51 (2011).
Kyle, P., Müller, C., Calvin, K. & Thomson, A. Meeting the radiative forcing targets of the representative concentration pathways in a world with agricultural climate impacts. Earth’s Future 2 , 83–98 (2014).
Miara, A., Vörösmarty, C. J., Stewart, R. J., Wollheim, W. M. & Rosenzweig, B. Riverine ecosystem services and the thermoelectric sector: strategic issues facing the Northeastern United States. Environ. Res. Lett. 8 , 025017 (2013).
Miara, A. et al. Climate and water resource change impacts and adaptation potential for US power supply. Nat. Clim. Change 7 , 793–798 (2017).
Miara, A. et al. Climate-water adaptation for future US electricity infrastructure. Environ. Sci. Technol. 53 , 14029–14040 (2019).
Byers, E., Hall, J., Amezaga, J., O’Donnell, G. & Leathard, A. Water and climate risks to power generation with carbon capture and storage. Environ. Res. Lett. 11 , 024011 (2016).
Angeles, M. E., González, J. E. & Ramírez, N. Impacts of climate change on building energy demands in the intra-Americas region. Theoret. Appl. Climatol 133 , 59–72 (2018).
Fan, J.-L., Hu, J.-W. & Zhang, X. Impacts of climate change on electricity demand in China: an empirical estimation based on panel data. Energy 170 , 880–888 (2019).
Taseska, V., Markovska, N. & Callaway, J. M. Evaluation of climate change impacts on energy demand. Energy 48 , 88–95 (2012).
Allen, M. R., Fernandez, S. J., Fu, J. S. & Olama, M. M. Impacts of climate change on sub-regional electricity demand and distribution in the southern United States. Nat. Energy 1 , 16103 (2016).
Zhou, Y. et al. Modeling the effect of climate change on US state-level buildings energy demands in an integrated assessment framework. Appl. Energy 113 , 1077–1088 (2014).
Hadley, S. W., Erickson Iii, D. J., Hernandez, J. L., Broniak, C. T. & Blasing, T. J. Responses of energy use to climate change: a climate modeling study. Geophys. Res. Lett. 33 , https://doi.org/10.1029/2006GL026652 (2006).
Eom, J., Clarke, L., Kim, S. H., Kyle, P. & Patel, P. China’s building energy demand: long-term implications from a detailed assessment. Energy 46 , 405–419 (2012).
McFarland, J. et al. Impacts of rising air temperatures and emissions mitigation on electricity demand and supply in the United States: a multi-model comparison. Clim. Change 131 , 111–125 (2015).
Clarke, L. et al. Effects of long-term climate change on global building energy expenditures. Energy Econ. 72 , 667–677 (2018).
Labriet, M. et al. Worldwide impacts of climate change on energy for heating and cooling. Mit. Adapt. Strat. Global Change 20 , 1111–1136 (2015).
Van Ruijven, B. J., De Cian, E. & Wing, I. S. Amplification of future energy demand growth due to climate change. Nat. Commun. 10 , 2762 (2019).
De Cian, E. & Wing, I. S. Global energy consumption in a warming climate. Environ. Res. Econ. 72 , 365–410 (2019).
Auffhammer, M., Baylis, P. & Hausman, C. H. Climate change is projected to have severe impacts on the frequency and intensity of peak electricity demand across the United States. Proc. Natl Acad. Sci. USA 114 , 1886–1891 (2017).
De Cian, E., Lanzi, E. & Roson, R. Seasonal temperature variations and energy demand. Clim. Change 116 , 805–825 (2013).
Invidiata, A. & Ghisi, E. Impact of climate change on heating and cooling energy demand in houses in Brazil. Energ. Buildings 130 , 20–32 (2016).
Wang, H. & Chen, Q. Impact of climate change heating and cooling energy use in buildings in the United States. Energ. Buildings 82 , 428–436 (2014).
Hamlet, A. F., Lee, S.-Y., Mickelson, K. E. & Elsner, M. M. Effects of projected climate change on energy supply and demand in the Pacific Northwest and Washington State. Clim. Change 102 , 103–128 (2010).
Davis, L. W. & Gertler, P. J. Contribution of air conditioning adoption to future energy use under global warming. Proc. Natl Acad. Sci. USA 112 , 5962–5967 (2015).
Park, C. et al. Avoided economic impacts of energy demand changes by 1.5 and 2 °C climate stabilization. Environ. Res. Lett. 13 , 045010 (2018).
Waite, M. et al. Global trends in urban electricity demands for cooling and heating. Energy 127 , 786–802 (2017).
Morakinyo, T. E. et al. Estimates of the impact of extreme heat events on cooling energy demand in Hong Kong. Renew. Energy 142 , 73–84 (2019).
Moazami, A., Nik, V. M., Carlucci, S. & Geving, S. Impacts of future weather data typology on building energy performance–Investigating long-term patterns of climate change and extreme weather conditions. Appl. Energy 238 , 696–720 (2019).
Dirks, J. A. et al. Impacts of climate change on energy consumption and peak demand in buildings: a detailed regional approach. Energy 79 , 20–32 (2015).
D’Oca, S., Hong, T. & Langevin, J. The human dimensions of energy use in buildings: a review. Renew. Sust. Energy Rev. 81 , 731–742 (2018).
Poortinga, W., Steg, L. & Vlek, C. Values, environmental concern, and environmental behavior: a study into household energy use. Environ. Behav. 36 , 70–93 (2004).
De Cian, E., Pavanello, F., Randazzo, T., Mistry, M. N. & Davide, M. Households’ adaptation in a warming climate. Air conditioning and thermal insulation choices. Environ. Sci. Policy 100 , 136–157 (2019).
Castleton, H. F., Stovin, V., Beck, S. B. & Davison, J. B. Green roofs; building energy savings and the potential for retrofit. Energy Buildings 42 , 1582–1591 (2010).
Jones, P., Lannon, S. & Patterson, J. Retrofitting existing housing: how far, how much? Building Res. Info. 41 , 532–550 (2013).
Da Silva Soito, J. L. & Freitas, M. A. V. J. R. Amazon and the expansion of hydropower in Brazil: vulnerability, impacts and possibilities for adaptation to global climate change. Renew. Sust. Energy Rev. 15 , 3165–3177 (2011).
Cohen, S. M., Macknick, J., Averyt, K. & Meldrum, J. Modeling Climate-Water Impacts on Electricity Sector Capacity Expansion (National Renewable Energy Laboratory, 2014).
Mima, S. & Criqui, P. The costs of climate change for the European energy system, an assessment with the POLES model. Environ. Model. Ass. 20 , 303–319 (2015).
Ackerman, F. & Stanton, E. A. The Cost of Climate Change: What We’ll Pay if Global Warming Continues Unchecked (Natural Resources Defence Council, 2008).
Turner, S. W., Hejazi, M., Kim, S. H., Clarke, L. & Edmonds, J. Climate impacts on hydropower and consequences for global electricity supply investment needs. Energy 141 , 2081–2090 (2017).
Van der Linden, P. & Mitchell, J. (eds) ENSEMBLES: Climate Change and its Impacts—Summary of Research and Results from the ENSEMBLES Project (European Environment Agency, 2009).
Rübbelke, D. & Vögele, S. Impacts of climate change on European critical infrastructures: the case of the power sector. Environ. Sci. Policy 14 , 53–63 (2011).
Pryor, S. & Barthelmie, R. Assessing the vulnerability of wind energy to climate change and extreme events. Clim. Change 121 , 79–91 (2013).
Miller, N. L., Hayhoe, K., Jin, J. & Auffhammer, M. Climate, extreme heat, and electricity demand in California. J. Appl. Meteorol. Climatol. 47 , 1834–1844 (2008).
Forzieri, G. et al. Escalating impacts of climate extremes on critical infrastructures in Europe. Global Environ. Change 48 , 97–107 (2018).
Bartos, M. et al. Impacts of rising air temperatures on electric transmission ampacity and peak electricity load in the United States. Environ. Res. Lett. 11 , 114008 (2016).
Panteli, M. & Mancarella, P. Influence of extreme weather and climate change on the resilience of power systems: Impacts and possible mitigation strategies. Electric Power Syst. Res. 127 , 259–270 (2015).
Dowling, P. The impact of climate change on the European energy system. Energy Policy 60 , 406–417 (2013).
Haddeland, I. et al. Multimodel estimate of the global terrestrial water balance: setup and first results. J. Hydrometeorol. 12 , 869–884 (2011).
Schewe, J. et al. Multimodel assessment of water scarcity under climate change. Proc. Natl Acad. Sci. USA 111 , 3245–3250 (2014).
Rosenzweig, C. et al. The agricultural model intercomparison and improvement project (AgMIP): protocols and pilot studies. Agri. Forest Meteorol. 170 , 166–182 (2013).
van Vuuren, D. P. & Carter, T. R. Climate and socio-economic scenarios for climate change research and assessment: reconciling the new with the old. Clim. Change 122 , 415–429 (2014).
Van Vuuren, D. P. et al. A new scenario framework for climate change research: scenario matrix architecture. Clim. Change 122 , 373–386 (2014).
O’Neill, B. C. et al. A new scenario framework for climate change research: the concept of shared socioeconomic pathways. Clim. Change 122 , 387–400 (2014).
Wiedenhofer, D., Lenzen, M. & Steinberger, J. K. Energy requirements of consumption: urban form, climatic and socio-economic factors, rebounds and their policy implications. Energy Policy 63 , 696–707 (2013).
Frieler, K. et al. Assessing the impacts of 1.5 C global warming–simulation protocol of the Inter-Sectoral Impact Model Intercomparison Project (ISIMIP2b). Geosci. Model Dev. 10 , 4321–4345 (2017).
Download references
Acknowledgements
We wish to thank the JPI Climate initiative and participating grant institutes for funding the ISIpedia project. We also thank J. Burrough for professional advice on the English of a near-final draft. E.d.C. has received funding from the European Research Council (ERC) under the European Union’s Horizon 2020 research and innovation programme under grant agreement no. 756194 (ENERGYA). J.G. is supported by a research grant from Science Foundation Ireland (SFI) and the National Natural Science Foundation of China (NSFC) under the SFI-NSFC Partnership Programme, grant no. 17/NSFC/5181. D.P.v.V., R.S. and D.E.H.J.G. are supported by the Horizon 2020 NAVIGATE project, and D.P.v.V., R.S. and D.E.H.J.G. also acknowledge support from the COMMIT and Horizon 2020 ENGAGE project. F.P. acknowledges support through the project ENGAGE funded in the framework of the Leibniz Competition (SAW-2016-PIK-1), as well as through the project CHIPS, part of AXIS, an ERA-NET initiated by JPI Climate, and funded by FORMAS (SE), DLR/BMBF (DE, grant no. 01LS19XXY), AEI (ES) and ANR (FR) with cofunding by the European Union (grant no. 776608). R.S. acknowledges the financial support from the National Council for Scientific and Technological Development (CNPq), from the National Institute of Science and Technology for Climate Change Phase 2 under CNPq grant no. 465501/2014-1 and the National Coordination for High Level Education and Training (CAPES) grant no. 88887.136402/2017-00, all from Brazil. A.M. acknowledges support from the US Department of Energy, Office of Science’s Integrated Multisector Multiscale Modelling project and National Science Foundation’s Water Sustainability and Climate grant no. 1360445. This work was authored in part by the National Renewable Energy Laboratory (A.M.), operated by Alliance for Sustainable Energy, LLC, for the US Department of Energy (DOE) under Contract No. DE-AC36-08GO28308. S.F. is supported by the Environment Research and Technology Development Fund (2-1908 and 2-2002) provided by the Environmental Restoration and Conservation Agency, Japan. C.P. is supported by Korea Environment Industry & Technology Institute (KEITI) through Climate Change R&D Programme, funded by the Korea Ministry of Environment (MOE) (2018001310003).
Author information
Authors and affiliations.
Copernicus Institute of Sustainable Development, Utrecht University, Utrecht, the Netherlands
Seleshi G. Yalew, David E. H. J. Gernaat, Ioanna Mouratiadou & Detlef P. van Vuuren
Water Systems and Global Change Group, Wageningen University, Wageningen, the Netherlands
Seleshi G. Yalew, Michelle T. H. van Vliet & Fulco Ludwig
Policy Analysis, Department of Multi-Actor Systems, Technical University of Delft, Delft, the Netherlands
Seleshi G. Yalew
Department of Physical Geography, Utrecht University, Utrecht, the Netherlands
Michelle T. H. van Vliet
Netherlands Environmental Assessment Agency-PBL, The Hague, the Netherlands
David E. H. J. Gernaat & Detlef P. van Vuuren
Advanced Science Research Center, GC/CUNY, New York City, NY, USA
Ariel Miara
National Renewable Energy Laboratory, Golden, CO, USA
Department of Landscape Architecture, College of Urban Science, University of Seoul, Seoul, Korea
International Institute for Applied Systems Analysis-IIASA, Laxenburg, Austria
Edward Byers
Fondazione CMCC, Venice, Italy
Enrica De Cian & Shouro Dasgupta
Università Ca’ Foscari Venezia, Venice, Italy
Potsdam Institute for Climate Impact Research, Leibniz Association, Potsdam, Germany
Franziska Piontek & Robert Pietzcker
Joint Global Change Research Institute, Pacific Northwest National Laboratory, College Park, MD, USA
Gokul Iyer, Mohamad Hejazi & Silvia R. Santos da Silva
MaREI Centre, Environmental Research Institute, University College Cork, Cork, Ireland
James Glynn
Institute for Sustainable Resources, University College London, London, UK
Olivier Dessens
Programa de Planejamento Energético, COPPE, Universidade Federal do Rio de Janeiro, Rio de Janeiro, Brazil
Pedro Rochedo & Roberto Schaeffer
Center for Social and Environmental Systems Research, National Institute for Environmental Studies, Tsukuba, Japan
Shinichiro Fujimori
Department of Environmental Engineering, Kyoto University, Kyoto, Japan
Laboratoire d’économie appliquée de Grenoble, Grenoble, France
Silvana Mima
Department of Atmospheric and Oceanic Science, University of Maryland, College Park, MD, USA
Silvia R. Santos da Silva
Council on Energy, Environment and Water, New Delhi, India
Vaibhav Chaturvedi
Laboratoire des Sciences du Climat et l’Environnement-LSCE, Paris, France
Robert Vautard
You can also search for this author in PubMed Google Scholar
Contributions
S.G.Y. and D.P.v.V. codesigned the study. S.G.Y. collected and analysed data, and cowrote the initial draft manuscript with D.P.v.V. S.G.Y., D.P.v.V. and M.T.H.v.V. performed sectoral analysis of energy systems. S.G.Y., D.P.v.V., M.T.H.v.V., D.E.H.J.G., F.L., A.M., C.P., E.B., E.d.C., F.P., G.I., I.M., J.G., M.H., O.D., P.R., R.P., R.S., S.F., S.D., S.M., S.R.S.d.S., V.C. and R.V. contributed to the review of sectoral and regional climate impacts.
Corresponding author
Correspondence to Seleshi G. Yalew .
Ethics declarations
Competing interests.
The authors declare no competing interests.
Additional information
Publisher’s note Springer Nature remains neutral with regard to jurisdictional claims in published maps and institutional affiliations.
Supplementary information
Supplementary information.
Supplementary Tables 1–3, Note 1 and Fig. 1.
Source data
Source data fig. 2.
Data points of articles, their category and years published.
Source Data Fig. 3
Data points of article category, warming level and scenario years.
Source Data Fig. 4
Data points of percentage changes of climate impact per region.
Rights and permissions
Reprints and permissions
About this article
Cite this article.
Yalew, S.G., van Vliet, M.T.H., Gernaat, D.E.H.J. et al. Impacts of climate change on energy systems in global and regional scenarios. Nat Energy 5 , 794–802 (2020). https://doi.org/10.1038/s41560-020-0664-z
Download citation
Received : 13 August 2019
Accepted : 01 July 2020
Published : 03 August 2020
Issue Date : October 2020
DOI : https://doi.org/10.1038/s41560-020-0664-z
Share this article
Anyone you share the following link with will be able to read this content:
Sorry, a shareable link is not currently available for this article.
Provided by the Springer Nature SharedIt content-sharing initiative
This article is cited by
Extreme weather events on energy systems: a comprehensive review on impacts, mitigation, and adaptation measures.
- Ana C. R. Gonçalves
- Xurxo Costoya
- Margarida L. R. Liberato
Sustainable Energy Research (2024)
High-resolution meteorology with climate change impacts from global climate model data using generative machine learning
- Grant Buster
- Brandon N. Benton
- Ryan N. King
Nature Energy (2024)
Sustainable pathways towards universal renewable electricity access in Africa
- Rebecca Peters
- Jürgen Berlekamp
- Christiane Zarfl
Nature Reviews Earth & Environment (2024)
Urban water and electricity demand data for understanding climate change impacts on the water-energy nexus
- Renee Obringer
- Roshanak Nateghi
- Rohini Kumar
Scientific Data (2024)
Floating photovoltaics may reduce the risk of hydro-dominated energy development in Africa
- Wyatt Arnold
- Matteo Giuliani
- Andrea Castelletti

Quick links
- Explore articles by subject
- Guide to authors
- Editorial policies
Sign up for the Nature Briefing newsletter — what matters in science, free to your inbox daily.

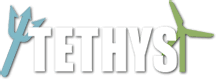
Reasons for Shifting and Barriers to Renewable Energy: A Literature Review
Consumption of fossil fuel resources leads to serious economic and environmental issues such as (high fossil fuel subsidies, high carbon emissions, and high energy demand). This current economic situation needs new methods, which should generate sustainable solutions that are mostly independent of the use of fossil fuels. However, there are many barriers to the development of renewable energy. Based on the literature the major barriers to renewable energy are economic, Policy and legal, and technical. A literature review was performed in this paper to determine the reasons for shifting from conventional energy to renewable energy and identifies the barriers to the development of renewable power generation.
MIT Technology Review
- Newsletters
Super-efficient solar cells: 10 Breakthrough Technologies 2024
Solar cells that combine traditional silicon with cutting-edge perovskites could push the efficiency of solar panels to new heights.
- Emma Foehringer Merchant archive page
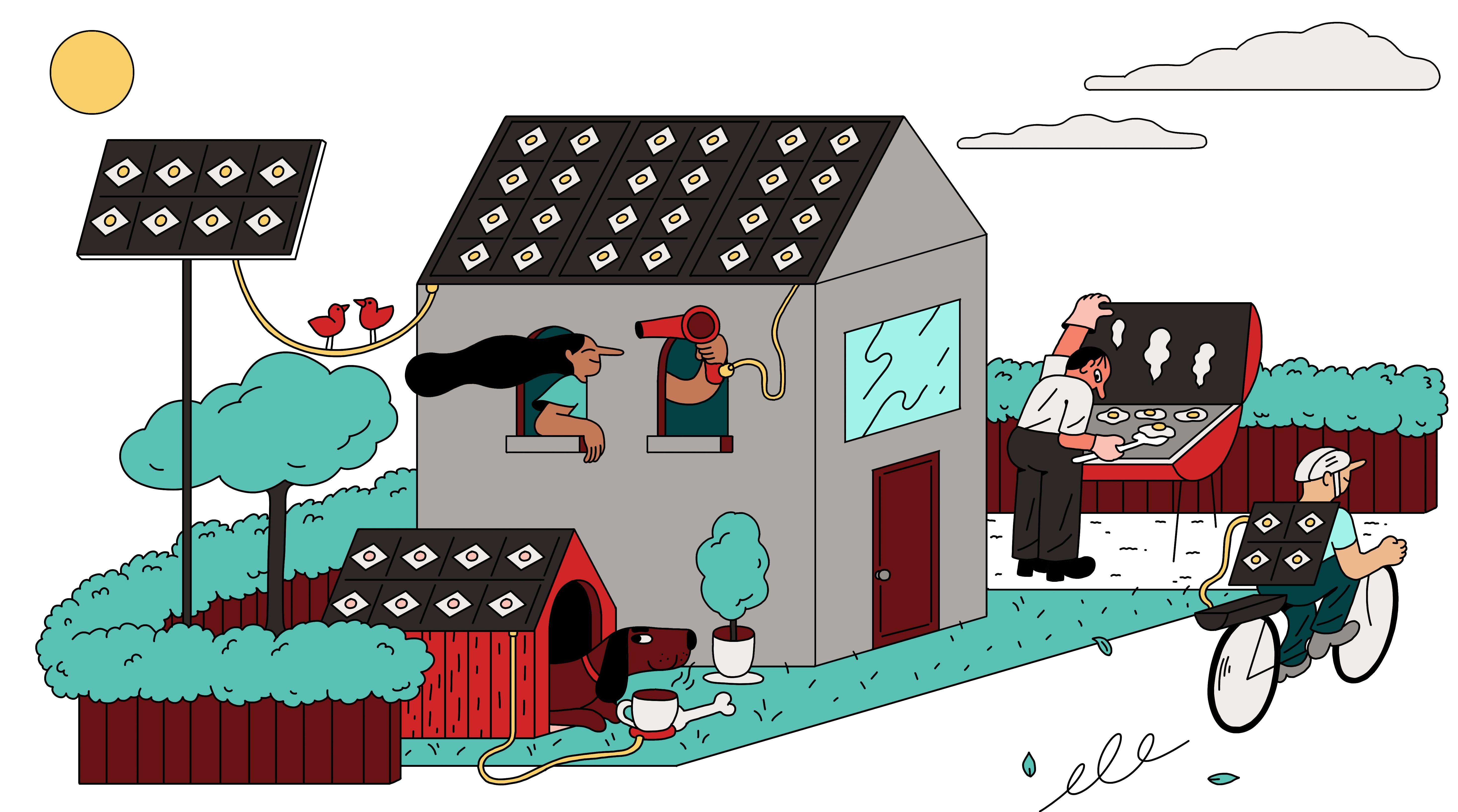
Beyond Silicon, Caelux, First Solar, Hanwha Q Cells, Oxford PV, Swift Solar, Tandem PV
3 to 5 years
In November 2023, a buzzy solar technology broke yet another world record for efficiency. The previous record had existed for only about five months—and it likely won’t be long before it too is obsolete. This astonishing acceleration in efficiency gains comes from a special breed of next-generation solar technology: perovskite tandem solar cells. These cells layer the traditional silicon with materials that share a unique crystal structure.
In the decade that scientists have been toying with perovskite solar technology , it has continued to best its own efficiency records, which measure how much of the sunlight that hits the cell is converted into electricity. Perovskites absorb different wavelengths of light from those absorbed by silicon cells, which account for 95% of the solar market today. When silicon and perovskites work together in tandem solar cells, they can utilize more of the solar spectrum, producing more electricity per cell.
Technical efficiency levels for silicon-based cells top out below 30%, while perovskite-only cells have reached experimental efficiencies of around 26%. But perovskite tandem cells have already exceeded 33% efficiency in the lab. That is the technology’s tantalizing promise: if deployed on a significant scale, perovskite tandem cells could produce more electricity than the legacy solar cells at a lower cost.
But perovskites have stumbled when it comes to actual deployment. Silicon solar cells can last for decades. Few perovskite tandem panels have even been tested outside.
The electrochemical makeup of perovskites means they’re sensitive to sucking up water and degrading in heat, though researchers have been working to create better barriers around panels and shifting to more stable perovskite compounds.
In May, UK-based Oxford PV said it had reached an efficiency of 28.6% for a commercial-size perovskite tandem cell, which is significantly larger than those used to test the materials in the lab, and it plans to deliver its first panels and ramp up manufacturing in 2024. Other companies could unveil products later this decade.
Climate change and energy
The problem with plug-in hybrids their drivers..
Plug-in hybrids are often sold as a transition to EVs, but new data from Europe shows we’re still underestimating the emissions they produce.
- Casey Crownhart archive page
Harvard has halted its long-planned atmospheric geoengineering experiment
The decision follows years of controversy and the departure of one of the program’s key researchers.
- James Temple archive page
How thermal batteries are heating up energy storage
The systems, which can store clean energy as heat, were chosen by readers as the 11th Breakthrough Technology of 2024.
These artificial snowdrifts protect seal pups from climate change
The human-built habitats shield the pups from predators and the freezing cold, but they’re threatened by global temperature rise.
- Matthew Ponsford archive page
Stay connected
Get the latest updates from mit technology review.
Discover special offers, top stories, upcoming events, and more.
Thank you for submitting your email!
It looks like something went wrong.
We’re having trouble saving your preferences. Try refreshing this page and updating them one more time. If you continue to get this message, reach out to us at [email protected] with a list of newsletters you’d like to receive.
Energy, exergy, and exergoeconomic cost optimization of wind-biomass multi-energy systems integrated for hydrogen production
- Published: 13 May 2024
Cite this article
- Caroline Acen 1 ,
- Olusola Bamisile ORCID: orcid.org/0000-0002-5154-6404 1 , 2 , 3 ,
- Michael Adedeji 4 ,
- Dongsheng Cai 1 ,
- Mustafa Dagbasi 4 ,
- Yihua Hu 3 &
- Iain Staffell 2
Explore all metrics
The recent effects of climate change and rising global warming levels have increased the need to transition towards clean energy. The use of multi-energy systems is one of the potential solutions to these issues, as validated in the literature. The production of hydrogen from cleaner sources has an integral role in decarbonizing the industrial, building, and transportation sectors. Hence, this study proposes novel multi-energy systems that can produce hydrogen from wind resources. The study is novel as it developed an innovative multi-energy system configuration and also considers hydrogen production as a means to utilize excess wind power production. The intermittency of wind resources has been a major drawback in using this renewable energy as the singular source for multi-generation systems. The multi-energy configuration developed and analyzed in this study proposed a solution for this by integrating a regenerative reheat biomass integrated power cycle as an auxiliary system for the multi-generation system (Wind-Bio-MGS). This system is modeled to produce electricity, heating, hot water, and hydrogen. The energy, exergy, and exergoeconomic approach is adopted in this study to evaluate the steady-state performance of the system, while the levelized cost of electricity (LCOE), levelized cost of heating (LCOh), and levelized cost of Hydrogen (LCOH) are also computed. A multi-objective optimization of the overall exergy efficiency and total product unit cost is presented. The parametric analysis of the energy systems is included to show the sensitivity of different parameters to changes and validate the robustness of the modeled system. The results show that the integration of hydrogen with wind-based multi-generation systems is a viable means of reducing carbon emissions and global warming. The overall energy and exergy efficiencies of the Wind-Bio-MGS system are 69.13% and 31.16%, respectively. The LCOE, LCOh, and LCOH for the system, respectively, are 0.02828 $ kWh −1 , 0.004038 $ kWh −1 , and 1.311 $ kg −1 s −1 .
This is a preview of subscription content, log in via an institution to check access.
Access this article
Price includes VAT (Russian Federation)
Instant access to the full article PDF.
Rent this article via DeepDyve
Institutional subscriptions
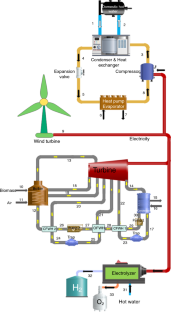
Abbreviations
Alkaline water electrolyzer
Brayton cycle
Specific cost
Closed feedwater heater
Carbon dioxide
Coefficient of performance
Specific exergy
Engineering equation solver
Greenhouse gas
Specific enthalpy
Hot water heater
Levelized cost of heating
Levelized cost of electricity
Levelized cost of hydrogen
Levelized cost of water
Linear Fresnel concentrator
Kilogram per cubic meter
Kilogram per second
Mass flow rate (kg s −1 )
Meters squared
Meters per second
Multi-effect desalination
Multi-generation energy system
Municipal solid waste
Open feedwater heater
Organic Rankine cycle
Proton exchange membrane
Parabolic trough collectors
Lower heating value
Heat transfer rate (kW)
Rankine cycle
Renewable energy sources
Specific entropy
Solid-oxide fuel cell
Steam Rankine cycle
Two-source multi-generation system
Work done/power
Exergy unit cost
Exergy efficiency
Dollar per kilogram
Dollar per kilowatts hour
Kilogram per hour
Dollar per hour
Destruction
Electricity
Heat exchanger
IPCC. Climate change 2021: the physical science basis. Cambridge: Cambridge University Press, 2021. https://doi.org/10.1017/9781009157896 .
IEA. World energy outlook 2020. IEA (2020) https://www.iea.org/reports/world-energy-outlook-2020 . Accessed 05, March, 2024
IEA. Renewables 2020. IEA , 2020. Available: https://www.iea.org/reports/renewables-2020 . Accessed 08 Nov 2023
UNFCC. The Paris agreement. Paris, 2016. https://unfccc.int/sites/default/files/english_paris_agreement.pdf . Accessed 05 March 2024.
IRENA. World energy transitions outlook: 1.5 °C pathway. In: International renewable energy agency, Abu Dhabi, 2021. www.irena.org/publications . Accessed 05 March 2024.
IRENA. Renewable capacity statistics 2021. In International renewable energy agency, Abu Dhabi, 2021 , www.irena.org/publications . Accessed: 05 March 2024
IEA. Achieving net zero heavy industry sectors in G7 members. IEA, Paris, 2022. https://www.iea.org/reports/achieving-net-zero-heavy-industry-sectors-in-g7-members . Accessed 05 March 2024
IEA. Energy technology perspectives 2020. IEA, Paris, 2020. Available: https://www.iea.org/reports/energy-technology-perspectives-2020 . Accessed 05 March 2024
Hassan Q, Sameen AZ, Salman HM, Jaszczur M, Al-Jiboory AK. Hydrogen energy future: advancements in storage technologies and implications for sustainability. J Energy Storage. 2023;72:108404. https://doi.org/10.1016/j.est.2023.108404 .
Article Google Scholar
Ferrada F, Babonneau F, Homem-de-Mello T, Jalil-Vega F. The role of hydrogen for deep decarbonization of energy systems: a Chilean case study. Energy Policy. 2023;177:113536. https://doi.org/10.1016/j.enpol.2023.113536 .
Article CAS Google Scholar
Rasul MG, Hazrat MA, Sattar MA, Jahirul MI, Shearer MJ. The future of hydrogen: challenges on production, storage and applications. Energy Convers Manag. 2022;272:116326. https://doi.org/10.1016/j.enconman.2022.116326 .
Ishaq H, Dincer I, Crawford C. A review on hydrogen production and utilization: challenges and opportunities. Int J Hydrogen Energy. 2022;47(62):26238–64. https://doi.org/10.1016/j.ijhydene.2021.11.149 .
Bamisile O, Cai D, et al. An innovative approach for geothermal-wind hybrid comprehensive energy system and hydrogen production modelling/process analysis. International Journal of Hydrogen Energy. 2022;47:27, p13261–13288. https://doi.org/10.1016/j.ijhydene.2022.02.084
Coelho A, Neyestani N, Soares F, Lopes JP. Wind variability mitigation using multi-energy systems. Int J Electr Power Energy Syst. 2020;118:105755. https://doi.org/10.1016/j.ijepes.2019.105755 .
Mahdavi M, Jurado F, Ramos RAV, Awaafo A. Hybrid biomass, solar and wind electricity generation in rural areas of Fez–Meknes region in Morocco considering water consumption of animals and anaerobic digester. Appl Energy. 2023;343:121253. https://doi.org/10.1016/j.apenergy.2023.121253 .
Lak Kamari M, Maleki A, Daneshpour R, Rosen MA, Pourfayaz F, Alhuyi Nazari M. Exergy, energy and environmental evaluation of a biomass-assisted integrated plant for multigeneration fed by various biomass sources. Energy. 2023;263:125649. https://doi.org/10.1016/j.energy.2022.125649 .
Lykas P, Bellos E, Kitsopoulou A, Tzivanidis C. Dynamic analysis of a solar-biomass-driven multigeneration system based on s-CO 2 Brayton cycle. Int J Hydrogen Energy. 2024;59:1268–86. https://doi.org/10.1016/j.ijhydene.2024.02.093 .
Sharifishourabi M, Dincer I, Mohany A. Performance assessment of a novel solar heliostat and digester-based multigeneration system with hydrogen production. Appl Therm Eng. 2023;232:121094. https://doi.org/10.1016/j.applthermaleng.2023.121094 .
Tian L, Zhang Z, Salah B, Marefati M. Multi-variable assessment/optimization of a new two-source multigeneration system integrated with a solid oxide fuel cell. Process Saf Environ Prot. 2023;179:754–73. https://doi.org/10.1016/j.psep.2023.08.003 .
Ghasemi A, Nikafshan Rad H, Akrami M, Marefati M. Exergoeconomic and exergoenvironmental analyzes of a new biomass/solar-driven multigeneration energy system: an effort to maximum utilization of the waste heat of gasification process. Therm Sci Eng Prog. 2024;48:102407. https://doi.org/10.1016/j.tsep.2024.102407 .
Nasrabadi AM, Korpeh M. Techno-economic analysis and optimization of a proposed solar-wind-driven multigeneration system; case study of Iran. Int J Hydrogen Energy. 2023;48(36):13343–61. https://doi.org/10.1016/j.ijhydene.2022.12.283 .
Mohammadi K, McGowan JG, Powell K. Thermoeconomic analysis of a multigeneration system using waste heat from a triple power cycle. Appl Therm Eng. 2021;190:116790. https://doi.org/10.1016/j.applthermaleng.2021.116790 .
Lin H, Liu J, Ifseisi AA, Taghavi M. A novel bio-waste-driven multigeneration cycle integrated with a solar thermal field and atmospheric water harvesting cycle: an effort to mitigate the environmental impacts of the wastewater treatment plants. Process Saf Environ Prot. 2023;180:386–403. https://doi.org/10.1016/j.psep.2023.09.066 .
Habib MA, Zubair SM. Second-law-based thermodynamic analysis of regenerative-reheat Rankine-cycle power plants. Energy. 1992;17(3):295–301. https://doi.org/10.1016/0360-5442(92)90057-7 .
Bamisile O, Huang Q, Dagbasi M, Abid M, Okafor EC, Ratlamwala TAH. Concentrated solar powered novel multi-generation system: a energy, exergy, and environmental analysis. J Sol Energy Eng. 2020;142(5):051005–17. https://doi.org/10.1115/1.4046392 .
Bamisile OO, Dagbasi M, Abbasoglu S. Energy and exergy analyses of a novel solar PTC assisted multi-generation system. Int J Exergy. 2019;28(2):158–82. https://doi.org/10.1504/IJEX.2019.097978 .
Bamisile O, Huang Q, Hu W, Dagbasi M, Kemena AD. Performance analysis of a novel solar PTC integrated system for multi-generation with hydrogen production. Int J Hydrog Energy. 2020;45(1):190–206. https://doi.org/10.1016/j.ijhydene.2019.10.234 .
Bamisile O, et al. Modelling and performance analysis of an innovative CPVT, wind and biogas integrated comprehensive energy system: An energy and exergy approach. Energy Convers Manag. 2020;209:112611. https://doi.org/10.1016/j.enconman.2020.112611 .
Cengel Y, Boles M. Thermodynamics: an engineering approach. 8th ed. New York: McGraw-Hill Education; 2014.
Google Scholar
Bamisile O, et al. An innovative approach for geothermal-wind hybrid comprehensive energy system and hydrogen production modeling/process analysis. Int J Hydrog Energy. 2022;47(27):13261–88. https://doi.org/10.1016/j.ijhydene.2022.02.084 .
Bamisile O, et al. Environmental impact of hydrogen production from Southwest China’s hydro power water abandonment control. Int J Hydrog Energy. 2020;45(46):25587–98. https://doi.org/10.1016/j.ijhydene.2020.06.289 .
Download references
Acknowledgements
This study was supported by National Natural Science Foundation of China (Grant No. 52007025), Sichuan Provincial Key Lab for Power System-Wide Area Measurement (Grant No. 2021KP012), Science and Technology Innovation Talent Program of Sichuan Provincial (Grant No. 22CJDRC0025), and Science and Technology Innovation Talent Program of Sichuan Provincial (Grant No. 22CXRC0010).
Author information
Authors and affiliations.
Nuclear Science and Technology, College of Nuclear Science and Automation Engineering, Chengdu University of Technology, Sichuan, People’s Republic of China
Caroline Acen, Olusola Bamisile & Dongsheng Cai
Centre for Environmental Policy, Faculty of Natural Science, Imperial College London, London, UK
Olusola Bamisile & Iain Staffell
Energy and Environment Division, University of Dundee, Dundee, Scotland, UK
Olusola Bamisile & Yihua Hu
Energy Systems Engineering Department, Cyprus International University, Mersin 10, KKTC, Turkey
Michael Adedeji & Mustafa Dagbasi
You can also search for this author in PubMed Google Scholar
Corresponding author
Correspondence to Olusola Bamisile .
Ethics declarations
Conflict of interest.
The authors declare that they have no known competing financial interests or personal relationships that could have appeared to influence the work reported in this paper. The authors declare the following financial interests/personal relationships which may be considered as potential competing interests.
Additional information
Publisher's note.
Springer Nature remains neutral with regard to jurisdictional claims in published maps and institutional affiliations.
Rights and permissions
Springer Nature or its licensor (e.g. a society or other partner) holds exclusive rights to this article under a publishing agreement with the author(s) or other rightsholder(s); author self-archiving of the accepted manuscript version of this article is solely governed by the terms of such publishing agreement and applicable law.
Reprints and permissions
About this article
Acen, C., Bamisile, O., Adedeji, M. et al. Energy, exergy, and exergoeconomic cost optimization of wind-biomass multi-energy systems integrated for hydrogen production. J Therm Anal Calorim (2024). https://doi.org/10.1007/s10973-024-13135-2
Download citation
Received : 20 August 2023
Accepted : 31 March 2024
Published : 13 May 2024
DOI : https://doi.org/10.1007/s10973-024-13135-2
Share this article
Anyone you share the following link with will be able to read this content:
Sorry, a shareable link is not currently available for this article.
Provided by the Springer Nature SharedIt content-sharing initiative
- Energy and exergy modeling
- Levelized cost analysis
- Multi-energy systems
- Optimization
- Wind energy
- Find a journal
- Publish with us
- Track your research
- Election 2024
- Entertainment
- Newsletters
- Photography
- Personal Finance
- AP Investigations
- AP Buyline Personal Finance
- AP Buyline Shopping
- Press Releases
- Israel-Hamas War
- Russia-Ukraine War
- Global elections
- Asia Pacific
- Latin America
- Middle East
- Election Results
- Delegate Tracker
- AP & Elections
- Auto Racing
- 2024 Paris Olympic Games
- Movie reviews
- Book reviews
- Personal finance
- Financial Markets
- Business Highlights
- Financial wellness
- Artificial Intelligence
- Social Media
More and faster: Electricity from clean sources reaches 30% of global total
Solar panels work near the small town of Milagro, Navarra Province, northern Spain, Feb. 24, 2023. (AP Photo/Alvaro Barrientos)
- Copy Link copied
Billions of people are using different kinds of energy each day and 2023 was a record-breaking year for renewable energy sources — ones that don’t emit planet-warming pollutants like carbon dioxide and methane — according to a report published Wednesday by Ember, a think tank based in London.
For the first time, 30% of electricity produced worldwide was from clean energy sources as the number of solar and wind farms continued to grow fast.
Of the types of clean energy generated last year, hydroelectric dams produced the most. That’s the same as in most years. Yet droughts in India, China, North America and Mexico meant hydropower hit a five-year low. Research shows climate change is causing droughts to develop more quickly and be more severe .
People used more electricity than ever last year, about 2% more, an increase of about as much as Canada uses in a year. Some of this new demand was for heat pumps , which are an efficient way to both heat and cool buildings, and for electric vehicles . It was also for electrolyzers, special machines used to get hydrogen out of water, for energy. These are all technologies that provide solutions to climate change.
Other increased demand was for electricity to feed new data centers and for air conditioning as places around the world become hotter.
Solar made up the biggest share of new clean energy last year. More than twice as much solar power was added as coal power. It was the 19th year in a row that solar was the fastest-growing source of electricity generation. A surge in solar installations happened at the end of the year and the report predicts 2024 will see an even larger jump.
China added more renewable energy than any other country last year — 51% of the new solar power and 60% of the new wind power globally. China, the European Union, the United States and Brazil together accounted for 81% of new solar generation in 2023.
Yet China was also responsible for 55% of coal generation globally and 60% of China’s electricity generation came from coal. The International Energy Agency says coal is the most carbon-intensive of the fossil fuels.
Scientists say emissions from burning fuels like coal must ramp steeply down to protect Earth’s climate, yet there was an increase in electricity made from burning fossil fuels. China, India, Vietnam and Mexico were responsible for nearly all of the rise.
The report said some countries burned coal to make up for the loss of hydroelectric power they experienced when drought caused their reservoirs to dry up. This is an example of a vicious cycle — when climate change prompts the use of more of the substances that cause climate change in the first place.
Despite all the growth in clean energy, fossil fuels still made up the majority of global electricity generated last year, causing a 1% rise in global power sector emissions. Scientists say even if we slashed all greenhouse gas emissions today, the planet would continue to warm for years because of the amount of pollutants already added to the atmosphere.
Analysts expect the world to use even more electricity in 2024. But renewable energy generation is forecast to grow even faster. That could mean a 2% drop (333 terawatt-hours) in energy generated from fossil fuels.
The Associated Press’ climate and environmental coverage receives financial support from multiple private foundations. AP is solely responsible for all content. Find AP’s standards for working with philanthropies, a list of supporters and funded coverage areas at AP.org .
Advertisement
Giant Batteries Are Transforming the Way the U.S. Uses Electricity
They’re delivering solar power after dark in California and helping to stabilize grids in other states. And the technology is expanding rapidly.
By Brad Plumer and Nadja Popovich
How California powered itself in April 2021 …
and in April 2024.
Peak demand
average daily generation, by fuel type
Solar power
Source: California Independent System Operator via Grid Status
Please see the bottom of this page for notes.
By The New York Times
California draws more electricity from the sun than any other state. It also has a timing problem: Solar power is plentiful during the day but disappears by evening, just as people get home from work and electricity demand spikes. To fill the gap, power companies typically burn more fossil fuels like natural gas.
That’s now changing. Since 2020, California has installed more giant batteries than anywhere in the world apart from China. They can soak up excess solar power during the day and store it for use when it gets dark.
Those batteries play a pivotal role in California’s electric grid, partially replacing fossil fuels in the evening. Between 7 p.m. and 10 p.m. on April 30, for example, batteries supplied more than one-fifth of California’s electricity and, for a few minutes, pumped out 7,046 megawatts of electricity, akin to the output from seven large nuclear reactors.
Across the country, power companies are increasingly using giant batteries the size of shipping containers to address renewable energy’s biggest weakness: the fact that the wind and sun aren’t always available.
“What’s happening in California is a glimpse of what could happen to other grids in the future,” said Helen Kou, head of U.S. power analysis at BloombergNEF, a research firm. “Batteries are quickly moving from these niche applications to shifting large amounts of renewable energy toward peak demand periods.”
Over the past three years, battery storage capacity on the nation’s grids has grown tenfold, to 16,000 megawatts. This year, it is expected to nearly double again, with the biggest growth in Texas, California and Arizona.
Battery Storage Plants Across the United States U.S.
California has more
grid-scale battery storage than any other state.
Net summer operating capacity
Texas is quickly adding new battery capacity.
California has more grid-scale battery storage than any other state.
Source: U.S. Energy Information Administration
Note: Each circle represents a facility that has at least one battery as of March 2024.
Most grid batteries use lithium-ion technology, similar to batteries in smartphones or electric cars. As the electric vehicle industry has expanded over the past decade, battery costs have fallen by 80 percent, making them competitive for large-scale power storage. Federal subsidies have also spurred growth .
As batteries have proliferated, power companies are using them in novel ways, such as handling big swings in electricity generation from solar and wind farms, reducing congestion on transmission lines and helping to prevent blackouts during scorching heat waves.
In California, which has set ambitious goals for fighting climate change, policymakers hope grid batteries can help the state get 100 percent of its electricity from carbon-free sources by 2045. While the state remains heavily dependent on natural gas, a significant contributor to global warming, batteries are starting to eat into the market for fossil fuels. State regulators plan to nearly triple battery capacity by 2035.
“The future is bright for energy storage,” said Andrés Gluski, chief executive of AES Corporation, one of the world’s largest power companies. “If you want more renewables on the grid, you need more batteries. It’s not going to work otherwise.”
How Batteries Work on the Grid Today

A battery storage facility under construction in Menifee, Calif., in March. The site, at 43 acres, is expected to be the largest in the state when completed.
Mike Blake/Reuters
When power companies first began connecting batteries to the grid in the 2010s, they mainly used them to smooth out small disruptions in the flow of electricity, say, if a power plant unexpectedly tripped offline. Many battery operators still earn most of their revenue by providing these “ancillary services.”
But power companies also use batteries to engage in a type of trading: charging up when electricity is plentiful and cheap and then selling power to the grid when electricity supplies are tighter and more expensive.
In California power prices often crash around midday, when the state produces more solar power than it needs, especially in the spring when air-conditioning use is low. Prices then soar in the evening when solar disappears and grid operators have to increase output from gas plants or hydroelectric dams to compensate.
California How Batteries Operated on the Grid in April 2024
April 30: Peak
battery output
Batteries mostly charge during the middle of the day, when cheap solar power is abundant.
+6,000 megawatts
Each line is a
day in April
April 8: Solar
−2,000
And discharge when the sun goes down, sending power back to the grid.
−4,000
How batteries operated on the grid
in California in April 2024
Sources: California Independent System Operator via Grid Status
California now has 10,000 megawatts of battery power capacity on the grid , enough to power 10 million homes for a few hours. Those batteries are “able to very effectively manage that evening ramp where solar is going down and customer demand is increasing,” said John Phipps, executive director of grid operations for the California Independent System Operator, which oversees the state’s grid.
Batteries can also help California’s grid handle stresses from heat waves and wildfires, Mr. Phipps said. “It made some differences last summer,” he said. “We were able to meet high load days and wildfire days when we might lose some power lines.”
In Texas, batteries are still largely used to provide ancillary services, stabilizing the grid against unexpected disruptions. Texas is also more reliant than California on wind energy, which fluctuates in less predictable patterns.
But Texas is quickly catching up to California in solar power, and batteries increasingly help with evening peaks. On April 28, the sun was setting just as wind power was unexpectedly low and many coal and gas plants were offline for repairs. Batteries jumped in, supplying 4 percent of Texas’ electricity at one point , enough to power a million homes. Last summer, batteries helped avert evening blackouts by providing additional power during record heat.
Texas How Batteries Operated on the Grid in April 2024
April 28: Peak
+2,000 megawatts
in Texas in April 2024
Sources: Electric Reliability Council of Texas via Grid Status
The two states built their battery fleets in distinct ways. In California, regulatory mandates were a key impetus: In 2019, officials worried that too many older gas plants were closing, risking blackouts, and ordered utilities to quickly install thousands of megawatts of storage.
In Texas, market forces dominate. The state’s deregulated electricity system allows prices to fluctuate sharply, rising as high as $5,000 per megawatt-hour during acute shortages. That makes it lucrative for battery developers to take advantage of spikes, such as in locations where power lines periodically get clogged.
“Anywhere we think the market is going to get tight, you can put batteries in and even things out,” said Stephanie Smith, chief operating officer of Eolian, a battery developer. “Then, we’re making bets all day about when to charge and discharge.”
One battery, for instance, sits near Fort Worth, absorbing excess wind power from West Texas during the nighttime, when no one needs it, and feeding it into the grid when demand surges.
Other states are following. In Arizona and Georgia, utilities plan to install thousands of megawatts of battery capacity to help manage rising demand from data centers and factories. It helps that batteries can be deployed quickly, said Aaron Mitchell, vice president of planning and pricing at Georgia Power.
The industry still faces obstacles, however. Lithium-ion batteries are flammable, and while operators have taken steps to reduce fire risk, some communities oppose projects in their backyards . Most batteries still come from China, making them vulnerable to trade disputes. In Texas, a state fund to subsidize gas plants could undercut the battery boom. In other states, complex regulations sometimes prevent utilities from adding energy storage .
“Because these storage resources are so new, the rules are still catching up,” said Natalie McIntire, who works on grid issues for the Natural Resources Defense Council, an environmental group.
Can Grid Batteries Help Fight Climate Change?

Wind turbines near Sweetwater, Texas. Nationwide, battery storage is being used to address renewable energy’s biggest weakness: the fact that the wind and sun aren’t always available.
Tamir Kalifa for The New York Times
Grid batteries could be a useful tool to slash planet-warming emissions, experts say, though they still need further advances in terms of costs, technologies and how they are used.
In Texas, many batteries today are actually increasing carbon-dioxide emissions, according to one analysis . That’s because operators focus on maximizing revenue and sometimes charge with coal or gas power.
“These batteries have an immense capability to abate carbon, but they need the right incentives to do so,” said Emma Konet, co-founder of Tierra Climate , a startup working to help batteries earn money for reducing emissions.
In California, by contrast, batteries appear to be cutting emissions from fossil fuels. The state’s gas use in April fell to a seven-year low . “We have reached the conclusion that batteries are displacing natural gas when solar generation is ramping up and down each day,” said Max Kanter, chief executive of Grid Status, an electricity data tracking firm.
Yet California still gets roughly 40 percent of its electricity from natural gas, and it could be difficult for current battery technology to replace all of that. One analysis from BloombergNEF found that solar and batteries can be a cost-effective alternative to smaller gas “peaker” plants that only switch on when demand spikes. But batteries remain too costly to replace many of the larger gas-burning plants that provide steadier power day and night.
“You don’t want to necessarily build a system where you’ve got batteries to suck up every last megawatt-hour, because that’s a pretty expensive system,” said Meredith Fowlie, an economist at the University of California, Berkeley.
Today’s lithium-ion batteries typically only deliver power for two to four hours before needing to recharge. If costs keep falling, battery companies might be able to extend that to eight or ten hours (it’s a matter of adding more battery packs) but it may not be economical to go far beyond that, said Nate Blair, an energy storage expert at the National Renewable Energy Laboratory.
That means additional long-duration storage technologies could be needed. If California wants to rely largely on renewable energy, it will have to handle weeklong periods where there’s no wind and little sun . Another challenge: There’s far more solar power available in summer than in winter, and no battery today can store electricity for months to manage those seasonal disparities.
Some companies are exploring solutions. In Sacramento, a start-up called ESS is building “flow” batteries that store energy in liquid electrolytes and can last 12 hours or longer. Another start-up, Form Energy, is building a 100-hour iron-air battery . These ideas will have to compete against alternatives like nuclear power, advanced geothermal or even using green hydrogen to store electricity .
California’s regulators say they may need five times as much storage capacity by midcentury, even if it’s unclear which technologies will prevail.
“We’re just at the beginning of this,” said Mr. Phipps of the California Independent System Operator.

Ross D. Franklin/Associated Press
In the top graphic, charts reflect average daily power generation, by fuel type, in five-minute increments for the month of April. The charts show imports from other regions, as well as times when battery power is discharged to the grid, but they do not show battery charging or electricity exports. The data reflects utility-scale generation and does not include “behind-the-meter” sources, such as rooftop solar panels. No adjustments are made for variations in weather.
The California Independent System Operator’s method for counting natural gas generation resources changed in December 2023 . Before then, the organization had been slightly overcounting gas “on the range of a few hundred megawatts,” according to a spokesperson.
Average sunrise and sunset times are shown on the charts.
- Share full article
Our Coverage of Climate and the Environment
News and Analysis
Florida’s state government will no longer be required to consider climate change when crafting energy policy under legislation signed by Gov. Ron DeSantis, a Republican.
Scientists have already established that the summer of 2023 was the warmest in the Northern Hemisphere since around 1850. Now, researchers say it was the hottest in 2,000 years .
The Federal Energy Regulatory Commission, an obscure climate agency , approved sweeping changes to how America’s electric grids are planned and funded . The new rule could help speed up wind and solar energy.
A Cosmic Perspective: Alarmed by the climate crisis and its impact on their work, a growing number of astronomers are using their expertise to fight back.
Struggling N.Y.C. Neighborhoods: New data projects are linking social issues with global warming. Here’s what that means for five communities in New York .
Biden Environmental Rules: The Biden administration has rushed to finalize 10 major environmental regulations to meet its self-imposed spring deadline.
F.A.Q.: Have questions about climate change? We’ve got answers .

COMMENTS
A review of renewable energy sources, sustainability issues and climate change mitigation ... It is evidential in literature that replacing fossil fuel-based energy sources with renewable energy sources, which includes: bioenergy, direct solar energy, geothermal energy, hydropower, wind and ocean energy (tide and wave), would gradually help the ...
The use of renewable energy resources, such as solar, wind, and biomass will not diminish their availability. Sunlight being a constant source of energy is used to meet the ever-increasing energy need. This review discusses the world's energy needs, renewable energy technologies for domestic use, and highlights public opinions on renewable energy. A systematic review of the literature was ...
Step by step the energy request is broadening and in this way the necessities for a manageable source that won't hurt the climate are of prime significance. Several projections express that by 2050 the energy requesting will basically increase. ... In this review paper the renewable energy s power and warmth, which is produced using sun based ...
The transition toward a 100% Renewable Energy System is a complex process with different technical and economic challenges. In order to achieve predetermined goals, several steps should be carried out simultaneously, including increment of energy efficiency, savings in primary energy consumption, and finally, deployment of variable renewable energy sources (VRES) [1].
If the definition of "alternative energy" is considered over time, two main considerations are to be drawn. In the first place, the literature usually separates between "traditional," "conventional," "modern," or "new" energy sources (Goldemberg and Lucon 2012).Traditional primary energy sources would be wood, for example, while conventional energy is medium and big ...
Climate change mitigation, the goal of reducing CO2 emissions, more stringent regulations and the increment in energy costs have pushed researchers to study energy efficiency and renewable energy sources. Manufacturing systems are large energy consumers and are thus responsible for huge greenhouse gas emissions; for these reasons, many studies have focused on this topic recently. This review ...
About other renewable energy sources, Turkey had the largest share in generating energy from wind and solar energy, with an annual growth rate of (88.03%, 98.22%), respectively.
Clean, affordable, and efficient energy sources are inevitable for a sustainable world. Energy crisis, especially the poor access and affordability, demand-supply mismatches, energy inequality, and high dependence on non-renewable energy sources, are the challenges before the attainment of clean energy goals for sustainable development. The 5-year review from the adoption of sustainable ...
On the supply side, renewable energy sources including bioenergy, ... E. T. Measuring climatic impacts on energy consumption: a review of the empirical literature. Energy Econ. 46, 522-530 (2014).
Moreover, these non-renewable resources are available in limited amount, and their formation requires long period. Thus, there is an urgent need to identify the eco-friendly sources of energy which may replace these non-renewable resources and would be able to meet the energy requirements of huge population of the world.
Worldwide, energy is harnessed from fossil fuels as well as from alternative sources of energy. However, for energy generation, fossil fuels are exploited at a larger scale, and the role of alternative energy sources is not substantial. In future, the contribution of renewable energy sources will be crucial for energy sustainability.
The role of hydrogen as a clean energy source is a promising but also a contentious issue. The global energy production is currently characterized by an unprecedented shift to renewable energy sources (RES) and their technologies. However, the local and environmental benefits of such RES-based technologies show a wide variety of technological maturity, with a common mismatch to local RES ...
This article provides a critical review of the literature on the relationship between renewable energies and sustainability considering the three dimensions of sustainability: economic, social, and environmental. First, a bibliometric tool is used and then a more in-depth analysis of selected literature is performed, focusing on the type of renewable energy analyzed and the level of ...
The social and political perspectives are important considerations for renewable energy technologies. These perspectives may have impacts that are positive, negative, or a combination. Positive impacts can improve the adoption of certain technologies. Adverse impacts can reduce the intended benefits or even threaten the viability of a ...
Fossil fuels are also regarded as conventional fuels, are the prime sources of non-renewable energy, whose loss cannot be sustained in years. According to the 2019 global statistical review of world energy, there is a 0.5% increased carbon dioxide emission rate and 1.3% primary energy consumption worldwide.
The importance of alternative energy sources comes together with climate change challenges associated with e excessive use of fossil fuels. th primary There are three motivators that stimulate the growth of renewable energy technologies: energy security, economic impacts and carbon dioxide emission reduction. The term "alternative energy"
3.1 Renewable Energy Planning and Policy Selecting between alternative energy sources has usually focused only on cost minimization. It is widely recognized now that energy planning is a much more complicated decision with many actors and factors involved. Pohekar and Table 1 Literature Review on MCDM Methods and Application to Renewable Energy ...
On the other hand, according to Word Watch Institute, in its report on The Road to the Future for Renewable Energy in CA, to develop a wind energy project is estimated an approximate cost of $ 36.5 million dollars to generate electricity for about 30 years. It would have a capacity to produce about 13 megawatts.
A literature review was performed in this paper to determine the reasons for shifting from conventional energy to renewable energy and identifies the barriers to the development of renewable power generation. Consumption of fossil fuel resources leads to serious economic and environmental issues such as (high fossil fuel subsidies, high carbon ...
In this study, studies on the use of renewable energy sources such as solar and wind on ships and alternative energy sources such as onshore energy are examined in order to reduce fuel consumption and minimize exhaust gas emissions. Current information on the use of alternative energy systems in ship technology is given.
There are various renewable energy resources are available in nature mainly like solar, wind, geo-thermal, tidal, biomass etc. So, in this paper, a brief literature review is carried away to get an idea that how these renewable energy resources had been used so far and to have an idea about the evolution of these energy resources.
The literature review covers different studies that examined the impacts of renewable energy on economic growth, job creation, welfare, CO2 emissions, electricity prices, and fuel imports. Researches have used different methodological approaches, different periods, and different countries to examine the impacts of renewable energy. The studies ...
Water is an essential requirement for agricultural productivity. In the agriculture sector, electricity generated by conventional sources contributes to a substantial amount of carbon footprints for pumping water through tube wells. Over the past few decades, a transitional shift towards renewable resources has increased leading to decarbonizing the environment and is considered as a viable ...
Renewable energy sources have emerged as an alternative to meet the growing demand for energy, mitigate climate change, and contribute to sustainable development. The integration of these systems is carried out in a distributed manner via microgrid systems; this provides a set of technological solutions that allows information exchange between the consumers and the distributed generation ...
Traditional locations for energy generation (e.g. lignite or hard coal mining areas) may lose their substantial significance in favour of renewable energy generation locations, if the former do not possess suitable local conditions for the generation of renewable energy (RE), 1 which leads to economic losses. In contrast, locations where energy ...
In May, UK-based Oxford PV said it had reached an efficiency of 28.6% for a commercial-size perovskite tandem cell, which is significantly larger than those used to test the materials in the lab ...
The world has passed a clean energy milestone, as a boom in wind and solar meant a record-breaking 30% of the world's electricity was produced by renewables last year, new data shows. The planet ...
The recent effects of climate change and rising global warming levels have increased the need to transition towards clean energy. The use of multi-energy systems is one of the potential solutions to these issues, as validated in the literature. The production of hydrogen from cleaner sources has an integral role in decarbonizing the industrial, building, and transportation sectors. Hence, this ...
Billions of people are using different kinds of energy each day and 2023 was a record-breaking year for renewable energy sources — ones that don't emit planet-warming pollutants like carbon dioxide and methane — according to a report published Wednesday by Ember, a think tank based in London.. For the first time, 30% of electricity produced worldwide was from clean energy sources as the ...
Since 2020, California has installed more giant batteries than anywhere in the world apart from China. They can soak up excess solar power during the day and store it for use when it gets dark ...