Risk Management
NASA’s Risk Management program’s mission is to provide a unified risk management structure that applies to all agency activities and all applicable risks and interactions, and is integrated across organizational boundaries to ensure that risk management decisions are delegated and/or elevated to the appropriate level. It is also the program’s mission that every organization has access to the information needed from subordinate organizations to effectively manage risks at its level. Risk Management includes opportunity management — recognizing that spaceflight is an inherently risky endeavor and that the proper attitude towards risk management is to reach an optimal balance between minimizing the potential for loss while maximizing the potential for gain (opportunity).
The various forms of Risk Management that are being pursued at NASA include:
Strategic, program/project, institutional, acquisition, and enterprise RM.
All forms of Risk Management consist of two main processes:
- Risk- (and Opportunity-) Informed Decision Making addresses informed selection of decision alternatives to assure effective approaches to achieving objectives.
- Continuous Risk (and Opportunity) Management addresses implementation of the selected alternative to assure that requirements are met.
All forms of Risk Management are concerned with the management of both known and Unknown/Underappreciated (UU) risks. UU risks often are associated with organizational and management issues such as oversight effectiveness, attention to best practices and employment of robust margins.

Risk Management News

Cancellation of NPR 8705.5 Reflects Evolution of NASA Policy for Safety and Mission Success
NPR 8705.5, Technical Probabilistic Risk Assessment Procedures for Safety and Mission Success for NASA Programs and Projects was cancelled, a move consistent with the policy direction to be more objectives-driven, as defined in the recent update to NPD 8700.1 , NASA Policy for Safety and Mission Success.

Changes to NPR 8000.4 Reflect Increased Relevancy of Cyber-Related Threats
Revision C of NPR 8000.4 , Agency Risk Management Procedural Requirements went into effect on April 19, 2022. The changes made primarily reflect the increasing relevance of cyber-related threats and incidents across both government agencies and the private sector. The update reflects an action item from the NASA Enterprise Protection Board to the Office of Safety and Mission Assurance to make the subject of cybersecurity and cyber risk explicit within NPR 8000.4.

Revision B of NPR 8000.4
Changes to NPR 8000.4B, Agency Risk Management Procedural Requirements, went into effect on Dec. 6, 2017, thereby replacing NID 8000-108 and NPR 8000.4A.
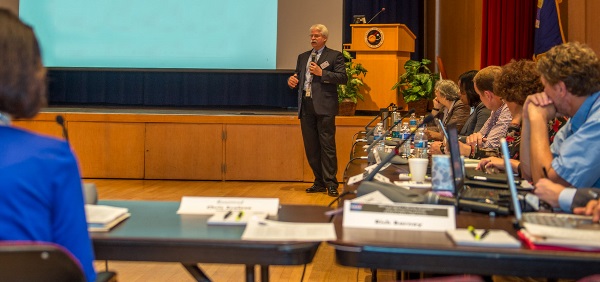
OSMA Hosts Risk Management Summit to Address Key Challenges and Opportunities
The Office of Safety and Mission Assurance hosted a Risk Management (RM) summit to address key RM challenges and opportunities for the agency.
Agency Risk Management Working Group
The Agency Risk Management Working Group was established to promote Risk Management and best practices and implementation of agency-level Risk Management policies within NASA and to communicate risks to the Office of Safety and Mission Assurance. It addresses the opportunities, challenges and concerns noted by NASA centers that develop and implement center-level Risk Management policies, plans and processes.
The Agency Risk Management Working Group covers the spectrum of related activities across institutional Risk Management and program and project Risk Management. Best practices and lessons learned that are identified through this working group are shared to promote and enhance communication and collaboration across the centers.
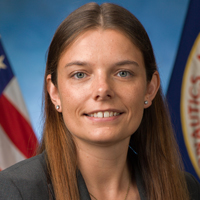
Dr. Mary Skow
Agency risk management officer.
Learn more about Agency Risk Management Officer Dr. Mary Skow.
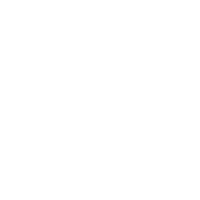
Dr. Homayoon Dezfuli
Risk management program manager.
Learn more about Risk Management Program Manager Dr. Homayoon Dezfuli.
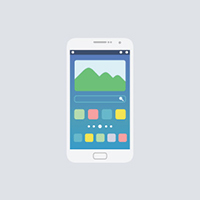
Points of Contact
For details on contacting a Risk Management Point of Contact (PoC) for your location, click below.
SATERN Courses
This course covers in detail one of the two processes comprised in the NASA Risk Management process required by NPR 8000.4 . Its content is based on the NASA RIDM Handbook and it provides detailed guidance for implementing the RIDM requirements of NPR 8000.4, with a specific focus on programs and projects in the Formulation Phase. Content includes RIDM process interfaces, the technical details of the various steps in the RIDM process and a case study with opportunities for student participation that runs throughout the course to illustrate the key learning points. This course is intended for NASA program, project, mission support, institutional, systems engineering, and Safety and Mission Assurance personnel who wish to play a role in bringing about improved decision-making anywhere in the agency.

Policy and Guidance
Recommended reading, important links.
Dr. Mary R. Coan Skow, Ph.D., is the Agency Risk Management Officer. In this role, which she helped formulate and establish, Dr. Skow integrates risks from various boards and forums to achieve perspective on top-enterprise risks. Additionally, she champions risk leadership initiatives through the facilitation of risk communication and clarifies risk posture. Dr. Skow also develops and implements best practices to integrate Enterprise Risk Management processes into day-to-day operations and decision-making, while investigating and developing methods to create and manage top risks for the agency. Prior to her current position, Dr. Skow served as the strategic evaluation program manager for the Chief Program Management Officer at NASA Headquarters, where she excelled at motivating diverse teams and initiating strategic initiatives. She previously held the role of portfolio analyst at Headquarters for the Office of the Chief Financial Officer, where she was responsible for understanding and working with the Exploration Systems Development Mission Directorate for the Human Landing System, Gateway and Spacesuit User Interface Technologies for Students (SUITS). Dr. Skow began her career at NASA as a graduate student in the co-op program at Kennedy Space Center working on In-Situ Resource Utilization. Following her co-op, she became a project manager at NASA Johnson Space Center for Environmental Control Life Support Systems. Dr. Skow graduated from the University of Rochester with a Bachelor of Science degree in chemical engineering. She received her PhD in chemical engineering with a focus in microelectronics from Texas A&M University. Dr. Skow actively participates in outreach programs, mentoring underprivileged children and college students and promoting NASA's mission to the next generation of scientists and engineers.
- Interact with NASA APPEL on LinkedIn
- Interact with NASA APPEL on Twitter
- Interact with NASA APPEL on Facebook
- View NASA APPEL Images on Flickr
- View NASA APPEL Videos on YouTube
- Subscribe to NASA APPEL's RSS Feed
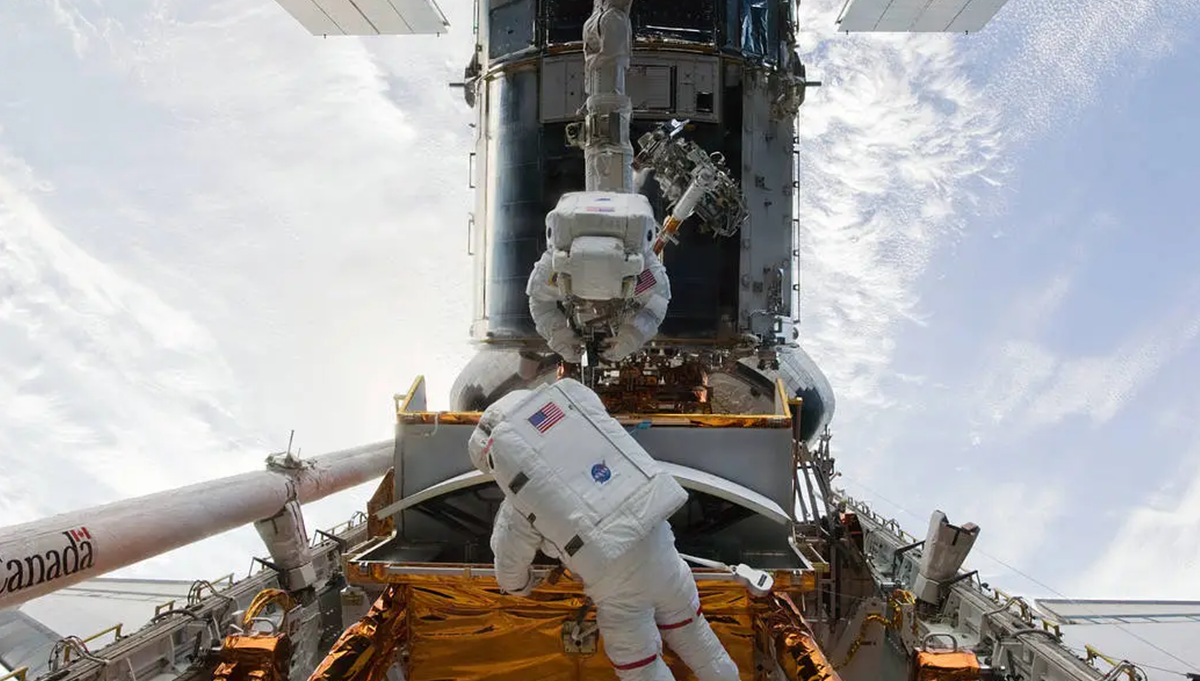
Subscribe to INSIGHT
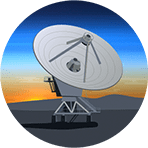
Expanding perspectives every month.
- Curated Curricula
- Competency Models
- Development Frameworks
- Maximize Your Learning Experience
- Systems Engineering Leadership Program (SELP)
- Accreditations & Affiliations
- Defense Acquisition University
- Course Support
- Registration Overview
- Virtual Backgrounds
- INSIGHT Publication
- Apollo Era Resources
- Case Studies
- Knowledge Capture and Transfer
- Knowledge Sharing Tools
- NASA Knowledge Community
- Shuttle Era Resources
- Spotlight on Lessons Learned Series
- Lessons Learned Lifecycle and Highlights
- Project Management Courses
- Program Management Series
- NPR 7120.5 Revision F Rollout Briefing (NASA Only)
- NPR 7120.5 Tailoring Resources
- NASA FAC-P/PM Certification Program
- Office of the Chief Engineer Handbooks
- Relevant Documents
- Affiliations
- Small Steps, Giant Leaps Podcast
- Quick Webinars
- On-Demand Courses
- Project Knowledge Expo
- NASA Knowledge on Video
- Knowledge Inventory
- Technical Authority
Risk Management I (APPEL-vRM I)
PARTICIPANT TESTIMONIALS
Skill Level: Open to all.
This course provides an introduction to both the Risk Informed Decision Making (RIDM) and Continuous Risk Management (CRM) in the context of the policies and recommended practices that guide their application.
This course is designed for NASA’s technical workforce, including systems engineers and project personnel who seek to develop the competencies required to succeed as a leader of a project team, functional team, or small project.
Project Management Competencies
- PM 1.2 - Risk Management
Systems Engineering Competencies
- SE 3.4 - Technical Risk Management
Upon completion of this course, participants will be able to:
- Describe NASA’s risk management policy.
- Analyze NASA risk management processes as defined by the Risk Management Handbook.
- Explain individual roles and responsibilities for identification and management of risks.
- Explain the purpose and application, and illustrate the steps and implementation of risk-informed decision making.
- Explain the relationships between project decisions and project risks.
- Explain the purpose and application, and illustrate the steps and implementation of continuous risk management.
- Identify sources of risks and circumstances that may result in risks.
- Evaluate the risk management requirements for a project or program.
- Formulate an appropriate risk management approach in a risk management plan.
Schedule & Registration
Click here to express interest.
Registration Question? Contact the training specialist below for assistance.
Susan Largent [email protected] (321) 861-1572
EXTERNAL CERTIFICATION CREDIT
- PMI Talent Triangle® Suggested PDUs
- 7 Technical Project Mgt.
- 0 Leadership
- 0 Strategic & Business Mgt.
- 0.7 IACET CEUs
- 7 FAC-P/PM CLPs
- PMI® PDUs (R.E.P #1895)
- Course ID: NASA APPEL - RM1
About the Instructor(s)
To be announced, curriculum lead.
Curriculum Question? Contact the curriculum lead below for assistance.
Diana Wehrell-Grabowski [email protected]
Explore the Course Catalog

IMAGES
VIDEO
COMMENTS
Risk Informed Decision Making (RIDM) SMA-RISK-OSMA-0013 This course covers in detail one of the two processes comprised in the NASA Risk Management process required by NPR 8000.4.Its content is based on the NASA RIDM Handbook and it provides detailed guidance for implementing the RIDM requirements of NPR 8000.4, with a specific focus on programs and projects in the Formulation Phase.
Approach to Risk Management: NASA Case Study Jeevan S. Perera, PhD, JD National Aeronautics and Space Administration Lyndon B. Johnson Space Center Houston, Texas 77058. 2 Agenda NASA's Current Environment Space Exploration Systems Short Video NASA's Risk Management Paradigm
May 11, 2011. APPEL News Staff. May 10, 2011 Vol. 4, Issue 3. The events leading up to the Deepwater Horizon accident offers several cautionary lessons for NASA. Offshore oil drilling is complex work that employs state-of-the art technology in an extremely dynamic environment. It involves using a drilling rig to penetrate the ocean floor, and ...
This study discusses the approach of NASA managers in the assessment of risk in three critical decisions: the Apollo 8 decision to orbit the Moon in 1968, the servicing of the Hubble Space Telescope in 1993, and the privitization of the Space Shuttle in the latter 1990s. 14. SUBJECTTERMS Apollo, Space Shuttle,Hubble Space Telescope, risk assessment
(Applicable NPRs include 7120.5 and 8000.4, which address project management and risk management, respectively.) Specific attention should be given to how risks associated with individual pieces of hardware/software are aggregated with risks of other subsystems to reflect total mission risk. Consult the lesson learned for complete lists.
i NASA/SP-2011-3422 Version 1.0 NASA Risk Management Handbook National Aeronautics and Space Administration NASA Headquarters Washington, D.C. 20546
Risk Informed Decision Making (RIDM) SMA-RISK-OSMA-0013 This course covers in detail one of the two processes comprised in the NASA Risk Management process required by NPR 8000.4.Its content is based on the NASA RIDM Handbook and it provides detailed guidance for implementing the RIDM requirements of NPR 8000.4, with a specific focus on programs and projects in the Formulation Phase.
The Big Dig is also famous for cost increases. Its initial estimated cost was $2.56 billion. Estimates increased to $7.74 billion in 1992, to $10.4 billion in 1994, and, finally, $14.8 billion in 2007—more than five times the original estimate. The reported reasons for the cost escalation included inflation, the failure to assess unknown ...
The following case study exercise provides lessons learned from the development and operations of the Space Shuttle Program (SSP). It is intended to highlight key transferable aspects of risk management, which may vary slightly from a particular case study to the next. Transferable principles include the identification of risks, evaluation of risks, mitigation of risks, risk trades, and risk ...
Figure 1. Intersection of NPR 8000.4 with Program and Project and Domain-Specific Directives and Requirements. 1.1.4 This NPR supports NASA's security objectives at project, program, and institutional infrastructure level, including both physical security and cybersecurity activities.
Describe NASA's risk management policy. Analyze NASA risk management processes as defined by the Risk Management Handbook. Explain individual roles and responsibilities for identification and management of risks. Explain the purpose and application, and illustrate the steps and implementation of risk-informed decision making.
Case Studies in NASA High-Technology Risk Assessment and Management This study discusses the approach of NASA managers in the assessment of risk in three critical decisions: the Apollo 8 decision to orbit the Moon in 1968, the servicing of the Hubble Space Telescope in 1993, and the privitization of the Space Shuttle in the latter 1990s.
Twenty-five years after project initiation, billions of dollars in cost overruns and a series of technical failures, spectacular, transcendent images from deep space are beaming back to Earth, visible evidence of the overwhelming success of the James Webb Space Telescope (JWST). Initially conceived as the Next Generation Space Telescope (NGST ...
developed written case studies, some of which include companion videotaped interviews with primary participants featured in the corresponding case study. The former CKO of the Human Exploration and Operations Mission Directorate (HEOMD), Dr. David Lengyel, collected a variety of case studies from across NASA, primarily focused on risk management.
Fosters decision-making thinking (NASA, 2008). This paper has presented a case study about a very complex project: the engineering design, procurement, and construction of a 400,000 barrel oil refinery. We hope that you have learned about risk on complex projects and mitigation of risk in the design and procurement phases.
Terrestrial protected areas (TPAs) are vital for ecosystem conservation and can easily be threatened by human activities or natural disasters. The causes and impacts of human intervention on TPAs have been documented in previous studies, while assessing the exposure and vulnerability of TPAs to natural hazards, particularly wind hazards, is scarce. This study developed a model for assessing ...