
An official website of the United States government
The .gov means it’s official. Federal government websites often end in .gov or .mil. Before sharing sensitive information, make sure you’re on a federal government site.
The site is secure. The https:// ensures that you are connecting to the official website and that any information you provide is encrypted and transmitted securely.
- Publications
- Account settings
Preview improvements coming to the PMC website in October 2024. Learn More or Try it out now .
- Advanced Search
- Journal List
- HHS Author Manuscripts


The case for case-cohort: An applied epidemiologist’s guide to re-framing case-cohort studies to improve usability and flexibility
Katie m. o’brien.
1 Epidemiology Branch, National Institute of Environmental Health Sciences, Research Triangle Park, NC
Kaitlyn G. Lawrence
Alexander p. keil.
2 Department of Epidemiology, Gillings School of Global Public Health, University of North Carolina at Chapel Hill, Chapel Hill NC
Associated Data
When research questions require the use of precious samples, expensive assays or equipment, or labor-intensive data collection or analysis, nested case-control or case-cohort sampling of observational cohort study participants can often reduce costs. The two study designs have similar statistical precision for addressing a singular research question, but case-cohort studies have broader efficiency and superior flexibility. Despite this, case-cohort designs are comparatively underutilized in the epidemiologic literature. Recent advances in statistical methods and software have made analyses of case-cohort data easier to implement, and advances from casual inference, such as inverse probability of sampling weights, have allowed the case-cohort design to be used with a variety of target parameters and populations. To provide an accessible link to this technical literature, we give a conceptual overview of case-cohort study analysis with inverse probability of sampling weights. We show how this general analytic approach can be leveraged to more efficiently study subgroups of interest or disease subtypes, or to examine associations independent of case status. A brief discussion of how this framework could be extended to incorporate other related methodologic applications further demonstrates the broad cost-effectiveness and adaptability of case-cohort methods for a variety of modern epidemiologic applications in resource-limited settings.
Introduction
When designing epidemiologic studies, we are often confronted with tradeoffs between statistical precision, measurement accuracy, and cost. 1 Some common examples where such trade-offs occur include studies involving expensive measurement of genetic, physiological or environmental exposure markers, including the rapidly growing “omics” fields of metabolomics, epigenomics, and proteomics. Other types of cost constraints include human capital, which may be required for complex sample preparation, records abstraction, or analysis time, and depletion of limited resources, as could occur if a certain assessment required all of an available sample.
When new data collection is needed, prospective cohort studies are often considered the gold standard of observational research. Such cohort studies are often assembled with multiple research topics in mind (e.g., the Nurses’ Health Studies 2 , the Southern Community Cohort Study, 3 the Sister Study 4 ), but some specific research questions may require the nesting of compact designs within the larger study due to cost constraints. One such commonly used approach is the nested case-control design. In such a design, individuals from the cohort with the outcome of interest are identified; this is typically followed by a selection of a referent group (the “controls”) from among those in the cohort who do not have the specified outcome. Alternatively, case-cohort designs use a random sample of the entire cohort as the comparison group for the cases. 5
Here, we summarize the benefits of case-cohort designs in terms of flexibility and cost-effectiveness, and renew a call for their increased usage. Although most of the points we raise here are not novel, 6 – 11 improvements in analytic tools and epidemiologic methods have rendered case-cohort designs more attractive in the time since previous tutorials were published. While recent technical literature provides a solid framework for generalizing the analysis of case-cohort designs, our primary aim is to provide an accessible translation of this literature for applied researchers. Specifically, we focus on how case-cohort designs can be understood within a general sampling framework where approaches like inverse probability weighting can be used to re-weight the study sample to represent a cohort study. For the applied researcher, these recent conceptual and software advances imply that case-cohort designs can be utilized and adapted to efficiently and flexibly address a broader array of study questions than may be currently appreciated.
We also describe how this general sampling framework can be used to extend standard case-cohort designs for assessing subgroup differences (i.e., effect measure modification or disease subtype differences) and to increase study-wide efficiency, while still maintaining compatibility with other epidemiologic study designs for pooled or meta-analyses. We also highlight examples of how case-control studies can be used to estimate population causal effects, which suggest strong advantages for the case-cohort design for making population-based inferences using modern causal inferential approaches.
Cohort studies and the need for more cost-efficient designs
Experiments and clinical trials can be used to assess how a limited number of exposures are associated with one or more outcomes. Case-control studies, in contrast, are usually limited to a single outcome but can consider multiple exposures. Cohort studies tend to offer more flexibility than the other designs, allowing researchers to assess the relationship between many different exposure-outcome combinations through the collection of data on a large number of variables (exposures, covariates, outcomes) at enrollment and during follow-up. Cohorts are usually sampled so that they represent a specific, identifiable population (e.g. women who identify as Black in the US or US adults over age 65 with no history of cancer), and often include the collection of environmental samples (e.g. house dust, air quality measures) or biospecimens (e.g. blood, urine, saliva, toenails, placenta) for genetic and/or molecular epidemiology studies.
When assessing relationships between exposures and health outcomes that occur at specific, known event times, investigators often report hazard ratios (HRs) and confidence intervals for the association of interest. HRs are often estimated in a Cox proportional hazards model via maximum partial likelihood. 12 Such models are semi-parametric, meaning that under the assumption that the estimated parameters are constant over time, a baseline hazard distribution need not be specified to obtain valid HRs. Cox models can be implemented in all standard statistical software packages and can easily accommodate additional covariates, including time-varying ones, and different time scale specifications (e.g., age time, calendar time, or time on study). Accelerated failure time models can be a useful parametric alternative for assessing time-to-event outcomes. 13 , 14 If investigators are less interested in when, specifically, the outcome occurs, it is also sometimes conceivable to treat study outcomes as binary measures at a fixed time point (e.g. diagnosis within five years of enrollment), and use parametric regression to estimate a contrast of cumulative risks, including risk differences, risk ratios, or odds ratios (ORs).
Each of these estimands could potentially be obtained through efficient, retrospective sampling and measurement designs that minimize random error. However, in epidemiologic analyses, systematic bias is often a greater concern than random error. Broadly, this concern has led to a preference for “prospective” cohort designs, where exposure and other relevant confounders can be assessed prior to when the outcome(s) occur. More specific advantages of prospective cohort studies include avoidance of reverse causality (when the symptoms of the disease or the disease itself contributes to changes in exposure or its measurement) and recall bias (differential reporting of exposure according to case status). 8 , 15 Prospective cohort studies may still be prone to selection bias, which occurs when the included participants are different from the target population, or when certain individuals have missing or censored covariate or outcome data. However, because all included participants are recruited prior to determining exposure, outcome, and covariate status, there is less concern that these biases are differential between comparison groups.
Given these advantages, there is a clear need for study designs that can make use of prospectively collected cohort data, even when financial or time constraints mean that it is not possible to get all of the desired information from everyone in the sample.
Nested case-control studies
Nested case-control studies were developed to address this need, yet retain a satisfactory level of statistical precision. Here we defined “nested case-control studies” to mean studies that include individuals specifically selected because they (1) already experienced the outcome of interest (“cases”), or (2) are known to be unaffected by the outcome of interest at the time of sampling (“controls”). The term “nested” is used to indicate that the sample is embedded within a larger prospective cohort and that the temporal relationship between exposure and outcome is thereby preserved.
Controls are often identified via risk-set sampling, 15 such that at the age/time each case that develops, one or more non-cases currently contributing person-time are selected as matched control(s). Alternatively, investigators can chose a “cumulative” design, 15 where controls are selected as individuals who do not develop the outcome over a fixed time period of interest (e.g. those who have not developed the outcome before age 50, or within the five years since enrollment). In both cases, individuals who develop the outcome at future time points are eligible to serve as controls.
Data from nested case-control studies are typically analyzed using conditional or unconditional logistic regression with adjustment for covariates, as needed. If risk-set sampling is used, an incidence rate ratio can be estimated using conditional logistic regression. 15 In unmatched scenarios, incidence odds ratio or cumulative incidence odds ratio can be estimated using unconditional logistic regression, with the latter approximating cumulative incidence ratios when the outcome is rare. It has also long been known that such studies can also be used to estimate cumulative incidence (and thus risk differences), 16 but this approach relies on external data on population risk that may not be available. Though initial sample selection can be complex depending on the matching protocol, logistic regression analyses are simple to implement and can be completed using standard statistical software. 17 – 19
Case-cohort studies
First developed and described by Prentice, 5 , 20 a case-cohort study is another nested alternative to full cohort analyses that cuts costs but preserves the temporal relationship between exposure and outcome. Here we define case-cohort studies to be those that include (1) a sample of individuals from the cohort (up to 100%) 21 , 22 who have experienced the outcome of interest (“cases”) and (2) a (possibly overlapping) sample of individuals randomly selected from among the members of the full cohort observed at baseline (the “sub-cohort”) ( Figure 1A ).

Visualization of case-cohort designs assuming a time-on-study time scale. (A) The case-cohort study includes (1) a sample of individuals from the cohort who have experienced the outcome of interest (“cases”) and (2) a sample of individuals randomly selected from among the members of the full cohort observed at baseline (the “sub-cohort”). Selection into the sub-cohort is conducted without respect to future case status, meaning that some cases may be included by chance. (B) When considering an inverse probability of sampling weights approach to analyzing case-cohort data, cases not in the sub-cohort only contribute person-time just before their diagnosis (shown in solid black line), with the weight determined by the probability of selection as a case (weight=1 if 100% of cases are selected for inclusion). All individuals selected into the sub-cohort contribute person-time at risk, with the non-cases contributing for their entire follow-up period and cases contributing from enrollment until just before their diagnosis. The sub-cohort person-time is weighted based on the probability of being sampled into the sub-cohort (e.g. if sub-cohort is a 10% sample of cohort, weight=10).
Sum of case weights = total number of cases in cohort
Sum of weighted sub-cohort person-time = total person-time observed for cohort
Assuming no loss to follow-up in the cohort and no competing risks for the event(s) of interest, risk ratios can be directly estimated from case-cohort data. 15 However, such assumptions are often unreasonable, and Prentice et al. first described how Cox proportional hazards models could be applied to case-cohort data to estimate HRs that are asymptotically identical to those obtained from the full cohort, albeit with increased estimated variance and thus larger estimated confidence intervals (CIs). 5 , 20 , 23 The modification requires a “pseudo-likelihood” approach, which is essentially a weighted version of the partial-likelihood used for Cox proportional hazards regression. 23 Updates to the original approach have shown that the weights can be considered time-varying, with values determined by each participant’s case and sub-cohort status at each observed event time. 11 , 23 , 24
In a basic case-cohort design, the person-time among the sub-cohort is a random sample of all person-time in the full cohort. 5 , 20 Cases outside the sub-cohort have a weight of 0 until at the exact time they become a case. At that point, they are compared to all individuals at risk of the outcome at that same timepoint, with other cases contributing little 5 (or no 20 ) person-time at risk.
Alternatively, it is possible to re-weight the person-time contributions of case-cohort sample, so that the weighted “pseudo-population” represents the source cohort in terms of exposures, covariates, outcomes, and follow-up times. With T 0 representing time at start of follow-up, T Y representing time at event/censoring, and ε a very small number (smaller than the time units being measured), we describe a simple version of this weighting scheme in the first row of the Table . Corresponding data examples and SAS and R code are provided online ( https://github.com/ TBD) and as an appendix .
Weighting schemes for case–cohort designs.
W = weight;T 0 = Start of follow-up; T Y = Event time, ε= a very small number (less than a unit increase on your time scale)
This “simple” approach to case-cohort analysis applies if all cases are selected (sampling probability=100%) and the members of the sub-cohort are all selected with equal probability given by x%. Accordingly: 1) cases not in the sub-cohort would get a weight of 1.0 from just before their event time (T Y -ε) to their diagnosis (T Y ); 2) non-cases in the sub-cohort would be weighted as 1 x % for all of their follow-up time (T 0 to T Y ); and 3) cases in the sub-cohort would get a weight of 1 x % from the start of follow-up until just before their event time ((T 0 to T Y -ε), and then a weight of 1.0 at the exact time of their event (T Y -ε to T Y ).
The key to this approach is understanding the weighting of the cases in the sub-cohort, who have their person-time split between into two separate observations in the modified data set (shown visually in Figure 1B ). If everyone in the sub-cohort received the inverse probability of selection weights ( 1 x % ) for their entire follow-up period, the sub-cohort would itself be weighted back to equal the full cohort, including all of the cases in the sub-cohort. Therefore, the number of cases in the sub-cohort would be weighted to approximately equal the number of true cases in the full cohort. As such, any additional data from cases outside the sub-cohort would overcount the total number of cases. By assigning all of the cases a weight of exactly 1.0 at the time of their event, the weighting scheme described here ensures that each case is only counted once and that all cases contribute equally. Their contribution prior to their event time is determined by whether they also contributed to the sub-cohort. Of note, if the cases were selected with a probability of less than 100%, either by design or as a result of missing data, the weight for cases at the time of their selection could be adjusted accordingly (e.g., a 20% sample would be weighted as 1/0.20=5.0).
Case-cohort data were initially difficult to analyze with standard software. Barlow et al. 23 and Langholz and Jiao 25 provided SAS code, but the robust variance calculations needed to account for the atypical data structure and/or weighting scheme required custom coding, and Cox model routines in available software packages could not always accommodate weights. However, changes to PHREG procedure to allow sandwich variance estimates and weighting (SAS versions 8.2 and 9.0, respectively), have made implementation much more straight-forward and adaptable. 11 , 23 , 25 Similar capabilities have also become available in numerous other statistical software packages, including R and Stata.
A comparison: Nested case-control versus case-cohort
Both nested case-control and case-cohort study designs can yield precision approaching what is seen for cohort designs, 5 , 8 , 20 , 26 while reducing costs incurred by measurement. Because of the previously stated software issues, case-cohort data have historically been more difficult to analyze. However, as this is no longer the case, case-cohort designs have several clear advantages compared to nested case-control designs.
A primary advantage of case-cohort studies is that the selected sub-cohort can easily be used as a comparison group for many different outcomes, or, in some circumstances, as a study sample on its own. For example, researchers could design a case-cohort study of breast cancer and measure the concentrations of a physiological marker in baseline blood samples collected from both (future) breast cancer cases and a random sub-cohort. 27 The same sub-cohort could then be used again as a comparison group for a study of the association between the same exposure and ovarian cancer, or perhaps another chronic disease such as diabetes or stroke. Additionally, the same researchers could use data from just the sub-cohort to examine the relationship between the environmental contaminants and a common outcome, such as obesity or hypertension, or to look at whether the concentrations of the measured contaminant varied by race/ethnicity or occupation.
In contrast, establishing a common control group for multiple outcomes with a nested case-control design is more challenging. For example, it might be reasonable to have a control group that is free of two rare diseases (e.g. ovarian cancer and pancreatic cancer), but each additional exclusion criterion diminishes the representativeness of exposure distributions within the control group, which biases the OR estimated from the nested case-control sample relative to the OR estimate from the full cohort. The use of matching for efficiency would limit flexibility and generalizability even further. Methods have been developed to re-weight or combine control groups to create a more appropriate referent group, 28 – 30 but such approaches often have specific data requirements and may be difficult to implement with standard software.
Both the case-control and the case-cohort study designs make it easy to layer exposure measurements on top of one another, given sufficient banked samples and/or consistent storage and analytical techniques. For example, if investigators are interested in studying the role of genetic factors as they relate to an exposure, an outcome, or their interaction, it is fairly straight-forward to genotype the same sample that was selected for a prior nested case-control or case-cohort study. 27 , 31 , 32 In doing this, investigators using case-cohort sampling would open up a wide range of genetic-related research opportunities within the sub-cohort, 33 a sample fully representative of the full cohort, while the control group for the nested case-control sample would have less utility and interpretability.
In a comparison of several different approaches to analyzing nested case-control and case-cohort data, Kim found that when inverse probability of selection weights were incorporated, the designs had similar statistical power. 9 Langholz and Thomas 26 previously showed that a single nested case-control study may be more efficient than a single case-cohort study of the same sample size, particularly in the presence of late entry and right censoring. The rarity of the outcome of interest also matters, with nested case-control studies having increasingly greater statistical precision than case-cohort studies of the same total sample size as the outcome becomes more common. 15
However, this does not account for the potential efficiency gains for case-cohort studies when studying alternative outcomes, where case-cohort designs may require identifying only new cases while nested case-control designs would generally necessitate identifying both new cases and controls. Further, though not covered in detail here, additional increases in statistical power could be gained by including auxiliary data from participants not selected into the sub-cohort, even if those participants were missing data on crucial covariates, 34 – 36 or by jointly considering multiple outcomes. 37 – 39 Some previous work suggests nested case-control studies may be better suited for studying biomarkers sensitive to batch, storage, and freeze/thaw cycle effects, 40 but nested case-control studies with alternative designs could be subject to similar biases. 29
The increasing advantages of case-cohort designs
Despite these potential benefits in terms of optimizing study resources, case-cohort studies have not permeated epidemiologic study design as fully as nested case-control studies. As an update to the numbers reported by Barlow et al., 23 a June 2021 MEDLINE keyword search indicated that “nested case-control” studies were much more common (9,364 entries, including 6,090 since January 2010), than “case-cohort” studies (2,192 entries, including 1,611 since January 2010).
This preference for nested case-control studies is likely due, at least in part, to perceived difficulties in understanding and implementing case-cohort studies, particularly if they go beyond the simplest of applications. Now that the software/programming concerns have been addressed through new software routines, we offer some additional thoughts on how to adapt case-cohort to more complex scenarios, including what we are calling “covariate-stratified case-cohort designs” and “outcome-stratified case-cohort designs”.
Stratified case-cohort sampling to enhance efficiency for subgroup analyses
Case-cohort designs can also be easily adapted to focus resources on the study of certain covariate-defined subgroups or disease types. We present examples of these stratified case-cohort designs in the Table , with the basic framework following what was previously presented for the simple scenario of a 100% case sample with an x% random sample of the cohort into the sub-cohort. The elements are flexible and adaptable, as long as the selection probabilities are known and their inverses are included as weights in the regression models. 7 , 10 , 11
A covariate-stratified case-cohort design could be used to maximize precision for groups of particular interest (e.g. Black or Latinx individuals in environmental studies where there are documented exposure disparities, 41 or infant boys when studying endocrine disruptors and there are expected differences in effect size 42 ). Such a design would involve over-sampling cohort members who are in the selected subgroups, rather than just taking a random sample of the full cohort. As shown in the Table , if there are two sampling groups “A” and “B”, the sampling weights (x A % and x B %, respectively) are factored in when determining the weights for the sub-cohort members. This includes both cases (weight = 1 x A % or weight = 1 x B % from T 0 to T Y -ε) and non-cases (weight = 1 x A % or weight = 1 x B % from T 0 to T Y ). If 100% of the cases are included, then the case weights do not change (weight=1.0 from T Y -ε to T Y ).
If the goal is to disproportionately sample one disease type over another, an outcome-stratified case-cohort design may be more appropriate. For example, researchers might want to use an outcome-stratified case-cohort design to include all incident cases of a rare subtype (e.g., estrogen receptor-negative breast cancer), but only a sample of a more common subtype (estrogen receptor-positive breast cancer). Here, the sampling weights for the sub-cohort would be the same as the simplest version (weight = 1 x % from T 0 to T Y for non-cases or T 0 to T Y -ε for cases), but the weights for cases would change depending on the outcome type. If 100% of those with the type I outcome were selected, but only y% of those with the type II outcome, type I cases (both in and out of the sub-cohort) would be given weights of 1.0 from T Y -ε to T Y and type II cases would be given weights of 1 ( y % + x % − ( x % * y % ) ) from T Y -ε to T Y ).
Provided that sampling probabilities are known, case-cohort designs can also incorporate both covariate-stratified and outcome-stratified elements together (described in the 4 th row of the Table ). It could also be expanded to include more than two strata of covariates or outcomes, and it is not limited to any one group being sampled with 100% probability. As a simple data checking step, investigators can construct weighted frequency tables using only the case-cohort sample and show that the person-time and case counts in the weighted sample have approximately the same distribution of exposure, covariates, and outcomes as the original eligible cohort (within sampling error).
Re-imagining the data for assessing case-independent research questions
As previously mentioned, an additional strength of case-cohort studies over nested case-control studies is the ability to use the sub-cohort as an independent sample that is fully representative of the original full cohort. For example, investigators might want to look at the cross-sectional relationship between a physiological biomarker and epigenetic modifications measured in the selected individuals. Both prospective and cross-sectional studies could be accommodated, with weighting only needed if covariate-stratified approaches had been utilized.
If looking to increase statistical precision, it is also possible to include the cases when assessing research questions not directly related to the outcome (for examples, see Lawrence et al. 43 and Kresovich et al. 44 ) To ensure that the measured association is “case-independent”, weights would be used to account for the inverse probability of selection into the study. However, unlike the previous examples, we cannot use event times to define the weights, and must instead assign them based on case or non-case status at a single time point. In other words, selected cases would be representative of all cases that existed at the time the case-cohort sample was selected (weight of 1 v % , if v% of cases were selected; Table ). Non-cases would be representative of all the individuals who had not yet become cases at the time the case-cohort sample was selected (weight = 1 z % , if z% of non-cases were selected). Though lower powered than an analysis that included all individuals weighted equally but with adjustment for case status (e.g. O’Brien et al. 32 , White et al. 45 ), an inverse-probability-of-sampling weighted analyses would more accurately estimate effects in the full cohort, if that is the desired target population.
Further adaptations in causal inference with case-cohort designs
Methods for correcting for selection bias and time-varying confounding in case-cohort samples have already been developed in ways that are compatible with the framework presented here. 46 – 48 By allowing the case-cohort to be described as a weighted version of a full cohort study, this places case-cohort designs in the context of many modern approaches to epidemiologic data analysis that include weighting, or in which special considerations for weight have already been made. This includes including estimation of absolute risk measures, imputation, quantitative bias analyses, 49 assessment of complex mixtures, 41 , 50 inverse probability of treatment weighting, 51 , 52 and generalizability and transport. 53 , 54
Notably, the preceding examples are mainly questions pertinent to causal inference in prospective study designs. When population odds of the study outcome is known, it may be possible to estimate population cumulative risks from case-control data. 55 However, because of the outcome dependent sampling scheme, understanding how covariates develop over time is sometimes challenging in case-control studies. Because case-cohort studies include a sample of the cohort, this design potentially opens up questions and methods that specifically leverage time-varying covariates, such as questions about mediation or methods such as the parametric g-formula 56 , 57 or doubly-robust estimation. 58 Details of such applications need further research and development and are beyond the scope of this review.
Compatibility of case-cohort and nested case-control
Despite what we see as clear arguments in favor of case-cohort over nested case-control designs, the reality is that many previous investigations of this type have already been sampled and analyzed as nested case-control studies. This can lead to issues of compatibility between the two, whether comparing effect estimates informally or formally (i.e. meta-analysis), or attempting to pool data for combined analysis.
Nested case-control data can be used to estimate HRs if the cases and controls if risk-set sampling is used. As mentioned earlier, assuming no loss to follow-up and no competing risks, risk ratios or ORs can be directly estimated from case-cohort data. 15 In other words, under certain assumptions, a case-cohort study can be thought of as a nested case-control study done over a fixed period of interest (e.g. 5-year risk of developing a disease). Importantly, without explicit matching on time, exposure assessments for both cases and non-cases should be based their status at some comparable timepoint to ensure exchangeability 59 and avoid being subject to differential misclassification by case status, as could occur with exposures prone to recall bias, reverse causation, or confounding by age or time.
Case-cohort designs are an established but little-utilized tool for answering epidemiologic questions that require prospective ascertainment of cases and expensive measurements. Recent software developments have made case-cohort studies simpler to analyze and the design offers clear benefits in flexibility and general efficiency compared to more traditional nested case-control approaches. With this inverse probability of sampling weights approach, we provide tools for improved implemention of case-cohort studies, including adaptions to allow for better representation of certain subgroups or disease types, and extensions to address research questions beyond their initial purpose. The proposed frameworks also offer great potential for further methodological application and development in the context of modern causal inference-based approaches to epidemiologic data.
Supplementary Material
This work was supported by the Intramural Research Program of the National Institutes of Health (National Institute of Environmental Health Sciences).
Abbreviations:
- En español – ExME
- Em português – EME
Case-control and Cohort studies: A brief overview
Posted on 6th December 2017 by Saul Crandon
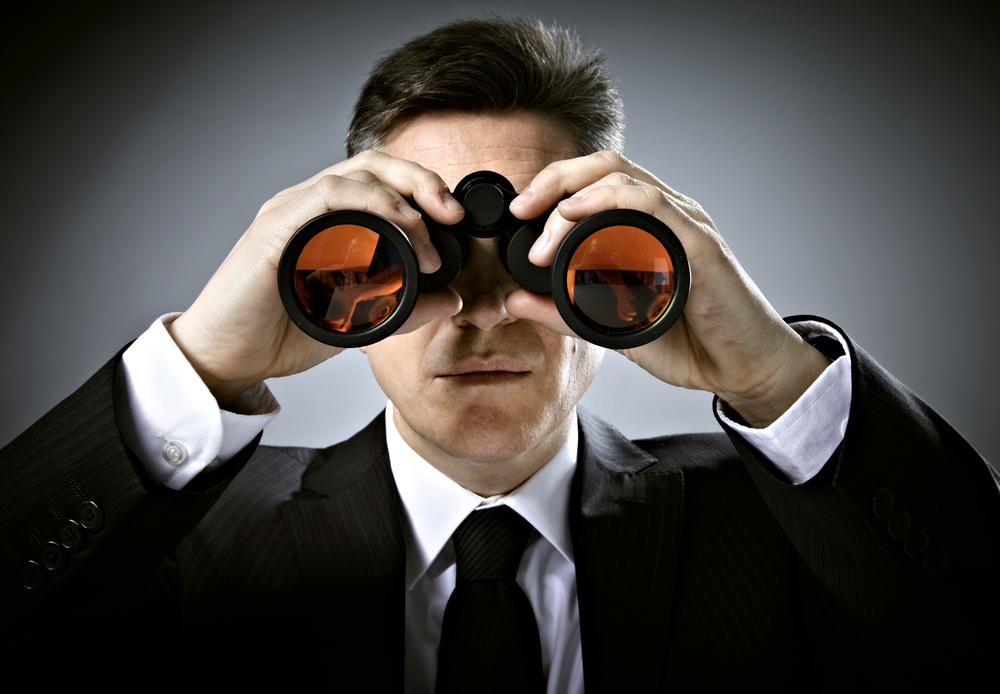
Introduction
Case-control and cohort studies are observational studies that lie near the middle of the hierarchy of evidence . These types of studies, along with randomised controlled trials, constitute analytical studies, whereas case reports and case series define descriptive studies (1). Although these studies are not ranked as highly as randomised controlled trials, they can provide strong evidence if designed appropriately.
Case-control studies
Case-control studies are retrospective. They clearly define two groups at the start: one with the outcome/disease and one without the outcome/disease. They look back to assess whether there is a statistically significant difference in the rates of exposure to a defined risk factor between the groups. See Figure 1 for a pictorial representation of a case-control study design. This can suggest associations between the risk factor and development of the disease in question, although no definitive causality can be drawn. The main outcome measure in case-control studies is odds ratio (OR) .
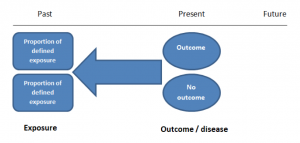
Figure 1. Case-control study design.
Cases should be selected based on objective inclusion and exclusion criteria from a reliable source such as a disease registry. An inherent issue with selecting cases is that a certain proportion of those with the disease would not have a formal diagnosis, may not present for medical care, may be misdiagnosed or may have died before getting a diagnosis. Regardless of how the cases are selected, they should be representative of the broader disease population that you are investigating to ensure generalisability.
Case-control studies should include two groups that are identical EXCEPT for their outcome / disease status.
As such, controls should also be selected carefully. It is possible to match controls to the cases selected on the basis of various factors (e.g. age, sex) to ensure these do not confound the study results. It may even increase statistical power and study precision by choosing up to three or four controls per case (2).
Case-controls can provide fast results and they are cheaper to perform than most other studies. The fact that the analysis is retrospective, allows rare diseases or diseases with long latency periods to be investigated. Furthermore, you can assess multiple exposures to get a better understanding of possible risk factors for the defined outcome / disease.
Nevertheless, as case-controls are retrospective, they are more prone to bias. One of the main examples is recall bias. Often case-control studies require the participants to self-report their exposure to a certain factor. Recall bias is the systematic difference in how the two groups may recall past events e.g. in a study investigating stillbirth, a mother who experienced this may recall the possible contributing factors a lot more vividly than a mother who had a healthy birth.
A summary of the pros and cons of case-control studies are provided in Table 1.
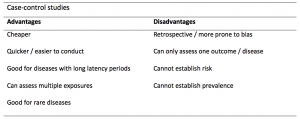
Table 1. Advantages and disadvantages of case-control studies.
Cohort studies
Cohort studies can be retrospective or prospective. Retrospective cohort studies are NOT the same as case-control studies.
In retrospective cohort studies, the exposure and outcomes have already happened. They are usually conducted on data that already exists (from prospective studies) and the exposures are defined before looking at the existing outcome data to see whether exposure to a risk factor is associated with a statistically significant difference in the outcome development rate.
Prospective cohort studies are more common. People are recruited into cohort studies regardless of their exposure or outcome status. This is one of their important strengths. People are often recruited because of their geographical area or occupation, for example, and researchers can then measure and analyse a range of exposures and outcomes.
The study then follows these participants for a defined period to assess the proportion that develop the outcome/disease of interest. See Figure 2 for a pictorial representation of a cohort study design. Therefore, cohort studies are good for assessing prognosis, risk factors and harm. The outcome measure in cohort studies is usually a risk ratio / relative risk (RR).
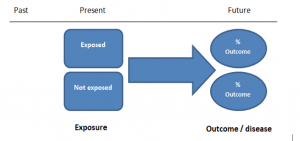
Figure 2. Cohort study design.
Cohort studies should include two groups that are identical EXCEPT for their exposure status.
As a result, both exposed and unexposed groups should be recruited from the same source population. Another important consideration is attrition. If a significant number of participants are not followed up (lost, death, dropped out) then this may impact the validity of the study. Not only does it decrease the study’s power, but there may be attrition bias – a significant difference between the groups of those that did not complete the study.
Cohort studies can assess a range of outcomes allowing an exposure to be rigorously assessed for its impact in developing disease. Additionally, they are good for rare exposures, e.g. contact with a chemical radiation blast.
Whilst cohort studies are useful, they can be expensive and time-consuming, especially if a long follow-up period is chosen or the disease itself is rare or has a long latency.
A summary of the pros and cons of cohort studies are provided in Table 2.
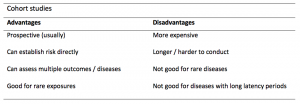
The Strengthening of Reporting of Observational Studies in Epidemiology Statement (STROBE)
STROBE provides a checklist of important steps for conducting these types of studies, as well as acting as best-practice reporting guidelines (3). Both case-control and cohort studies are observational, with varying advantages and disadvantages. However, the most important factor to the quality of evidence these studies provide, is their methodological quality.
- Song, J. and Chung, K. Observational Studies: Cohort and Case-Control Studies . Plastic and Reconstructive Surgery.  2010 Dec;126(6):2234-2242.
- Ury HK. Efficiency of case-control studies with multiple controls per case: Continuous or dichotomous data . Biometrics . 1975 Sep;31(3):643–649.
- von Elm E, Altman DG, Egger M, Pocock SJ, Gøtzsche PC, Vandenbroucke JP; STROBE Initiative. The Strengthening the Reporting of Observational Studies in Epidemiology (STROBE) statement: guidelines for reporting observational studies.  Lancet 2007 Oct;370(9596):1453-14577. PMID: 18064739.
Saul Crandon
Leave a reply cancel reply.
Your email address will not be published. Required fields are marked *
Save my name, email, and website in this browser for the next time I comment.
No Comments on Case-control and Cohort studies: A brief overview
Very well presented, excellent clarifications. Has put me right back into class, literally!
Very clear and informative! Thank you.
very informative article.
Thank you for the easy to understand blog in cohort studies. I want to follow a group of people with and without a disease to see what health outcomes occurs to them in future such as hospitalisations, diagnoses, procedures etc, as I have many health outcomes to consider, my questions is how to make sure these outcomes has not occurred before the “exposure disease”. As, in cohort studies we are looking at incidence (new) cases, so if an outcome have occurred before the exposure, I can leave them out of the analysis. But because I am not looking at a single outcome which can be checked easily and if happened before exposure can be left out. I have EHR data, so all the exposure and outcome have occurred. my aim is to check the rates of different health outcomes between the exposed)dementia) and unexposed(non-dementia) individuals.
Very helpful information
Thanks for making this subject student friendly and easier to understand. A great help.
Thanks a lot. It really helped me to understand the topic. I am taking epidemiology class this winter, and your paper really saved me.
Happy new year.
Wow its amazing n simple way of briefing ,which i was enjoyed to learn this.its very easy n quick to pick ideas .. Thanks n stay connected
Saul you absolute melt! Really good work man
am a student of public health. This information is simple and well presented to the point. Thank you so much.
very helpful information provided here
really thanks for wonderful information because i doing my bachelor degree research by survival model
Quite informative thank you so much for the info please continue posting. An mph student with Africa university Zimbabwe.
Thank you this was so helpful amazing
Apreciated the information provided above.
So clear and perfect. The language is simple and superb.I am recommending this to all budding epidemiology students. Thanks a lot.
Great to hear, thank you AJ!
I have recently completed an investigational study where evidence of phlebitis was determined in a control cohort by data mining from electronic medical records. We then introduced an intervention in an attempt to reduce incidence of phlebitis in a second cohort. Again, results were determined by data mining. This was an expedited study, so there subjects were enrolled in a specific cohort based on date(s) of the drug infused. How do I define this study? Thanks so much.
thanks for the information and knowledge about observational studies. am a masters student in public health/epidemilogy of the faculty of medicines and pharmaceutical sciences , University of Dschang. this information is very explicit and straight to the point
Very much helpful
Subscribe to our newsletter
You will receive our monthly newsletter and free access to Trip Premium.
Related Articles
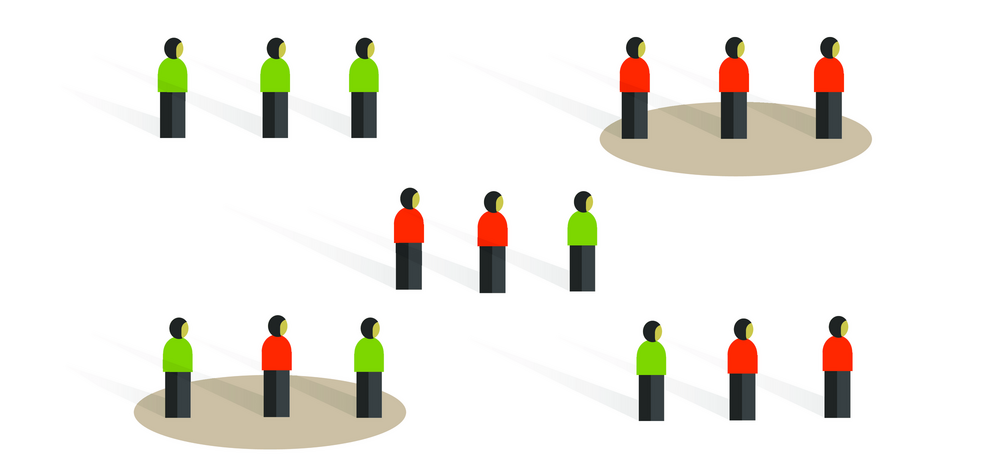
Cluster Randomized Trials: Concepts
This blog summarizes the concepts of cluster randomization, and the logistical and statistical considerations while designing a cluster randomized controlled trial.
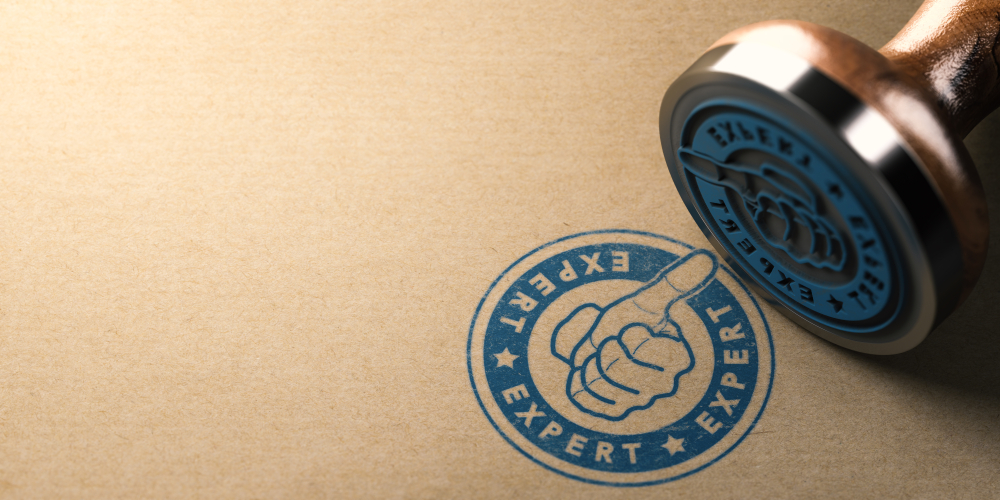
Expertise-based Randomized Controlled Trials
This blog summarizes the concepts of Expertise-based randomized controlled trials with a focus on the advantages and challenges associated with this type of study.
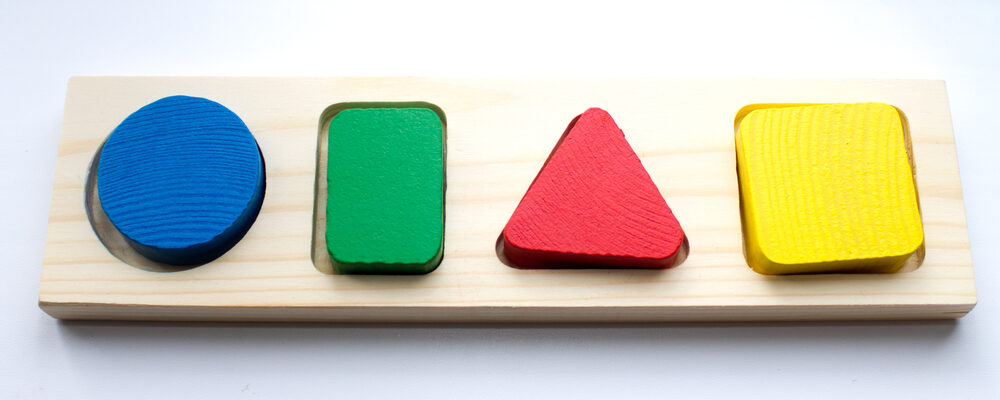
An introduction to different types of study design
Conducting successful research requires choosing the appropriate study design. This article describes the most common types of designs conducted by researchers.
- Research article
- Open access
- Published: 14 May 2020
Application of the matched nested case-control design to the secondary analysis of trial data
- Christopher Partlett ORCID: orcid.org/0000-0001-5139-3412 1 , 2 ,
- Nigel J. Hall 3 ,
- Alison Leaf 4 , 2 ,
- Edmund Juszczak 2 &
- Louise Linsell 2
BMC Medical Research Methodology volume 20 , Article number: 117 ( 2020 ) Cite this article
19k Accesses
14 Citations
3 Altmetric
Metrics details
A nested case-control study is an efficient design that can be embedded within an existing cohort study or randomised trial. It has a number of advantages compared to the conventional case-control design, and has the potential to answer important research questions using untapped prospectively collected data.
We demonstrate the utility of the matched nested case-control design by applying it to a secondary analysis of the Abnormal Doppler Enteral Prescription Trial. We investigated the role of milk feed type and changes in milk feed type in the development of necrotising enterocolitis in a group of 398 high risk growth-restricted preterm infants.
Using matching, we were able to generate a comparable sample of controls selected from the same population as the cases. In contrast to the standard case-control design, exposure status was ascertained prior to the outcome event occurring and the comparison between the cases and matched controls could be made at the point at which the event occurred. This enabled us to reliably investigate the temporal relationship between feed type and necrotising enterocolitis.
Conclusions
A matched nested case-control study can be used to identify credible associations in a secondary analysis of clinical trial data where the exposure of interest was not randomised, and has several advantages over a standard case-control design. This method offers the potential to make reliable inferences in scenarios where it would be unethical or impractical to perform a randomised clinical trial.
Peer Review reports
Key messages
A matched nested case-control design provides an efficient way to investigate causal relationships using untapped data from prospective cohort studies and randomised controlled trials
This method has several advantages over a standard case-control design, particularly when studying time-dependent exposures on rare outcomes
It offers the potential to make reliable inferences in scenarios where unethical or practical issues preclude the use of a randomised controlled trial
Randomised controlled trials (RCTs) are regarded as the gold standard in evidence based medicine, due to their prospective design and the minimisation of important sources of bias through the use of randomisation, allocation concealment and blinding. However, RCTs are not always appropriate due to ethical or practical issues, particularly when investigating risk factors for an outcome. If beliefs about the causal role of a risk factor are already embedded within a clinical community, based on concrete evidence or otherwise, then it is not possible to conduct an RCT due to lack of equipoise. It is often not feasible to randomise potential risk factors, for example, if they are biological or genetic or if there is a strong element of patient preference involved. In such scenarios, the main alternative is to conduct an observational study; either a prospective cohort study which can be complicated and costly, or a retrospective case-control study with methodological shortcomings.
The nested case-control study design employs case-control methodology within an established prospective cohort study [ 1 ]. It first emerged in the 1970–80s and was typically used when it was expensive or difficult to obtain data on a particular exposure for all members of the cohort; instead a subset of controls would be selected at random [ 2 ]. This method with the use of matching has been shown to be an efficient design that can be used to provide unbiased estimates of relative risk with considerable cost savings [ 3 , 4 , 5 ]. Cases who develop the outcome of interest at a given point in time are matched to a random subset of members of the cohort who have not experienced the outcome at that time. These controls may develop the outcome later and become a case themselves, and they may also act as a control for other cases [ 6 , 7 ]. This approach has a number of advantages compared to the standard case-control design: (1) cases and controls are sampled from the same population, (2) exposures are measured prior to the outcome occurring, and (3) cases can be matched to controls at the time (e.g. age) of the outcome event.
More recently, the nested case-control design has been used within RCTs to investigate the causative role of risk factors in the development of trial outcomes [ 8 , 9 , 10 ]. In this paper we investigate the utility of the matched nested case-control design in a secondary analysis of the ADEPT: Abnormal Doppler Enteral Prescription Trial (ISRCTN87351483) data, to investigate the role of different types of milk feed (and changes in types of milk feed) in the development of necrotising enterocolitis. We illustrate the use of this methodology and explore issues relating to its implementation. We also discuss and appraise the value of this methodology in answering similar challenging research questions using clinical trial data more generally.
ADEPT: Abnormal Doppler Enteral Prescription Trial (ISRCTN87351483) was funded by Action Medical Research (SP4006) and investigated whether early (24–48 h after birth) or late (120–144 h after birth) introduction of milk feeds was a risk factor for necrotising enterocolitis (NEC) in a population of 404 infants born preterm and growth-restricted, following abnormal antenatal Doppler blood flow velocities [ 11 ]. Consent and randomisation occurred in the first 2 days after birth. There was no difference found in the incidence of NEC between the two groups, however there was interest in the association between feed type (formula/fortifier or exclusive mother/donor breast milk) and the development of NEC. Breast milk is one of few factors believed to reduce the risk of NEC that has been widely adopted into clinical practice, despite a paucity of high quality population based data [ 12 , 13 ]. However, due to lack of equipoise it would not be ethical or feasible to conduct a trial randomising newborn infants to formula or breast milk.
With additional funding from Action Medical Research (GN2506), the authors used a matched nested case-control design to investigate the association between feed type and the development of severe NEC, defined as Bell’s staging Stage II or III [ 14 ], using detailed daily feed log data from the ADEPT trial. The feed type and quantity of feed was recorded daily until an infant had reached full feeds and had ceased parenteral nutrition, or until 28 days after birth, whichever was longest. Using this information, infants were classified according to the following predefined exposures:
Exposure to formula milk or fortifier in the first 14 days of life
Exposure to formula milk or fortifier in the first 28 days of life
Any prior exposure to formula milk or fortifier
Change in feed type (between formula, fortifier or breast milk) within the previous 7 days.
In the remainder of the methods section we discuss the challenges of conducting this analysis and practical issues encountered in applying the matched nested case-control methodology. In the results section we present data from different aspects of the analysis, to illustrate the utility of this approach in answering the research question.
Cohort time axis
For the main trial analysis, time of randomisation was defined as time zero, which is the conventional approach given that events occurring prior to randomisation cannot be influenced by the intervention under investigation. However, for the nested case-control analysis, time zero was defined as day of delivery because age in days was considered easier to interpret, and also it was possible for an outcome event to occur prior to randomisation. Infants were followed up until their exit time, which was defined by the first occurrence of NEC, death or the last daily feed log record.
Case definition
An infant was defined as a case at their first recorded incidence of severe NEC, defined as Bell’s staging Stage II or III [ 14 ]. Infants could only be included as a case once; subsequent episodes of NEC in the same infant were not counted. Once an infant had been identified as a case, they could not be included in any future risk sets for other cases, even if the NEC episode had been resolved.
Risk set definition
One of the major challenges was identifying an appropriate risk set from which controls could be sampled, whilst also allowing the analysis to incorporate the time dependent feed log data and adjust for known confounders. A diagnosis of NEC has a crucial impact on the subsequent feeding of an infant, therefore it was essential that the analysis only included exposure to non-breast milk feeds prior to the onset of NEC. A standard case-control analysis would have produced misleading results in this context, as infants would have been defined as a cases if they had experienced NEC prior to the end of the study period, regardless of the timing of the event in relation to exposure to non-breast milk. Using a matched nested case-control design allowed us to match an infant with a diagnosis of NEC (case) at a given point in time (days from delivery) to infants with similar characteristics (with respect to other important confounding factors), who had not experienced NEC at the failure time of the case. Figure 1 is a schematic diagram of this process. Each time an outcome event occurred (case), infants that were still at risk were eligible to be selected as a control (risk set). A matching algorithm was used to select a sample of controls with similar characteristics from this risk set. Infants selected as controls could go on to become a case themselves, and could also be included in the risk sets for other cases.
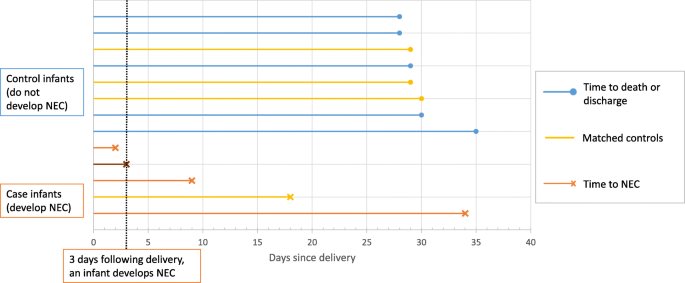
Schematic diagram illustrating the selection of controls from each risk set. Three days following delivery, an infant develops NEC. At this point, there are 11 infants left in the risk set. Four controls with the closest matching are selected, including one infant that becomes a future case on day 18
Selection of matching factors
An important consideration was the appropriate selection of matching factors as well as identifying the optimum mechanism for matching. Sex, gestational age and birth weight were considered to be clear candidates for matching factors, as they are all associated with the development NEC. Gestational age and birth weight in particular are both likely to impact the infant’s feeding and thus their exposure to non-breast milk feeds. Both gestational age and birth weight were matched simultaneously, because of the strong collinearity between gestational age and birth weight, illustrated in Fig. 2 . This was achieved by minimising the Mahalanobis distance from the case to prospective controls of the same sex [ 15 ]. That is, selecting the control closest in gestational age and birth weight to the case while taking into account the correlation between these characteristics.
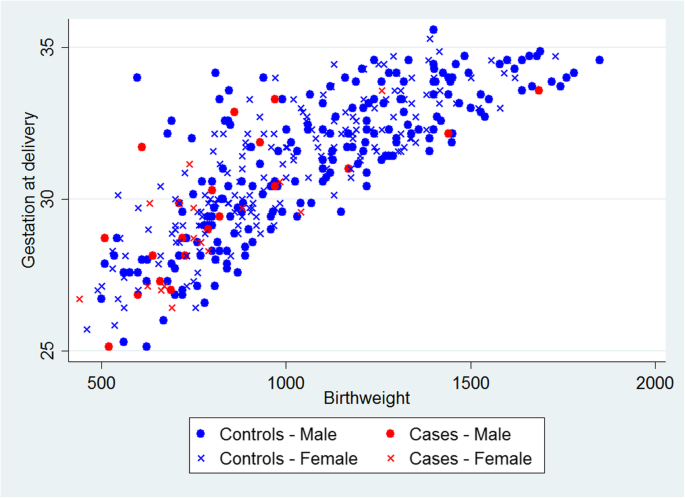
Scatterplot of birth weight versus gestational age for infants with NEC (cases) and those without (controls)
Typically, treatment allocation would be incorporated as a matching factor since in a secondary analysis it is a nuisance factor imposed by the trial design, which should be accounted for. However, in this example, the ADEPT allocation is associated with likelihood of exposure, since it directly influences the feeding regime. For example, an infant randomised to receive early introduction of feeds is more likely to be exposed to non-breast milk feeds in the first 14 days (44%) than an infant randomised to late introduction of feeds (23%). The main trial results also demonstrated no evidence of association with the outcome (NEC) and therefore there was a concern about the potential for overmatching. Overmatching is caused by inappropriate selection of matching factors (i.e. factors which are not associated with the outcome of interest), which may harm the statistical efficiency of the analysis [ 16 ]. Therefore, we did not include the ADEPT allocation as a matching factor, but we conduct an unadjusted and adjusted analysis by trial arm, to examine its impact on the results.
Selection of controls
Another important consideration was the method used to randomly select controls from each risk set for each case. This can be performed with or without replacement and including or excluding the case in the risk set. We chose the recommended option of sampling without replacement and excluding the case from the risk set, which produces the optimal unbiased estimate of relative risk, with greater statistical efficiency [ 17 , 18 ]. However, infants could be included in multiple risk sets and be selected more than once as a control. We also included future cases of NEC as controls in earlier risk sets, as their exclusion can also lead to biased estimates of relative risk [ 19 ].
Number of controls
In standard case-control studies it has been shown that there is little statistical efficiency gained from having more than four matched controls relative to each case [ 20 , 21 ]. Using five controls is only 4% more efficient than using four, therefore there is no added benefit in using additional controls if a cost is attached, for example taking extra biological samples in a prospective cohort setting. However gains in statistical efficiency are possible by using more than four controls if the probability of exposure among controls is low (< 0.1) [ 4 , 5 ]. Neither of these were issues for this particular analysis, as there were no additional costs involved in using more controls and prevalence of the defined exposures to non-breast milk was over 20% among infants without a diagnosis of NEC. However, there was a concern that including additional controls with increasing distance from the gestational age and birth weight of the case may undermine the matching algorithm. Also, increasing the number of controls sampled per case would lead to an increase in repeated sampling, resulting in larger number of duplicates present in the overall matched control population. This was a particular concern as control duplication was most likely to occur for infants with the lowest birth weight and gestational ages, from which there is a much smaller pool of control infants to sample from. This would have resulted in a small number of infants (with low birth weight and gestational age) being sampled multiple times and having disproportionate weighting in the matched control sample. Therefore, we limited the number of matched controls to four per case.
Statistical analysis
The baseline characteristics of infants with NEC, the matched control group, and all infants with no diagnosis of NEC (non-cases) were compared. Numbers (with percentages) were presented for binary and categorical variables, and means (and standard deviations) or medians (with interquartile range and/or range) for continuous variables. Cases were matched to four controls with the same sex and smallest Mahalanobis distance based on gestational age and birth weight. Conditional logistic regression was used to calculate the odds ratio of developing NEC for cases compared matched controls for each predefined exposure with 95% confidence intervals. Unadjusted odds ratios were calculated, along with estimates adjusting for ADEPT allocation.
The results of the full analysis, including the application of this method to explore the relationship between feed type and other clinically relevant outcomes, are reported in a separate clinical paper (in preparation). Of the 404 infants randomised to ADEPT, 398 were included in this analysis (1 infant was randomised in error, 1 set of parents withdrew consent, 3 infants had no daily feed log data and for 1 infant the severity of NEC was unknown). There were 35 cases of severe NEC and 363 infants without a diagnosis of severe NEC (non-cases). Of the 140 matched controls randomly sampled from the risk set, 109 were unique, 31 were sampled more than once, and 8 had a subsequent diagnosis of severe NEC.
The baseline characteristics of infants with severe NEC (cases) and their matched controls are shown in Table 1 , alongside the characteristics of infants without a diagnosis of severe NEC (non-cases). The matching algorithm successfully produced a well matched collection of controls, based on the majority of these characteristics. There were, however, a slightly higher proportion of infants with the lowest birthweights (< 750 g) among the cases compared to the matched controls (49% vs 38%). The only other factors to show a noticeable difference between cases and matched controls are maternal hypertension (37% vs 49%) and ventilation at trial entry (6% vs 21%), neither of which have been previously identified as risk factors for NEC. Figure 3 shows scatter plots of birth weight and gestational age for the 35 individual cases of NEC and their matched controls, which provides a visual representation of the matching.
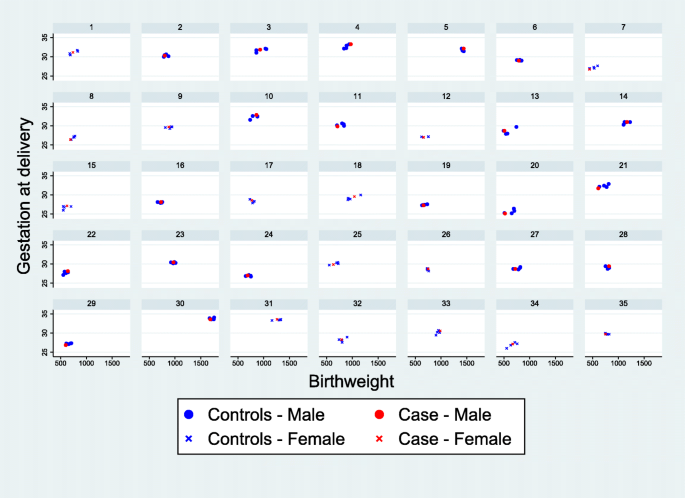
Scatterplots showing the matched cases and controls for each case of severe NEC. Each panel contains a separate case of NEC and the matched controls
The main results of the adjusted analysis are presented in Fig. 4 . Unadjusted analyses are included in Table A 1 in the supplementary material, alongside a post-hoc sensitivity analysis that additionally includes covariate adjustment for gestational age and birthweight. While the study did not identify any significant trends between feed-type and severe NEC the findings were consistent with the a priori hypothesis, that exposure to non-breast milk feeds is associated with an increased risk of NEC. In addition, the study identified some potential trends in the association of feed-type with other important outcomes, worthy of further investigation.
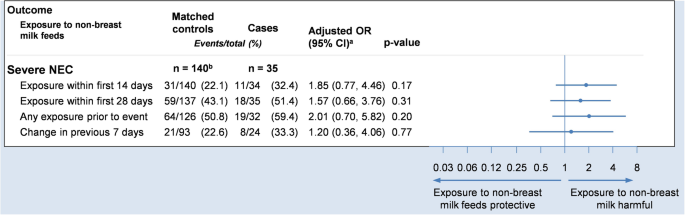
Forest plot showing the adjusted odds ratio comparing severe NEC to exposures. Odds ratios are adjusted for sex, gestational age and birthweight (via matching) and trial arm (via covariate adjustment). a Odds ratio and 95% confidence interval. b 109 unique controls
Employing a matched nested case-control design for this secondary analysis of clinical trial data overcame many of the limitations of a standard case-control analysis. We were able to select controls from the same population as the cases thus avoiding selection bias. Using matching, we were able to create a comparable sample of controls with respect to important clinical characteristics and confounding factors. This method allowed us to reliably investigate the temporal relationship between feed type and severe NEC since the exposure data was collected prospectively prior to the outcome occurring. We were also able to successfully investigate the relationship between feed type and several other important outcomes such as sepsis. A standard case-control analysis is typically based on recall or retrospective data collection once the outcome is known, which can introduce recall bias. If we had performed a simple comparison between cases and non-cases of NEC without taking into account the timing of the exposure, this would have produced misleading results. Another advantage of the matched nested case-control design was that we were able to match cases to controls at the time of the outcome event so that they were of comparable ages. The methodology is especially powerful when the timing of the exposure is of importance, particularly for time-dependent exposures such as the one studied here.
While the efficient use of existing trial data has a number of benefits, there are of course disadvantages to using data that were collected for another primary purpose. For instance, it is possible that such data are less robustly collected and checked. As a result, researchers may be more likely to encounter participants with either invalid or missing data.
For instance, the some of the additional feed log data collected in ADEPT were never intended to be used to answer clinical research questions, rather, their purpose was to monitor the adherence of participants to the intervention or provide added background information. In this study, it was necessary to make assumptions about missing data to fill small gaps in the daily feed logs. Researchers should take care that such assumptions are fully documented in the statistical analysis plan in advance and determined blinded to the outcome. Another option is to plan these sub-studies at the design phase, however, there needs to be a balance between the potential burden of additional data collection and having a streamlined trial that is able to answer the primary research question.
Another limitation of the methodology is that it is only possible to match on known confounders. This is in contrast to a randomised controlled trial, in which it is possible to balance on unknown and unmeasured baseline characteristics. As a consequence, particular care must be given to select important matching factors, but also to avoid overmatching.
The methodology allows for participants to be selected as controls multiple times, so there is the possibility that systematic duplication of a specific subset of participants (e.g. infants with a lower birthweight and smaller gestational age) could lead to a small number of participants disproportionately influencing the results. Within this study, we conducted sensitivity analyses with fewer controls, and were able to demonstrate that this had a minimal impact on the findings.
We have demonstrated how a matched nested case-control design can be embedded within an RCT to identify credible associations in a secondary analysis of clinical trial data where the exposure of interest was not randomised. We planned this study after the clinical trial data had already been collected, but it could have been built in seamlessly as a SWAT (Study Within A Trial) during the trial design phase, to ensure that all relevant data were collected in advance with minimal effort. This method has several advantages over a standard case-control design and offers the potential to make reliable inferences in scenarios where unethical or practical issues preclude the use of an RCT. Moreover, because of the flexibility of the methodology in terms of the design and analysis, the matched nested case-control design could reasonably be applied to a wide range of challenging research questions. There is an abundance of high quality large prospective studies and clinical trials with well characterised cohorts, in which this methodology could be applied to investigate causal relationships, adding considerable value for money to the original studies.
Availability of data and materials
ADEPT trial data are available upon reasonable request, subject to the NPEU Data Sharing Policy.
Abbreviations
Abnormal Doppler Enteral Prescription Trial
- Randomised controlled trial
Necrotising enterocolitis
Continuous positive airway pressure
Umbilical artery catheter
Umbilical venous catheter
Study within a trial
Breslow N. Design and analysis of case-control studies. Annu Rev Public Health. 1982;3(1):29–54.
Article CAS Google Scholar
Mantel N. Synthetic retrospective studies and related topics. Biometrics. 1973;29(3):479–86.
Breslow NE. Statistics in epidemiology: the case-control study. J Am Stat Assoc. 1996;91(433):14–28.
Breslow NE, Lubin J, Marek P, Langholz B. Multiplicative models and cohort analysis. J Am Stat Assoc. 1983;78(381):1–12.
Article Google Scholar
Goldstein L, Langholz B. Asymptotic theory for nested case-control sampling in the cox regression model. Ann Stat. 1992;20(4):1903–28.
Ernster VL. Nested Case-Control Studies. Prev Med. 1994;23(5):587–90. https://doi.org/10.1006/pmed.1994.1093 .
Article CAS PubMed Google Scholar
Essebag V, Genest J Jr, Suissa S, Pilote L. The nested case-control study in cardiology. Am Heart J. 2003;146(4):581–90. https://doi.org/10.1016/S0002-8703(03)00512-X .
Article PubMed Google Scholar
Nieuwlaat R, Connolly BJ, Hubers LM, Cuddy SM, Eikelboom JW, Yusuf S, et al. Quality of individual INR control and the risk of stroke and bleeding events in atrial fibrillation patients: a nested case control analysis of the ACTIVE W study. Thromb Res. 2012;129(6):715–9.
Fox GJ, Nhung NV, Loi NT, Sy DN, Britton WJ, Marks GB. Barriers to adherence with tuberculosis contact investigation in six provinces of Vietnam: a nested case–control study. BMC Infect Dis. 2015;15(1):103.
Mattson CL, Bailey RC, Agot K, Ndinya-Achola J, Moses S. A nested case-control study of sexual practices and risk factors for prevalent HIV-1 infection among young men in Kisumu, Kenya. Sex Trans Dis. 2007;34(10):731.
Google Scholar
Leaf A, Dorling J, Kempley S, McCormick K, Mannix P, Linsell L, et al. Early or delayed enteral feeding for preterm growth-restricted infants: a randomized trial. Pediatrics. 2012;129(5):e1260–e8. https://doi.org/10.1542/peds.2011-2379 .
Lucas A, Cole TJ. Breast milk and neonatal necrotising enterocolitis. Lancet. 1990;336(8730):1519–23.
McGuire W, Anthony MY. Donor human milk versus formula for preventing necrotising enterocolitis in preterm infants: systematic review. Arch Dis Child Fetal Neonatal Ed. 2003;88(1):F11–F4.
Walsh MC, Kliegman RM. Necrotizing enterocolitis: treatment based on staging criteria. Pediatr Clin N Am. 1986;33:179–201.
Mahalanobis PC. On the Generalized Distance in Statistics. Proceedings of the National Institute of Science of India. 1936;2:49-55.
Brookmeyer R, Liang K, Linet M. Matched case-control designs and overmatched analyses. Am J Epidemiol. 1986;124(4):693–701.
Lubin JH. Extensions of analytic methods for nested and population-based incident case-control studies. J Chronic Dis. 1986;39(5):379–88.
Robins JM, Gail MH, Lubin JH. More on" biased selection of controls for case-control analyses of cohort studies". Biometrics. 1986;42(2):293–9.
Lubin JH, Gail MH. Biased selection of controls for case-control analyses of cohort studies. Biometrics. 1984;40(1):63–75.
Ury HK. Efficiency of case-control studies with multiple controls per case: continuous or dichotomous data. Biometrics. 1975;31(3):643–9.
Gail M, Williams R, Byar DP, Brown C. How many controls? J Chronic Dis. 1976;29(11):723–31.
Meeting abstracts from the 5th International Clinical Trials Methodology Conference (ICTMC 2019). Trials. 2019;20(Suppl 1):579 Brighton, UK. 06–09 October 2019. doi: 10e.1186/s13063-019-3688-6 .
Download references
Acknowledgements
This work was presented at the International Clinical Trials Methodology Conference (ICTMC) in 2019 and the abstract is published within Trials [ 22 ].
This work was supported by Action Medical Research [Grant number GN2506]. The funder had no role in study design, data collection and analysis, decision to publish, or preparation of the manuscript.
Author information
Authors and affiliations.
Nottingham Clinical Trials Unit, University of Nottingham, Nottingham, UK
Christopher Partlett
National Perinatal Epidemiology Unit, Nuffield Department of Population Health, University of Oxford, Oxford, UK
Christopher Partlett, Alison Leaf, Edmund Juszczak & Louise Linsell
University Surgery Unit, Faculty of Medicine, University of Southampton, Southampton, UK
Nigel J. Hall
Department of Child Health, Faculty of Medicine, University of Southampton, Southampton, UK
Alison Leaf
You can also search for this author in PubMed Google Scholar
Contributions
NH, AL, EJ and LL conceived the project. CP performed the statistical analyses under the supervision of LL and EJ. CP and LL drafted the manuscript and EJ, AL and NH critically reviewed it. All authors were involved in the interpretation of results. The author(s) read and approved the final manuscript.
Corresponding author
Correspondence to Christopher Partlett .
Ethics declarations
Ethics approval and consent to participate.
No ethical approval was required for this study, since it used only previously collected, fully anonymised research data.
Consent for publication
Not applicable.
Competing interests
The authors declare that they have no competing interest.
Additional information
Publisher’s note.
Springer Nature remains neutral with regard to jurisdictional claims in published maps and institutional affiliations.
Supplementary information
Additional file 1..
Table A1 Association between exposures and the development of Severe NEC. Each case is matched to 4 controls with the same sex and the smallest distance in terms of the Malhalanobis distance based on gestational age and birthweight.
Rights and permissions
Open Access This article is licensed under a Creative Commons Attribution 4.0 International License, which permits use, sharing, adaptation, distribution and reproduction in any medium or format, as long as you give appropriate credit to the original author(s) and the source, provide a link to the Creative Commons licence, and indicate if changes were made. The images or other third party material in this article are included in the article's Creative Commons licence, unless indicated otherwise in a credit line to the material. If material is not included in the article's Creative Commons licence and your intended use is not permitted by statutory regulation or exceeds the permitted use, you will need to obtain permission directly from the copyright holder. To view a copy of this licence, visit http://creativecommons.org/licenses/by/4.0/ . The Creative Commons Public Domain Dedication waiver ( http://creativecommons.org/publicdomain/zero/1.0/ ) applies to the data made available in this article, unless otherwise stated in a credit line to the data.
Reprints and permissions
About this article
Cite this article.
Partlett, C., Hall, N.J., Leaf, A. et al. Application of the matched nested case-control design to the secondary analysis of trial data. BMC Med Res Methodol 20 , 117 (2020). https://doi.org/10.1186/s12874-020-01007-w
Download citation
Received : 03 December 2019
Accepted : 05 May 2020
Published : 14 May 2020
DOI : https://doi.org/10.1186/s12874-020-01007-w
Share this article
Anyone you share the following link with will be able to read this content:
Sorry, a shareable link is not currently available for this article.
Provided by the Springer Nature SharedIt content-sharing initiative
- Preterm infants
- Neonatology
- Statistical methods
- Nested case-control
BMC Medical Research Methodology
ISSN: 1471-2288
- General enquiries: [email protected]
- - Google Chrome
Intended for healthcare professionals
- Access provided by Google Indexer
- My email alerts
- BMA member login
- Username * Password * Forgot your log in details? Need to activate BMA Member Log In Log in via OpenAthens Log in via your institution

Search form
- Advanced search
- Search responses
- Search blogs
- Analysis of matched...
Analysis of matched case-control studies
- Related content
- Peer review
- Neil Pearce , professor 1 2
- 1 Department of Medical Statistics and Centre for Global NCDs, Faculty of Epidemiology and Population Health, London School of Hygiene and Tropical Medicine, London WC1E 7HT, UK
- 2 Centre for Public Health Research, Massey University, Wellington, New Zealand
- neil.pearce{at}lshtm.ac.uk
- Accepted 30 December 2015
There are two common misconceptions about case-control studies: that matching in itself eliminates (controls) confounding by the matching factors, and that if matching has been performed, then a “matched analysis” is required. However, matching in a case-control study does not control for confounding by the matching factors; in fact it can introduce confounding by the matching factors even when it did not exist in the source population. Thus, a matched design may require controlling for the matching factors in the analysis. However, it is not the case that a matched design requires a matched analysis. Provided that there are no problems of sparse data, control for the matching factors can be obtained, with no loss of validity and a possible increase in precision, using a “standard” (unconditional) analysis, and a “matched” (conditional) analysis may not be required or appropriate.
Summary points
Matching in a case-control study does not control for confounding by the matching factors
A matched design may require controlling for the matching factors in the analysis
However, it is not the case that a matched design requires a matched analysis
A “standard” (unconditional) analysis may be most valid and appropriate, and a “matched” (conditional) analysis may not be required or appropriate
Matching on factors such as age and sex is commonly used in case-control studies. 1 This can be done for convenience (eg, choosing a control admitted to hospital on the same day as the case), to improve study efficiency by improving precision (under certain conditions) when controlling for the matching factors (eg, age, sex) in the analysis, or to enable control in the analysis of unquantifiable factors such as neighbourhood characteristics (eg, by choosing neighbours as controls and then controlling for neighbourhood in the analysis). The increase in efficiency occurs because it ensures similar numbers of cases and controls in confounder strata. For example, in a study of lung cancer, if controls are sampled at random from the source population, their age distribution will be much younger than that of the lung cancer cases. Thus, when age is controlled in the analysis, the young age stratum may contain mostly controls and few cases, whereas the old age stratum may contain mostly cases and fewer controls. Thus, statistical precision may be improved if controls are age matched to ensure roughly equal numbers of cases and controls in each age stratum.
There are two common misconceptions about case-control studies: that matching in itself eliminates confounding by the matching factors; and that if matching has been performed, then a “matched analysis” is required.
Matching in the design does not control for confounding by the matching factors. In fact, it can introduce confounding by the matching factors even when it did not exist in the source population. 1 The reasons for this are complex and will only be discussed briefly here. In essence, the matching process makes the controls more similar to the cases not only for the matching factor but also for the exposure itself. This introduces a bias that needs to be controlled in the analysis. For example, suppose we were conducting a case-control study of poverty and death (from any cause), and we chose siblings as controls (that is, for each person who died, we matched on family or residence by choosing a sibling who was still alive as a control). In this situation, since poverty runs in families we would tend to select a disadvantaged control for each disadvantaged person who had died and a wealthy control for each wealthy person who had died. We would find roughly equal percentages of disadvantaged people among the cases and controls, and we would find little association between poverty and mortality. The matching has introduced a bias, which fortunately (as we will illustrate) can be controlled by controlling for the matching factor in the analysis.
Thus, a matched design will (almost always) require controlling for the matching factors in the analysis. However, this does not necessarily mean that a matched analysis is required or appropriate, and it will often be sufficient to control for the matching factors using simpler methods. Although this is well recognised in both recent 2 3 and historical 4 5 texts, other texts 6 7 8 9 do not discuss this issue and present the matched analysis as the only option for analysing matched case-control studies. In fact, the more standard analysis may not only be valid but may be much easier in practice, and yield better statistical precision.
In this paper I explore and illustrate these problems using a hypothetical pair matched case-control study.
Options for analysing case-control studies
Unmatched case-control studies are typically analysed using the Mantel-Haenszel method 10 or unconditional logistic regression. 4 The former involves the familiar method of producing a 2×2 (exposure-disease) stratum for each level of the confounder (eg, if there are five age groups and two sex groups, then there will be 10 2×2 tables, each showing the association between exposure and disease within a particular stratum), and then producing a summary (average) effect across the strata. The Mantel-Haenszel estimates are robust and not affected by small numbers in specific strata (provided that the overall numbers of exposed or non-exposed cases or controls are adequate), although it can be difficult or impossible to control for factors other than the matching factors if some strata involve small numbers (eg, just one case and one control). Furthermore, the Mantel-Haenszel approach works well when there are only a few confounder strata, but will experience problems of small numbers (eg, strata with only cases and no controls) if there are too many confounders to adjust for. In this situation, logistic regression may be preferred, since this uses maximum likelihood methods, which enable the adjustment (given certain assumptions) of more confounders.
Suppose that for each case we have chosen a control who is in the same five year age group (eg, if the case is aged 47 years, then a control is chosen who is aged 45-49 years). We can then perform a standard analysis, which adjusts for the matching factor (age group) by grouping all cases and controls into five year age groups and using unconditional logistic regression 4 (or the Mantel-Haenszel method 10 ); if there are eight age groups then this analysis will just have eight strata (represented by seven age group dummy variables), each with multiple cases and controls. Alternatively we can perform a matched analysis (that is, retaining the pair matching of one control for each case) using conditional logistic regression (or the matched data methods, which are equivalent to the Mantel-Haenszel method); if there are 100 case-control pairs, this analysis will then have 100 strata.
The main reason for using conditional (rather than unconditional) logistic regression is that when the analysis strata are very small (eg, with just one case and one control for each stratum), problems of sparse data will occur with unconditional methods. 11 For example, if there are 100 strata, this requires 99 dummy variables to represent them, even though there are only 200 study participants. In this extreme situation, unconditional logistic regression is biased and produces an odds ratio estimate that is the square of the conditional (true) estimate of the odds ratio. 5 12
Example of age matching
Table 1 ⇓ gives an example of age matching in a population based case-control study, and shows the “true’ findings for the total population, the findings for the corresponding unmatched case-control study, and the findings for an age matched case-control study using the standard analysis. Table 2 ⇓ presents the findings for the same age matched case-control study using the matched analysis. All analyses were performed using the Mantel-Haenszel method, but this yields similar results to the corresponding (unconditional or conditional) logistic regression analyses.
Hypothetical study population and case-control study with unmatched and matched standard analyses
- View inline
Hypothetical matched case-control study with matched analysis
Table 1 ⇑ shows that the crude odds ratio in the total population is 0.86 (0.70 to 1.05), but this changes to 2.00 (1.59 to 2.51) when the analysis is adjusted for age (using the Mantel-Haenszel method). This occurs because there is strong confounding by age—the cases are mostly old, and old people have a lower exposure than young people. Overall, there are 390 cases, and when 390 controls are selected at random from the non-cases in the total population (which is half exposed and half not exposed), this yields the same crude (0.86) and adjusted (2.00) odds ratios, but with wider confidence intervals, reflecting the smaller numbers of non-cases (controls) in the case-control study.
Why matching factors need to be controlled in the analysis
Now suppose that we reconduct the case-control study, matching for age, using two very broad age groups: old and young (table 1 ⇑ ). The number of cases and controls in each age group are now equal. However, the crude odds ratio (1.68, 1.25 to 2.24) is different from both the crude (0.86) and the adjusted (2.00) odds ratios in the total population. In contrast, the adjusted odds ratio (2.00) is the same as that in the total population and in the unmatched case-control study (both of these adjusted odds ratios were estimated using the standard approach). Thus, matching has not removed age confounding and it is still necessary to control for age (this occurs because the matching process in a case-control study changes the association between the matching factor and the outcome and can create an association even if there were none before the matching was conducted). However, there is a small increase in precision in the matched case-control study compared with the unmatched case-control studies (95% confidence intervals of 1.42 to 2.81 compared with 1.38 to 2.89) because there are now equal numbers of cases and controls in each age group (table 1 ⇑ ).
A pair matched study does not necessarily require a pair matched analysis
However, control for simple matching factors such as age does not require a pair matched analysis. Table 2 ⇑ gives the findings that would have been obtained from a pair matched analysis (this is created by assuming that in each age group, and for each case, the control was selected at random from all non-cases in the same age group). The standard adjusted (Mantel-Haenszel) analysis (table 1 ⇑ ) yields an odds ratio of 2.00 (95% confidence interval 1.42 to 2.81); the matched analysis (table 2 ⇑ ) yields the same odds ratio (2.00) but with a slightly wider confidence interval (1.40 to 2.89).
Advantages of the standard analysis
So for many matched case-control studies, we have a choice of doing a standard analysis or a matched analysis. In this situation, there are several possible advantages of using the standard approach.
The standard analysis can actually yield slightly better statistical precision. 13 This may apply, for example, if two or more cases and their matched controls all have identical values for their matching factors; then combining them into a single stratum produces an estimator with lower variance and no less validity 14 (as indicated by the slightly narrower confidence interval for the standard adjusted analysis (table 1 ⇑ ) compared with the pair matched analysis (table 2 ⇑ ). This particularly occurs because combining strata with identical values for the matching factors (eg, if two case-control pairs all concern women aged 55-59 years) may mean that fewer data are discarded (that is, do not contribute to the analysis) because of strata where the case and control have the same exposure status. Further gains in precision may be obtained if combining strata means that cases with no corresponding control (or controls without a corresponding case) can be included in the analysis. When such strata are combined, a conditional analysis may still be required if the resulting strata are still “small,” 13 but an unconditional analysis will be valid and yield similar findings if the resulting strata are sufficiently large. This may often be the case when matching has only been performed on standard factors such as sex and age group.
The standard analysis may also enhance the clarity of the presentation, particularly when analysing subgroups of cases and controls selected for variables on which they were not matched, since it involves standard 2×2 tables for each subgroup. 15
A further advantage of the standard analysis is that it makes it easier to combine different datasets that have involved matching on different factors (eg, if some have matched for age, some for age and sex, and some for nothing, then all can be combined in an analysis adjusting for age, sex, and study centre). In contrast, one multicentre study 16 (of which I happened to be a coauthor) attempted to (unnecessarily) perform a matched analysis across centres. Because not all centres had used pair matching, this involved retrospective pair matching in those centres that had not matched as part of the study design. This resulted in the unnecessary discarding of the unmatched controls, thus resulting in a likely loss of precision.
Conclusions
If matching is carried out on a particular factor such as age in a case-control study, then controlling for it in the analysis must be considered. This control should involve just as much precision as was used in the original matching 14 (eg, if exact age in years was used in the matching, then exact age in years should be controlled for in the analysis), although in practice such rigorous precision may not always be required (eg, five year age groups may suffice to control confounding by age, even if age matching was done more precisely than this). In some circumstances, this control may make no difference to the main exposure effect estimate—eg, if the matching factor is unrelated to exposure. However, if there is an association between the matching factor and the exposure, then matching will introduce confounding that needs to be controlled for in the analysis.
So when is a pair matched analysis required? The answer is, when the matching was genuinely at (or close to) the individual level. For example, if siblings have been chosen as controls, then each stratum would have just one case and the sibling control; in this situation, an unconditional logistic regression analysis would suffer from problems of sparse data, and conditional logistic regression would be required. Similar situations might arise if controls were neighbours or from the same general practice (if each general practice only had one or a few cases), or if matching was performed on many factors simultaneously so that most strata (in the standard analysis) had just one case and one control.
Provided, however, that there are no problems of sparse data, such control for the matching factors can be obtained using an unconditional analysis, with no loss of validity and a possible increase in precision.
Thus, a matched design will (nearly always) require controlling for the matching factors in the analysis. It is not the case, however, that a matched design requires a matched analysis.
I thank Simon Cousens, Deborah Lawlor, Lorenzo Richiardi, and Jan Vandenbroucke for their comments on the draft manuscript. The Centre for Global NCDs is supported by the Wellcome Trust Institutional Strategic Support Fund, 097834/Z/11/B.
Competing interests: I have read and understood the BMJ policy on declaration of interests and declare the following: none.
Provenance and peer review: Not commissioned; externally peer reviewed.
This is an Open Access article distributed in accordance with the Creative Commons Attribution Non Commercial (CC BY-NC 3.0) license, which permits others to distribute, remix, adapt, build upon this work non-commercially, and license their derivative works on different terms, provided the original work is properly cited and the use is non-commercial. See: http://creativecommons.org/licenses/by-nc/3.0/ .
- ↵ Rothman KJ, Greenland S, Lash TL, eds Design strategies to improve study accuracy. Modern epidemiology. 3rd ed . Lippincott Williams & Wilkins, 2008 .
- ↵ Rothman KJ. Epidemiology: an introduction. Oxford University Press, 2012 .
- ↵ Rothman KJ, Greenland S, Lash TL, eds. Modern epidemiology. 3rd ed . Lippincott Williams & Wilkins, 2008 .
- ↵ Breslow NE, Day NE. Statistical methods in cancer research. Vol I: the analysis of case-control studies. IARC, 1980 .
- ↵ Kleinbaum DG, Kupper LL, Morgenstern H. Epidemiologic research: principles and quantitative methods. Lifetime Learning Publications, 1982 .
- ↵ Dos Santos Silva I. Cancer epidemiology: principles and methods. IARC, 1999 .
- ↵ Keogh RH, Cox DR. Case-control studies. Cambridge University Press, 2014 doi:10.1017/CBO9781139094757 . .
- ↵ Lilienfeld DE, Stolley PD. Foundations of epidemiology. 3rd ed . Oxford University Press, 1994 .
- ↵ MacMahon B, Trichopolous D. Epidemiology: principles and methods. 2nd ed . Little Brown, 1996 .
- ↵ Mantel N, Haenszel W. Statistical aspects of the analysis of data from retrospective studies of disease. J Natl Cancer Inst 1959 ; 22 :719- 48 . 13655060 .
- ↵ Robins J, Greenland S, Breslow NE. A general estimator for the variance of the Mantel-Haenszel odds ratio. Am J Epidemiol 1986 ; 124 :719- 23 . 3766505 .
- ↵ Pike MC, Hill AP, Smith PG. Bias and efficiency in logistic analyses of stratified case-control studies. Int J Epidemiol 1980 ; 9 :89- 95 . doi:10.1093/ije/9.1.89 . 7419334 .
- ↵ Brookmeyer R, Liang KY, Linet M. Matched case-control designs and overmatched analyses. Am J Epidemiol 1986 ; 124 :693- 701 . 3752063 .
- ↵ Greenland S. Applications of stratified analysis methods. In: Rothman KJ, Greenland S, Lash TL, eds. Modern epidemiology. 3rd ed . Lippincott Williams & Wilkins, 2008 .
- ↵ Vandenbroucke JP, Koster T, Briët E, Reitsma PH, Bertina RM, Rosendaal FR. Increased risk of venous thrombosis in oral-contraceptive users who are carriers of factor V Leiden mutation. Lancet 1994 ; 344 :1453- 7 . doi:10.1016/S0140-6736(94)90286-0 . 7968118 .
- ↵ Cardis E, Richardson L, Deltour I, et al. The INTERPHONE study: design, epidemiological methods, and description of the study population. Eur J Epidemiol 2007 ; 22 :647- 64 . doi:10.1007/s10654-007-9152-z . 17636416 .
- Mansournia MA, Hernán MA, Greenland S. Matched designs and causal diagrams. Int J Epidemiol 2013 ; 42 :860- 9 . doi:10.1093/ije/dyt083 . 23918854 .
- Hernán MA, Hernández-Díaz S, Robins JM. A structural approach to selection bias. Epidemiology 2004 ; 15 :615- 25 . doi:10.1097/01.ede.0000135174.63482.43 . 15308962 .
- Journal home
- Advance online publication
- About the journal
- J-STAGE home
- Annals of Clinical Epidemiolog ...
- Volume 4 (2022) Issue 2
- Article overview
Department of Health Services Research, Faculty of Medicine, University of Tsukuba Faculty of Epidemiology and Population Health, London School of Hygiene and Tropical Medicine
Tokyo University of Science, Department of Information and Computer Technology
Corresponding author

2022 Volume 4 Issue 2 Pages 33-40
- Published: 2022 Received: - Available on J-STAGE: April 04, 2022 Accepted: - Advance online publication: - Revised: -
(compatible with EndNote, Reference Manager, ProCite, RefWorks)
(compatible with BibDesk, LaTeX)
Matching is a technique through which patients with and without an outcome of interest (in case-control studies) or patients with and without an exposure of interest (in cohort studies) are sampled from an underlying cohort to have the same or similar distributions of some characteristics. This technique is used to increase the statistical efficiency and cost efficiency of studies. In case-control studies, besides time in risk set sampling, controls are often matched for each case with respect to important confounding factors, such as age and sex, and covariates with a large number of values or levels, such as area of residence (e.g., post code) and clinics/hospitals. In the statistical analysis of matched case-control studies, fixed-effect models such as the Mantel-Haenszel odds ratio estimator and conditional logistic regression model are needed to stratify matched case-control sets and remove selection bias artificially introduced by sampling controls. In cohort studies, exact matching is used to increase study efficiency and remove or reduce confounding effects of matching factors. Propensity score matching is another matching method whereby patients with and without exposure are matched based on estimated propensity scores to receive exposure. If appropriately used, matching can improve study efficiency without introducing bias and could also present results that are more intuitive for clinicians.
Matching is mainly used in observational studies, including case-control and cohort studies. Matching is a technique by which patients with and without an outcome of interest (in case-control studies) or patients with and without an exposure of interest (in cohort studies) are sampled from an underlying cohort to have the same or similar distributions of characteristics such as age and sex.
The main purpose of matching is to increase study efficiency for data collection and subsequent statistical analysis. Matching helps researchers reduce the volume of data for collection without much loss of information (i.e., improving cost efficiency) and obtain more precise estimates than simple random sampling of the same number of patients (i.e., improving statistical efficiency). In addition, in cohort studies, matching can remove or reduce confounding effects of matching factors.
This paper aims to introduce basic principles of matching in case-control and cohort studies, with some recent examples.
Fig. 1 Graphical representation of cumulative incidence sampling (A), case-control sampling (B), and risk set sampling (C) for 10 example patients in a cohort. ● indicates an outcome onset and time at selection as a case. ○ indicates time at selection as a control.
1. Wacholder S. The case-control study as data missing by design: estimating risk differences. Epidemiology 1996;7:144–150.
2. Noma H, Tanaka S. Analysis of case-cohort designs with binary outcomes: improving efficiency using whole-cohort auxiliary information. Stat Methods Med Res 2017;26:691–706.

Fig. 2 Graphical representation of a risk set sampling for 10 example patients in a population-based cohort. ● indicates an outcome onset and time at selection as a case. ○ indicates time at selection as a control.

In a study requiring primary data collection, case-control study designs are efficient because only information on cases and selected controls, instead of all people in the underlying cohort, is collected and used for statistical analysis. Especially for rare outcomes, a cohort study recruiting many people to observe a sufficient number of outcomes is not feasible. However, a case-control design would still be feasible, with reduced costs and efforts.
3. Schuemie MJ, Ryan PB, Man KKC, Wong ICK, Suchard MA, Hripcsak G. A plea to stop using the case-control design in retrospective database studies. Stat Med 2019;38:4199–4208.
4. Schneeweiss S, Suissa S.Discussion of Schuemie et al. “A plea to stop using the case-control design in retrospective database studies”. Stat Med 2019;38:4209–4212.
Similar to cohort studies, case-control studies typically require confounder adjustment using stratified analysis or regression modeling. To further improve statistical efficiency in adjusted analyses, case-control studies may match controls on confounders to be adjusted for, i.e., sampling a control(s) with an identical (or nearly identical) value of confounders for each case. When the total number of cases and controls to be sampled is fixed, the adjusted odds ratio estimates are likely to be less variable (i.e., more statistically efficient) in case-control data matched on strong confounders than in unmatched data.
Besides common confounding factors such as age and sex, area of residence (e.g., post code) or clinics/hospitals (which patients are registered to or visit) are sometimes matched between cases and controls. If variables with a large number of values or levels (e.g., over 1,000 post codes or clinics/hospitals) are adjusted for as “surrogate” confounders in the statistical analysis, at least one case and one control in each area (or clinic/hospital) are needed; otherwise, the data are discarded in the fixed-effect models (stratification). Although a case and control may rarely come from the same area (or clinic/hospital) in unmatched case-control sampling, matching can ensure that the pairs (or sets) of cases and controls are derived from the same area (or clinics/hospitals). Consequently, the odds ratio adjusted for these variables can be efficiently estimated.
5. Rothman KJ, Lash TL. 6 Epidemiologic study design with validity and efficiency considerations. Modern epidemiology 4th edition. Lippincott Williams & Wilkins, 2021:105–140.
6. Marsh JL, Hutton JL, Binks K. Removal of radiation dose response effects: an example of over-matching. BMJ 2002;325:327–330.
7. Richardson K, Fox C, Maidment I, Steel N, Loke YK, Arthur A, et al. Anticholinergic drugs and risk of dementia: case-control study. BMJ 2018;361:k1315.
8. Lapi F, Azoulay L, Yin H, Nessim SJ, Suissa S. Concurrent use of diuretics, angiotensin converting enzyme inhibitors, and angiotensin receptor blockers with non-steroidal anti-inflammatory drugs and risk of acute kidney injury: nested case-control study. BMJ 2013;346:e8525.
9. Woodward M. Epidemiology: study design and data analysis. Chapman & Hall: Boca Raton, 1999:265.
10. Hennessy S, Bilker WB, Berlin JA, Strom BL. Factors influencing the optimal control-to-case ratio in matched case-control studies. Am J Epidemiol 1999;149:195–197.
Sometimes, a case cannot find a prespecified number of controls. For example, in a case-control study planning 1:4 matching, some cases could find only less than four controls. However, it is not necessary to exclude these pairs when matching factors or matched sets of cases and controls are stratified in the analysis. The mixture of pairs with different matching ratios will not result in a biased estimate as long as an adequate adjustment for matching factors is adopted.
11. Wang MH, Shugart YY, Cole SR, Platz EA. A simulation study of control sampling methods for nested case-control studies of genetic and molecular biomarkers and prostate cancer progression. Cancer Epidemiol Biomarkers Prev 2009;18:706–711.
To remove the selection bias artificially introduced by case-control matching, it is necessary to “stratify” data on matching factors in the statistical analysis. One traditional method is the Mantel-Haenszel odds ratio estimator that stratifies on matching factors themselves (e.g., subgroups by age group and sex, if controls are matched on these factors) or matched sets (e.g., each pair of a case and control). The Mantel-Haenszel estimator adjusts for matching factors as fixed effects and estimates a common odds ratio assumed to be constant across strata. The Mantel-Haenszel odds ratio estimator consistently estimates the common odds ratio when each stratum contains sparse data (e.g., only two patients, one case and one control, in each stratum) but the number of strata increases. Adjusting for confounding factors besides the matching factors by additional stratification within the matching factor strata is infeasible.
12. Pearce N. Analysis of matched case-control studies. BMJ 2016;352:i969.
13. Greenland S. Partial and marginal matching in case-control studies. Modern statistical methods in chronic disease epidemiology. Wiley: New York, NY, 1986:35–49.
Finally, time at matching (time from cohort entry, calendar time, or possibly age as time from birth) can be considered one of the “matching factors” in risk set sampling. If the hazard of disease incidence varies with time and the exposure prevalence changes during follow-up, time should be accounted for as a “confounder.” To do so, one can use the Mantel-Haenszel odds ratio estimator or a conditional logistic regression model, which estimates the hazard ratio constant over time (and across other matching factors, if any) that would be modeled by the Cox proportional hazards model in an underlying cohort.
14. Hayashi M, Takamatsu I, Kanno Y, Yoshida T, Abe T, Sato Y, Japanese Calciphylaxis Study Group. A case-control study of calciphylaxis in Japanese end-stage renal disease patients. Nephrol Dial Transplant 2012;27:1580–1584.
15. Iwagami M, Taniguchi Y, Jin X, Adomi M, Mori T, Hamada S, et al. Association between recorded medical diagnoses and incidence of long-term care needs certification: a case control study using linked medical and long-term care data in two Japanese cities. Annals Clin Epidemiol 2019;1:56–68.
A matched cohort study may also be conducted from a practical viewpoint: it would provide an intuitive presentation of patient characteristics in “comparable” exposure groups matched on important confounding factors such as age, sex, and calendar time. As crude absolute measures (such as risks and rates) during the follow-up period are easily summarized in exposed and unexposed patients, clinicians unfamiliar with statistical analysis can grasp the difference between the two groups in a non-statistical manner.
Fig. 3 Graphical representation of a matched-pair cohort study for 10 example patients in a cohort. Solid lines indicate that people are exposed, dotted lines denote that people are not exposed, and ● indicates the incidence of outcome.

Fig. 4 Graphical representation of a matched-pair cohort study for 10 example patients in a population-based cohort. Solid lines denote that people are exposed, dotted lines denote that people are not exposed, ▼ indicates the timing of the matched-pair cohort inclusion in the exposed group, ▽ indicates the timing of the matched-pair cohort inclusion in the non-exposed group, and ● indicates the incidence of outcome.
16. Suissa S, Dell’Aniello S. Time-related biases in pharmacoepidemiology. Pharmacoepidemiol Drug Saf 2020;29:1101–1110.
17. Thomas LE, Yang S, Wojdyla D, Schaubel DE. Matching with time-dependent treatments: a review and look forward. Stat Med 2020;39:2350–2370.

18. Greenland S, Morgenstern H. Matching and efficiency in cohort studies. Am J Epidemiol 1990;131:151–159.
Regarding the matching ratio, 1:4 or 1:5 is sometimes chosen in matched-pair cohort studies, whereas 1:1 may be chosen more frequently to prioritize simplicity and intuitiveness. Mixed matching ratios (meaning that, for example, some pairs are matched in a ratio of 1:4, whereas other pairs are matched by a ratio of 1:3, 1:2, or 1:1 between exposed and unexposed people) will not cause bias if matching variables or matched sets are adjusted for in the analysis. In contrast, as such varying matching ratios do not balance the distributions of matching factors in exposed and unexposed people, the unadjusted comparison in the matched cohort still suffers from confounding bias.
Matching with or without replacement remains the choice of researchers, although matching without replacement may be more intuitive for clinicians.
19. Shinozaki T, Mansournia MA, Matsuyama Y. On hazard ratio estimators by proportional hazards models in matched-pair cohort studies. Emerg Themes Epidemiol 2017;14:6.
20. Sjölander A, Greenland S. Ignoring the matching variables in cohort studies – when is it valid and why? Stat Med 2013;32:4696–4708.
21. Sutradhar R, Baxter NN, Austin PC. Terminating observation within matched pairs of subjects in a matched cohort analysis: a Monte Carlo simulation study. Stat Med 2016;35:294–304.
22. Shinozaki T, Mansournia MA. Hazard ratio estimators after terminating observation within matched pairs in sibling and propensity score matched designs. Int J Biostat 2019;15.
23. Yasunaga H. Introduction to applied statistics—chapter 1 propensity score analysis. Annals Clin Epidemiol 2020;2:33–37.
24. Abadie A, Imbens GW. Matching on the estimated propensity score. Econometrica 2016;84:781–807.
25. Shinozaki T, Nojima M. Misuse of regression adjustment for additional confounders following insufficient propensity score balancing. Epidemiology 2019;30:541–548.
26. Ohbe H, Goto T, Miyamoto Y, Yasunaga H. Risk of cardiovascular events after spouse’s ICU admission. Circulation 2020;142:1691–1693.
27. Nagasu H, Yano Y, Kanegae H, Heerspink HJL, Nangaku M, Hirakawa Y, et al. Kidney outcomes associated with SGLT2 inhibitors versus other glucose-lowering drugs in real-world clinical practice: the Japan chronic kidney disease database. Diabetes Care 2021;44:2542–2551.
We have provided an overview and some recent examples of matching in case-control and cohort studies. Matching in case-control studies can increase study efficiency, including both cost and statistical efficiencies. Nevertheless, caution is still warranted since inappropriate sampling of controls and application of statistical analysis without stratification would result in a biased estimate. In cohort studies, exact matching can increase efficiency and remove or reduce the confounding effect of matching factors, whereas a propensity score matching can be used to balance the distributions of measured confounding factors between exposed and unexposed individuals. If appropriately used, matching can improve study efficiency without introducing bias and can present results that are more intuitive for clinicians.
We would like to thank Dr. Hiroyuki Ohbe of the Department of Clinical Epidemiology and Health Economics, School of Public Health, The University of Tokyo, and Dr. Motohiko Adomi in the Department of Epidemiology, Harvard T.H. Chan School of Public Health, for their critical reading of the manuscript and feedback.
No potential competing interests relevant to this paper are reported.
- 1. Wacholder S. The case-control study as data missing by design: estimating risk differences. Epidemiology 1996; 7 :144–150.
- 2. Noma H, Tanaka S. Analysis of case-cohort designs with binary outcomes: improving efficiency using whole-cohort auxiliary information. Stat Methods Med Res 2017; 26 :691–706.
- 3. Schuemie MJ, Ryan PB, Man KKC, Wong ICK, Suchard MA, Hripcsak G. A plea to stop using the case-control design in retrospective database studies. Stat Med 2019; 38 :4199–4208.
- 4. Schneeweiss S, Suissa S.Discussion of Schuemie et al. “A plea to stop using the case-control design in retrospective database studies”. Stat Med 2019; 38 :4209–4212.
- 5. Rothman KJ, Lash TL. 6 Epidemiologic study design with validity and efficiency considerations. Modern epidemiology 4th edition. Lippincott Williams & Wilkins, 2021:105–140.
- 6. Marsh JL, Hutton JL, Binks K. Removal of radiation dose response effects: an example of over-matching. BMJ 2002; 325 :327–330.
- 7. Richardson K, Fox C, Maidment I, Steel N, Loke YK, Arthur A, et al. Anticholinergic drugs and risk of dementia: case-control study. BMJ 2018; 361 :k1315.
- 8. Lapi F, Azoulay L, Yin H, Nessim SJ, Suissa S. Concurrent use of diuretics, angiotensin converting enzyme inhibitors, and angiotensin receptor blockers with non-steroidal anti-inflammatory drugs and risk of acute kidney injury: nested case-control study. BMJ 2013; 346 :e8525.
- 9. Woodward M. Epidemiology: study design and data analysis. Chapman & Hall: Boca Raton, 1999:265.
- 10. Hennessy S, Bilker WB, Berlin JA, Strom BL. Factors influencing the optimal control-to-case ratio in matched case-control studies. Am J Epidemiol 1999; 149 :195–197.
- 11. Wang MH, Shugart YY, Cole SR, Platz EA. A simulation study of control sampling methods for nested case-control studies of genetic and molecular biomarkers and prostate cancer progression. Cancer Epidemiol Biomarkers Prev 2009; 18 :706–711.
- 12. Pearce N. Analysis of matched case-control studies. BMJ 2016; 352 :i969.
- 13. Greenland S. Partial and marginal matching in case-control studies. Modern statistical methods in chronic disease epidemiology. Wiley: New York, NY, 1986:35–49.
- 14. Hayashi M, Takamatsu I, Kanno Y, Yoshida T, Abe T, Sato Y, Japanese Calciphylaxis Study Group. A case-control study of calciphylaxis in Japanese end-stage renal disease patients. Nephrol Dial Transplant 2012; 27 :1580–1584.
- 15. Iwagami M, Taniguchi Y, Jin X, Adomi M, Mori T, Hamada S, et al. Association between recorded medical diagnoses and incidence of long-term care needs certification: a case control study using linked medical and long-term care data in two Japanese cities. Annals Clin Epidemiol 2019; 1 :56–68.
- 16. Suissa S, Dell’Aniello S. Time-related biases in pharmacoepidemiology. Pharmacoepidemiol Drug Saf 2020; 29 :1101–1110.
- 17. Thomas LE, Yang S, Wojdyla D, Schaubel DE. Matching with time-dependent treatments: a review and look forward. Stat Med 2020; 39 :2350–2370.
- 18. Greenland S, Morgenstern H. Matching and efficiency in cohort studies. Am J Epidemiol 1990; 131 :151–159.
- 19. Shinozaki T, Mansournia MA, Matsuyama Y. On hazard ratio estimators by proportional hazards models in matched-pair cohort studies. Emerg Themes Epidemiol 2017; 14 :6.
- 20. Sjölander A, Greenland S. Ignoring the matching variables in cohort studies – when is it valid and why? Stat Med 2013; 32 :4696–4708.
- 21. Sutradhar R, Baxter NN, Austin PC. Terminating observation within matched pairs of subjects in a matched cohort analysis: a Monte Carlo simulation study. Stat Med 2016; 35 :294–304.
- 22. Shinozaki T, Mansournia MA. Hazard ratio estimators after terminating observation within matched pairs in sibling and propensity score matched designs. Int J Biostat 2019; 15 .
- 23. Yasunaga H. Introduction to applied statistics—chapter 1 propensity score analysis. Annals Clin Epidemiol 2020; 2 :33–37.
- 24. Abadie A, Imbens GW. Matching on the estimated propensity score. Econometrica 2016; 84 :781–807.
- 25. Shinozaki T, Nojima M. Misuse of regression adjustment for additional confounders following insufficient propensity score balancing. Epidemiology 2019; 30 :541–548.
- 26. Ohbe H, Goto T, Miyamoto Y, Yasunaga H. Risk of cardiovascular events after spouse’s ICU admission. Circulation 2020; 142 :1691–1693.
- 27. Nagasu H, Yano Y, Kanegae H, Heerspink HJL, Nangaku M, Hirakawa Y, et al. Kidney outcomes associated with SGLT2 inhibitors versus other glucose-lowering drugs in real-world clinical practice: the Japan chronic kidney disease database. Diabetes Care 2021; 44 :2542–2551.

Register with J-STAGE for free!
Already have an account? Sign in here
- Open access
- Published: 29 May 2024
Neoadjuvant chemotherapy followed by surgery versus concurrent chemoradiotherapy in patients with stage IIB cervical squamous cell carcinoma: a retrospective cohort study
- Xin-Bin Pan 1 ,
- You-Sheng Wei 2 &
- De-Sheng Yao 2
BMC Cancer volume 24 , Article number: 655 ( 2024 ) Cite this article
92 Accesses
Metrics details
This study aims to compare treatment outcomes between neoadjuvant chemotherapy (NACT) followed by surgery and concurrent chemoradiotherapy (CCRT) in patients with stage IIB cervical squamous cell carcinoma (CSCC).
Materials and methods
We conducted a retrospective cohort study involving patients with stage IIB CSCC treated at Guangxi Medical University Cancer Hospital between June 2012 and June 2019. We compared overall survival (OS), locoregional-free survival (LRFS), and distant metastasis-free survival (DMFS) between the NACT + surgery and CCRT groups.
A total of 257 patients were enrolled: 165 underwent NACT + surgery and 92 received CCRT. Before propensity score matching, the NACT + surgery group exhibited lower 5-year OS (68.2% vs. 85.6%; hazard ratio [HR] = 2.50, 95% confidence interval [CI]: 1.26–4.96; P = 0.009), LRFS (85.2% vs. 96.9%; HR = 5.88, 95% CI: 1.33–25.94; P = 0.019), and DMFS (81.9% vs. 97.4%; HR = 6.65, 95% CI: 1.51–29.23; P = 0.012) compared to the CCRT group. After propensity score matching, OS, LRFS, and DMFS remained worse in the NACT + surgery group compared to the CCRT group.
NACT followed by surgery is associated with decreased OS, LRFS, and DMFS compared to CCRT among patients with stage IIB CSCC.
Peer Review reports
Introduction
Cervical squamous cell carcinoma (CSCC) presents a significant health challenge globally, especially affecting women in developing countries [ 1 ]. In these regions, patients frequently present with advanced stages of the disease [ 2 ]. While concurrent chemoradiotherapy (CCRT) has been established as the standard treatment modality for these locally advanced CSCC [ 3 , 4 ], surgical intervention is also proposed as a viable alternative [ 5 , 6 ]. However, the efficacy of surgery in comparison to CCRT is currently a subject of debate. Divergent studies have reported variable outcomes. Some studies indicate that surgery may yield overall survival (OS) rates comparable to those achieved with CCRT [ 7 , 8 , 9 , 10 ]. In contrast, others indicate worse survival outcomes with surgical approaches [ 11 , 12 ]. Our study is designed to compare the survival outcomes between neoadjuvant chemotherapy (NACT) followed by surgery and CCRT in patients with stage IIB CSCC.
This retrospective cohort study analyzed CSCC patients treated at Guangxi Medical University Cancer Hospital from June 2012 to June 2019. Inclusion criteria were as follows: [ 1 ] pathologically confirmed cervical cancer, [ 2 ] squamous cell carcinoma, [ 3 ] stage IIB according to the International Federation of Gynaecology and Obstetrics (FIGO) staging system [ 13 ], [ 4 ] patients received CCRT or NACT + surgery. Exclusion criteria were as follows: [ 1 ] patients did not receive any treatments, [ 2 ] patients had incomplete data, [ 3 ] patients did not complete treatments, [ 4 ] patients underwent surgery alone, [ 5 ] patients received CCRT combined with neoadjuvant or adjuvant therapies.
Clinical variables collected included Eastern Cooperative Oncology Group (ECOG) status, age, tumor grade, human papillomavirus (HPV) infection status, hemoglobin levels, and tumor diameter. Tumor status, lymph node status, and systemic status were recorded according to clinical, imaging, pathological findings [ 13 ].
Surgical procedures comprised laparoscopic or open hysterectomy, as detailed in our previous studies [ 14 , 15 , 16 ]. Procedures included Piver-Rutledge class III abdominal hysterectomy, bilateral pelvic lymphadenectomy, and lower para-aortic lymph node sampling, all performed by expert gynecologic oncologists. Before surgery, 1 mL of carbon nanoparticles was injected into the cervix surrounding the tumor. The injection process lasted at least 3 min. The first lymph nodes to exhibit black staining after the injection initiation were identified as the sentinel lymph nodes. The number and location of these sentinel lymph nodes were recorded. Subsequently, the sentinel lymph nodes were excised and sent for pathological examination.
Prior to surgery, patients underwent 1 to 3 cycles of platinum-based NACT every three weeks. The surgery was scheduled 3–4 weeks after the final NACT cycle, based on the clinical response. Post-surgery, patients identified with risk prognostic factors were administered tailored adjuvant therapies. These therapies included options like chemotherapy, radiotherapy, or concurrent chemoradiotherapy [ 17 , 18 ]. The prognostic risk factors guiding these decisions included more than one third stromal invasion, capillary lymphatic space involvement, a tumor diameter exceeding 4 cm, positive pelvic lymph nodes, positive surgical margins, and microscopic involvement of the parametrium.
- Concurrent chemoradiotherapy
Radiotherapy combined pelvic external beam radiotherapy (48–50 Gy over 24–25 fractions using intensity-modulated radiotherapy) with high-dose-rate intracavitary brachytherapy (28–35 Gy over 4–5 fractions targeting the high-risk clinical target volume). Cone beam computed tomography was used for daily verification during the first week of treatment, followed by weekly verification thereafter.
The radiation doses were carefully adjusted to minimize impact on surrounding structures like the tumor, rectum, and bladder. To standardize bladder filling and reduce interaction motion, we employed a systematic drinking protocol. Patients were instructed to drink 500 mL of water 45 min before each radiotherapy session. This protocol was consistently followed to ensure reproducible bladder volumes, which is critical for maintaining the precision of radiation delivery and minimizing dose variations to surrounding organs at risk.
Concurrent chemotherapy involved weekly intravenous cisplatin (30–40 mg/m2/d1) or nedaplatin (50 mg/m2/d1) administered during the external beam radiotherapy period.
The primary endpoint of our study was OS, defined as the duration from diagnosis to death from any cause. Secondary endpoints were locoregional-free survival (LRFS) and distant metastasis-free survival (DMFS), which measured the time from diagnosis to either locoregional recurrence or distant metastasis, respectively.
Statistical analysis
Patients were divided into two groups: those receiving NACT + surgery, and those undergoing CCRT. We categorized continuous variables like age and hemoglobin levels at their median values. Tumor diameter, a continuous variable, was grouped at 4 cm [ 19 ]. Categorical variables, including ECOG status, age, tumor grade, HPV infection status, hemoglobin levels, and tumor diameter, were analyzed using the χ 2 test or Fisher’s exact test.
For survival analysis, we employed the Kaplan-Meier method, using log-rank tests to compare OS, LRFS, and DMFS between NACT + surgery and CCRT groups. Multivariable proportional hazards models, adjusted for ECOG status, age, tumor grade, HPV infection status, hemoglobin levels, tumor diameter, and treatment modalities, were used to identify independent prognostic factors. The results were presented as hazard ratios (HRs) with 95% confidence intervals (CIs).
To minimize selection bias between the NACT + surgery and CCRT groups, we used a matched case-control approach through propensity score matching (PSM). The scores were calculated using a logistic regression model with CCRT as the dependent variable. We matched cases one-to-one without replacement, based on nearest-neighbor matching on the logit of the propensity score, considering confounding factors and a caliper of 0.01.
All statistical analyses were performed using SPSS Statistics Version 26.0 (IBM Co., Armonk, NY, USA) and R software (version 4.2.2). A two-tailed P value below 0.05 was considered statistically significant.
Ethical approval for this study was obtained from the Guangxi Medical University Cancer Hospital Ethics Committee. The study was conducted in compliance with the principles outlined in the Declaration of Helsinki. However, informed consent was not obtained due to the retrospective nature of the study.
Patient characteristics
The selection process is illustrated in Fig. 1 . Our study included 257 patients: 165 (64.2%) underwent NACT + surgery and 92 (35.8%) received CCRT. Patient characteristics, both before and after PSM, are detailed in Table 1 . Prior to PSM, baseline characteristics like age, ECOG status, and HPV infection status showed imbalances between the NACT + surgery and CCRT groups. After PSM, 69 patients who received NACT + surgery and 69 patients who received CCRT were matched. Patient characteristics showing no significant differences across all covariates ( P > 0.05).
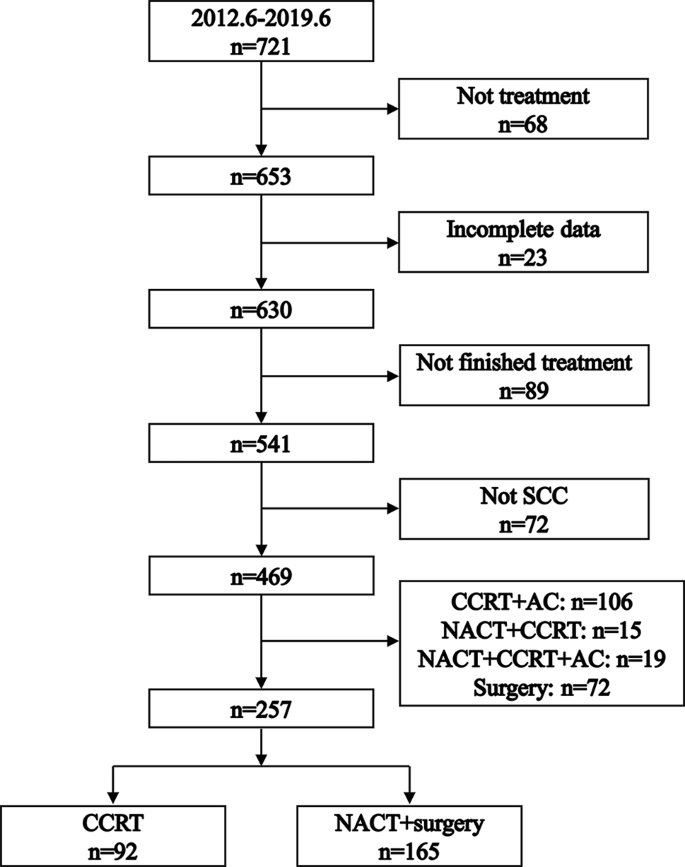
Flowchart illustrating the process of patient selection. SCC: squamous cell carcinoma. NACT: neoadjuvant chemotherapy. CCRT: concurrent chemoradiotherapy. AC: adjuvant chemotherapy
Within the CCRT group, the median number of concurrent chemotherapy cycles was 4 (interquartile range: 3–5 cycles). In the NACT + surgery group, 76 (46.1%) patients receive NACT + surgery alone, 51 (30.9%) patents receive radiotherapy after NACT + surgery, 5 (3.0%) patients received chemotherapy after NACT + surgery, and 33 (20.0%) patients received CCRT after NACT + surgery. Post-surgery, 68 (41.2%) patients in the NACT + surgery group were diagnosed with lymph node metastases.
Logistic regression for factors associated with NACT + surgery
Figure 2 presents the logistic regression analysis results, exploring factors influencing the choice of NACT + surgery. The analysis revealed a significant association between the selection of NACT + surgery and both age and ECOG status. Specifically, patients older than 54 years were less likely to undergo NACT + surgery (odds ratio = 0.37, 95% CI: 0.20–0.66; P < 0.001). Similarly, a lower likelihood of opting for NACT + surgery was observed in patients with an ECOG score of 1 (odds ratio = 0.51, 95% CI: 0.26–0.99; P = 0.048).
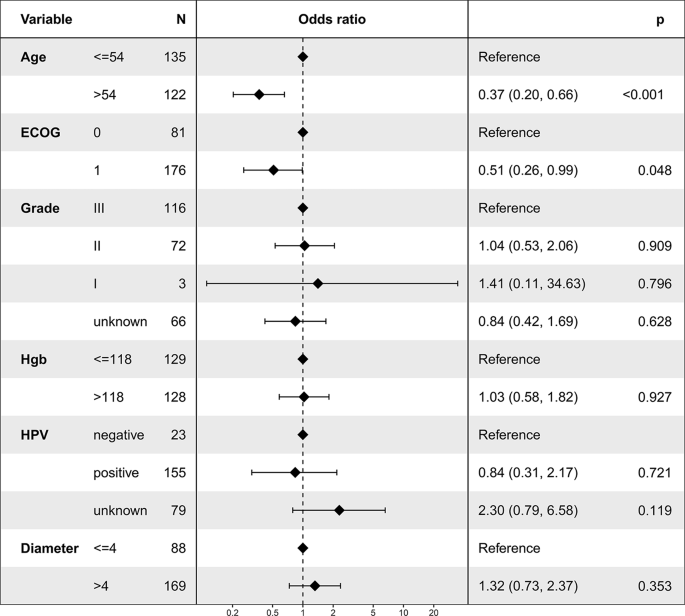
Logistic regression analysis depicting factors associated with the utilization of neoadjuvant chemotherapy followed by surgery
Overall survival
Before PSM, the 5-year OS rates were 68.2% in the NACT + surgery group and 85.6% in the CCRT group ( P = 0.004, Fig. 3 A). Multivariable proportional hazards models revealed that NACT + surgery was an independent risk prognostic factor for OS (HR = 2.50, 95% CI: 1.26–4.96; P = 0.009, Table 2 ).
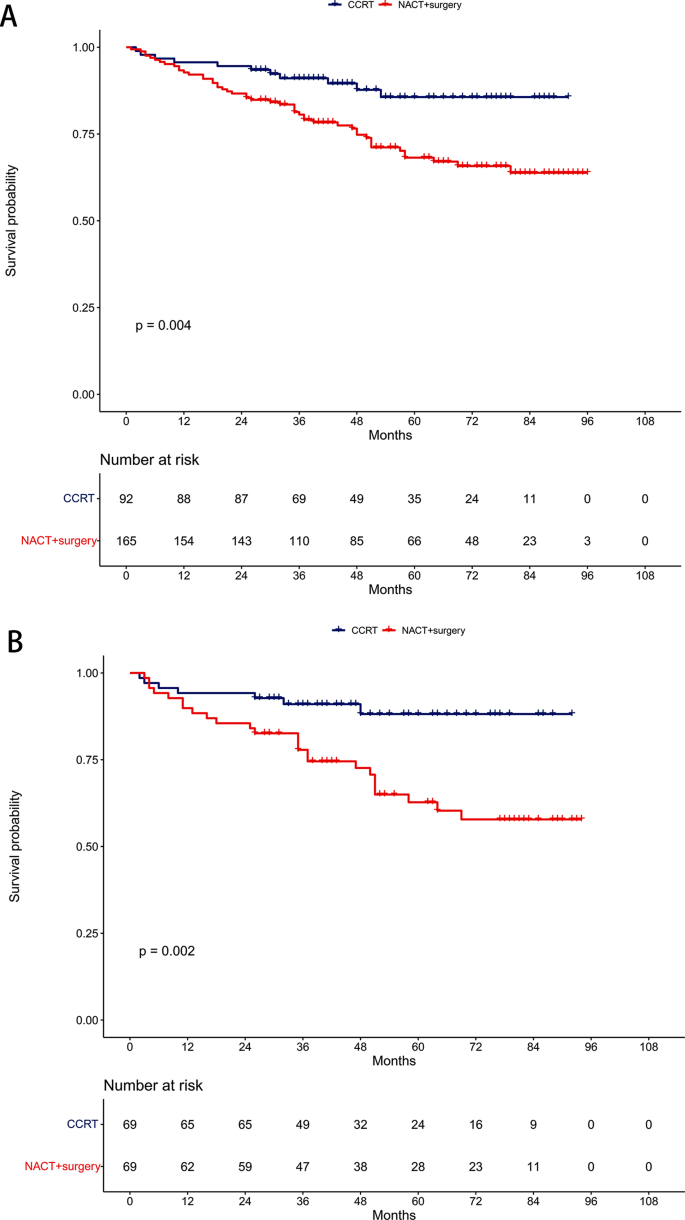
Comparison of overall survival between the neoadjuvant chemotherapy followed by surgery and concurrent chemoradiotherapy groups. ( A ) Unmatched cohort. ( B ) Propensity-matched cohort
After PSM, the 5-year OS rates were 62.7% in the NACT + surgery group and 88.2% in the CCRT group ( P = 0.002, Fig. 3 B). Similarly, NACT + surgery remained an independent risk prognostic factor for OS in multivariable proportional hazards models (HR = 3.67, 95% CI: 1.58–8.52; P = 0.003, Table 3 ).
Locoregional-free survival
Pre-PSM, the 5-year LRFS rates were 85.2% in the NACT + surgery group and 96.9% in the CCRT group ( P = 0.009, Fig. 4 A). Multivariable proportional hazards models demonstrated that NACT + surgery was an independent risk prognostic factor for LRFS (HR = 5.88, 95% CI: 1.33–25.94; P = 0.019, Table 2 ).

Comparison of locoregional-free survival between the neoadjuvant chemotherapy followed by surgery and concurrent chemoradiotherapy groups. ( A ) Unmatched cohort. ( B ) Propensity-matched cohort
Post-PSM, the 5-year LRFS rates were 84.7% in the NACT + surgery group and 96.9% in the CCRT group ( P = 0.018, Fig. 4 B). NACT + surgery retained its significance as an independent risk prognostic factor for LRFS in multivariable proportional hazards models (HR = 8.31, 95% CI: 1.03–67.32; P = 0.047, Table 3 ).
Distant metastasis-free survival
Before PSM, the 5-year DMFS rates were 81.9% in the NACT + surgery group and 97.4% in the CCRT group ( P = 0.002, Fig. 5 A). Multivariable proportional hazards models revealed NACT + surgery as an independent risk prognostic factor for DMFS (HR = 6.65, 95% CI: 1.51–29.23; P = 0.012, Table 2 ).
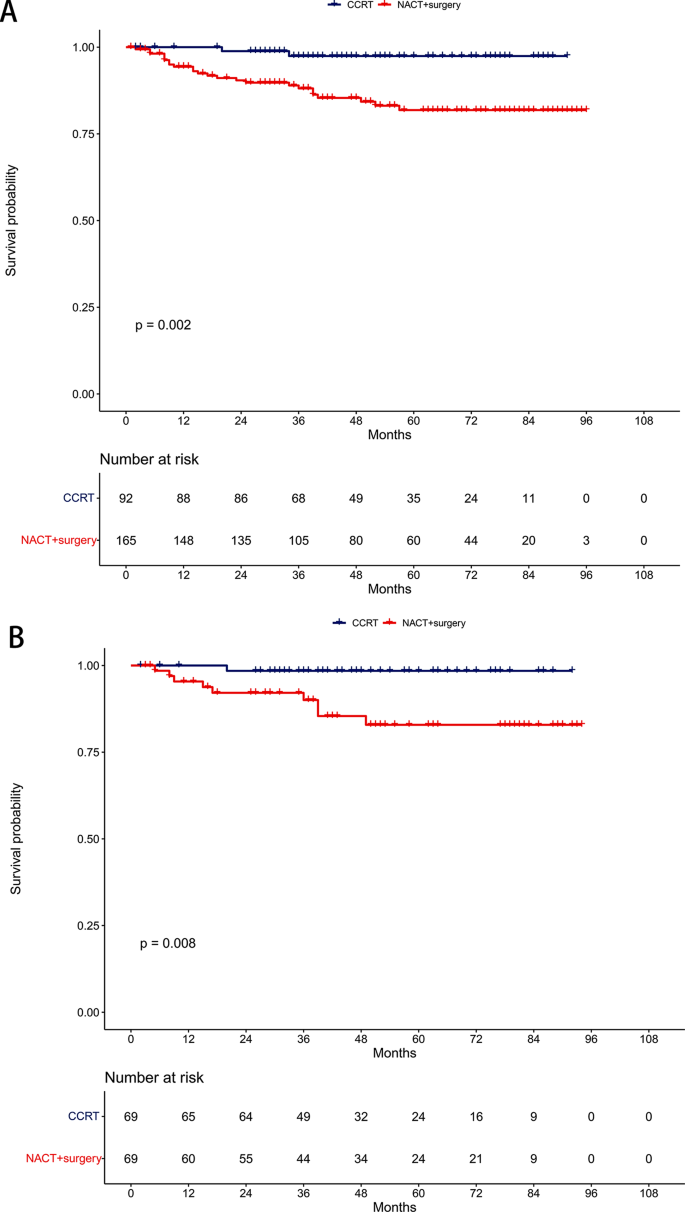
Comparison of distant metastasis-free survival between the neoadjuvant chemotherapy followed by surgery and concurrent chemoradiotherapy groups. ( A ) Unmatched cohort. ( B ) Propensity-matched cohort
After PSM, the 5-year DMFS rates were 82.9% in the NACT + surgery group and 98.5% in the CCRT group ( P = 0.008, Fig. 5 B). NACT + surgery continued to be an independent risk prognostic factor for DMFS in multivariable proportional hazards models (HR = 10.94, 95% CI: 1.34–89.21; P = 0.026, Table 3 ).
Our study offered a pivotal understanding that NACT before surgery did not improve survival outcomes in stage IIB CSCC patients. This aligns with the existing perspective that stage IIB CSCC is an unresectable disease [ 20 ]. Consequently, CCRT remains the fundamental treatment strategy. These results strongly suggested that surgery as an initial radical approach should not be considered for this patient subgroup [ 21 ].
Our results may suggest that the delay in surgery due to NACT might compromise survival rates. The initial decrease in 5-year LRFS and DMFS associated with NACT + surgery could adversely affect OS, indicating that NACT-induced tumor size reduction may not be adequate for effective radical surgery in this specific patient group [ 22 ].
Despite being the preferred treatment, CCRT for stage IIB CSCC may lead to significant long-term radiation-induced complications, including ovarian failure, vaginal fibrosis, enteritis, fistulas, bowel obstruction, and lymphedema [ 23 ]. These adverse effects significantly impact the quality of life for patients during long-term survival [ 24 ]. This reality might incline clinicians toward considering surgical interventions for these patients. However, recent studies indicate that achieving an optimal dose distribution, compliant with the treatment planning objectives of the EMBRACE II protocol, is more feasible when the external beam radiotherapy dose is limited to 45 Gy [ 25 ]. Moreover, the systematic use of an interstitial brachytherapy component can increase the dose delivered to 98% of the volume from 83 ± 14 Gy to 92 ± 13 Gy without increasing the dose to organs at risk. Adhering to these updated international guidelines could substantially reduce the complications associated with CCRT and increase the locoregional control rates [ 13 ].
However, the approach to treating stage IIB CSCC is not always consistent in clinical practice, primarily due to the subjective nature of staging, which relies on physical examination. This variability often leads to different surgical decisions among clinicians [ 26 , 27 ]. In certain cases, experts might recommend surgery, basing their decision on specific factors such as tumor size, histopathological grade, and lymph node status [ 28 ].
Moreover, recent advancements in chemotherapy regimens, particularly those involving platinum and cisplatin, have shown notable effectiveness in CSCC [ 29 ]. Platinum-based NACT has been found to reduce the primary tumor burden, which could lead to a higher rate of complete resection. Additionally, Platinum-based NACT is believed to have the capability to prevent cancer cell implantation and to eradicate circulating cancer cells, thereby potentially diminishing subclinical metastasis. This could lead to improved DMFS, a hypothesis that is gaining support from various studies [ 30 , 31 , 32 ].
The primary aim of using NACT is to enhance treatment outcomes compared to CCRT. However, findings from a single-center, phase III, randomized controlled trial presented a different picture [ 12 ]. This study reported a 5-year disease-free survival of 69.3% in the NACT + surgery group, compared to 76.7% in the CCRT group (HR = 1.38, 95% CI: 1.02–1.87; P = 0.038). Interestingly, the corresponding 5-year OS rates were quite similar: 75.4% for NACT + surgery and 74.7% for CCRT (HR = 1.025, 95% CI: 0.752–1.398; P = 0.87). This suggested that NACT + surgery did not significantly improve survival rates over CCRT.
A notable observation from this trial was that the reduced disease-free survival in the NACT + surgery group did not lead to a worse OS. This could be attributed to the effectiveness of salvage treatments following recurrence. It is important to note that a majority of first recurrences (102 out of 162 recurrences, constituting 62.96%) were localized, emphasizing the critical role of local control. After recurrence, 30.0% of patients in the NACT + surgery group received local radiotherapy, compared to just 11.0% in the CCRT group. This finding underscored the importance of local control in managing recurrent CSCC and illustrated the complexity inherent in treatment decisions and their long-term implications.
A major strength of our study was the consistent quality of treatment delivery by a multidisciplinary team of experts. However, its retrospective nature introduced potential limitations, including possible confounders between the NACT + surgery and CCRT groups. Although we employed PSM and multivariable proportional hazards models to address these biases, our findings need to be validated by larger-scale studies conducted at diverse centers.
In conclusion, our research highlights that NACT + surgery decreased OS, LRFS, and DMFS compared to CCRT in treating stage IIB CSCC, emphasizing the need for cautious consideration in treatment planning.
Data availability
The data are available from the corresponding author upon request.
Abbreviations
- Cervical squamous cell carcinoma
- Neoadjuvant chemotherapy
The International Federation of Gynaecology and Obstetrics
Eastern Cooperative Oncology Group
Human papilloma virus
Propensity score matching
Hazard ratio
Confidence interval
Sung H, Ferlay J, Siegel RL, Laversanne M, Soerjomataram I, Jemal A, et al. Global Cancer statistics 2020: GLOBOCAN estimates of incidence and Mortality Worldwide for 36 cancers in 185 countries. CA Cancer J Clin. 2021;71(3):209–49.
Article PubMed Google Scholar
Zhang M, Zhong Y, Zhao Z, Huang Z, Zhang X, Li C, et al. Cervical Cancer Screening Rates among Chinese Women - China, 2015. China CDC Wkly. 2020;2(26):481–6.
Article CAS PubMed PubMed Central Google Scholar
Rose PG, Bundy BN, Watkins EB, Thigpen JT, Deppe G, Maiman MA, et al. Concurrent cisplatin-based radiotherapy and chemotherapy for locally advanced cervical cancer. N Engl J Med. 1999;340(15):1144–53.
Article CAS PubMed Google Scholar
National Health Commission Of The People’s Republic Of C. Chinese guidelines for diagnosis and treatment of cervical cancer 2018 (English version). Chin J Cancer Res. 2019;31(2):295–305.
Article Google Scholar
Marth C, Landoni F, Mahner S, McCormack M, Gonzalez-Martin A, Colombo N, et al. Cervical cancer: ESMO Clinical Practice guidelines for diagnosis, treatment and follow-up. Ann Oncol. 2017;28(suppl4):iv72–83.
Ebina Y, Mikami M, Nagase S, Tabata T, Kaneuchi M, Tashiro H, et al. Japan Society of Gynecologic Oncology guidelines 2017 for the treatment of uterine cervical cancer. Int J Clin Oncol. 2019;24(1):1–19.
Kokka F, Bryant A, Olaitan A, Brockbank E, Powell M, Oram D. Hysterectomy with radiotherapy or chemotherapy or both for women with locally advanced cervical cancer. Cochrane Database Syst Rev. 2022;8(8):CD010260.
PubMed Google Scholar
Ye Q, Yang Y, Tang X, Li J, Li X, Zhang Y. Neoadjuvant Chemotherapy followed by radical surgery versus Radiotherapy (with or without chemotherapy) in patients with stage IB2, IIA, or IIB cervical Cancer: a systematic review and Meta-analysis. Dis Markers. 2020;2020:7415056.
Article PubMed PubMed Central Google Scholar
Hu Y, Han Y, Shen Y, Chen J, Chen Y, Chen Y, et al. Neoadjuvant chemotherapy for patients with international federation of gynecology and obstetrics stages IB3 and IIA2 cervical cancer: a multicenter prospective trial. BMC Cancer. 2022;22(1):1270.
Zou W, Han Y, Zhang Y, Hu C, Feng Y, Zhang H, et al. Neoadjuvant chemotherapy plus surgery versus concurrent chemoradiotherapy in stage IB2-IIB cervical cancer: a systematic review and meta-analysis. PLoS ONE. 2019;14(11):e0225264.
Miriyala R, Mahantshetty U, Maheshwari A, Gupta S. Neoadjuvant chemotherapy followed by surgery in cervical cancer: past, present and future. Int J Gynecol cancer: Official J Int Gynecol Cancer Soc. 2022;32(3):260–5.
Gupta S, Maheshwari A, Parab P, Mahantshetty U, Hawaldar R, Sastri Chopra S, et al. Neoadjuvant Chemotherapy followed by radical surgery Versus Concomitant Chemotherapy and Radiotherapy in patients with Stage IB2, IIA, or IIB squamous cervical Cancer: a Randomized Controlled Trial. J Clin Oncol. 2018;36(16):1548–55.
Cibula D, Raspollini MR, Planchamp F, Centeno C, Chargari C, Felix A, et al. ESGO/ESTRO/ESP guidelines for the management of patients with cervical cancer - update 2023. Int J Gynecol Cancer. 2023;33(5):649–66.
Lu Y, Wei JY, Yao DS, Pan ZM, Yao Y. Application of carbon nanoparticles in laparoscopic sentinel lymph node detection in patients with early-stage cervical cancer. PLoS ONE. 2017;12(9):e0183834.
Wei YS, Yao DS, Long Y. Evaluation of the association between perineural invasion and clinical and histopathological features of cervical cancer. Mol Clin Oncol. 2016;5(3):307–11.
Long Y, Yao DS, Wei YS, Wei CH, Chen XY. Prognostic significance of perineural invasion in vulvar squamous cell carcinoma. Cancer Manag Res. 2019;11:4461–9.
Peters WA 3rd, Liu PY, Barrett RJ 2nd, Stock RJ, Monk BJ, Berek JS, et al. Concurrent chemotherapy and pelvic radiation therapy compared with pelvic radiation therapy alone as adjuvant therapy after radical surgery in high-risk early-stage cancer of the cervix. J Clin Oncology: Official J Am Soc Clin Oncol. 2000;18(8):1606–13.
Article CAS Google Scholar
Rotman M, Sedlis A, Piedmonte MR, Bundy B, Lentz SS, Muderspach LI, et al. A phase III randomized trial of postoperative pelvic irradiation in Stage IB cervical carcinoma with poor prognostic features: follow-up of a gynecologic oncology group study. Int J Radiat Oncol Biol Phys. 2006;65(1):169–76.
Carlson JA, Rusthoven C, DeWitt PE, Davidson SA, Schefter TE, Fisher CM. Are we appropriately selecting therapy for patients with cervical cancer? Longitudinal patterns-of-care analysis for stage IB-IIB cervical cancer. Int J Radiat Oncol Biol Phys. 2014;90(4):786–93.
Bhatla N, Aoki D, Sharma DN, Sankaranarayanan R. Cancer of the cervix uteri. Int J Gynaecol Obstet. 2018;143(Suppl 2):22–36.
Borghi C, Biagioli E, Mauro J, Roberto A, Borghese M, Buda A. Neoadjuvant chemotherapy prior to radical hysterectomy in locally advanced cervical cancer: a systematic review and meta-analysis. Int J Gynecol Cancer. 2023.
Chidambaram S, Owen R, Sgromo B, Chmura M, Kisiel A, Evans R et al. Delayed Surgical Intervention After Chemoradiotherapy in Esophageal Cancer: (DICE) Study. Annals of surgery. 2023.
Hoekman EJ, Knoester D, Peters AAW, Jansen FW, de Kroon CD, Hilders C. Ovarian survival after pelvic radiation: transposition until the age of 35 years. Arch Gynecol Obstet. 2018;298(5):1001–7.
Bergmark K, Avall-Lundqvist E, Dickman PW, Henningsohn L, Steineck G. Vaginal changes and sexuality in women with a history of cervical cancer. N Engl J Med. 1999;340(18):1383–9.
Chargari C, Tanderup K, Planchamp F, Chiva L, Humphrey P, Sturdza A, et al. ESGO/ESTRO quality indicators for radiation therapy of cervical cancer. Int J Gynecol Cancer. 2023;33(6):862–75.
Fujii S, Takakura K, Matsumura N, Higuchi T, Yura S, Mandai M, et al. Precise anatomy of the vesico-uterine ligament for radical hysterectomy. Gynecol Oncol. 2007;104(1):186–91.
Swailes AL, Gockley A, Phaeton R, Kesterson JP. The Wertheim hysterectomy: development, modifications, and impact in the present day. Gynecol Oncol. 2017;145(1):3–8.
Mallmann P, Mallmann C. Neoadjuvant and Adjuvant Chemotherapy of Cervical Cancer. Oncol Res Treat. 2016;39(9):522–4.
Moore DH, Blessing JA, McQuellon RP, Thaler HT, Cella D, Benda J, et al. Phase III study of cisplatin with or without paclitaxel in stage IVB, recurrent, or persistent squamous cell carcinoma of the cervix: a gynecologic oncology group study. J Clin Oncology: Official J Am Soc Clin Oncol. 2004;22(15):3113–9.
Rydzewska L, Tierney J, Vale CL, Symonds PR. Neoadjuvant chemotherapy plus surgery versus surgery for cervical cancer. Cochrane Database Syst Rev. 2012;12(12):CD007406.
Chen H, Liang C, Zhang L, Huang S, Wu X. Clinical efficacy of modified preoperative neoadjuvant chemotherapy in the treatment of locally advanced (stage IB2 to IIB) cervical cancer: randomized study. Gynecol Oncol. 2008;110(3):308–15.
Huang X, Lan C, Huang H, Zhang Y, Huang H, Cao X, et al. Neoadjuvant docetaxel combined with cisplatin and followed by radical surgery for the treatment of locally advanced (stage IB2 - IIB) cervical cancer: preliminary results of a single-institution experience. Expert Opin Pharmacother. 2011;12(2):165–73.
Download references
Acknowledgements
Not Applicable.
Author information
Authors and affiliations.
Department of Radiation Oncology, Guangxi Medical University Cancer Hospital, Nanning, Guangxi, 530021, P.R. China
Xin-Bin Pan
Department of Gynecologic Oncology, Guangxi Medical University Cancer Hospital, No. 71 Hedi Road, Qingxiu District, Nanning, Guangxi, 530021, P.R. China
Yan Lu, You-Sheng Wei & De-Sheng Yao
You can also search for this author in PubMed Google Scholar
Contributions
Conceptualization: X-B.P., Methodology: Y.L., Formal Analysis: Y-S.W., Investigation: X-B.P., Resources: Y.L., Validation: Y.L. and Y-S.W., Writing-Original Draft Preparation: X-B.P., Writing-Review & Editing: D-S.Y.
Corresponding author
Correspondence to De-Sheng Yao .
Ethics declarations
Ethics approval and consent to participate.
The requirement for ethics approval was approved by the ethics committee/Institutional Review Board of Guangxi Medical University Cancer Hospital. The requirement for informed consent was waived by the ethics committee/Institutional Review Board of Guangxi Medical University Cancer Hospital. All methods were performed in accordance with the relevant guidelines and regulations.

Consent for publication
Not applicable.
Competing interests
The authors declare no competing interests.
Additional information
Publisher’s note.
Springer Nature remains neutral with regard to jurisdictional claims in published maps and institutional affiliations.
Rights and permissions
Open Access This article is licensed under a Creative Commons Attribution 4.0 International License, which permits use, sharing, adaptation, distribution and reproduction in any medium or format, as long as you give appropriate credit to the original author(s) and the source, provide a link to the Creative Commons licence, and indicate if changes were made. The images or other third party material in this article are included in the article’s Creative Commons licence, unless indicated otherwise in a credit line to the material. If material is not included in the article’s Creative Commons licence and your intended use is not permitted by statutory regulation or exceeds the permitted use, you will need to obtain permission directly from the copyright holder. To view a copy of this licence, visit http://creativecommons.org/licenses/by/4.0/ . The Creative Commons Public Domain Dedication waiver ( http://creativecommons.org/publicdomain/zero/1.0/ ) applies to the data made available in this article, unless otherwise stated in a credit line to the data.
Reprints and permissions
About this article
Cite this article.
Pan, XB., Lu, Y., Wei, YS. et al. Neoadjuvant chemotherapy followed by surgery versus concurrent chemoradiotherapy in patients with stage IIB cervical squamous cell carcinoma: a retrospective cohort study. BMC Cancer 24 , 655 (2024). https://doi.org/10.1186/s12885-024-12411-6
Download citation
Received : 18 January 2024
Accepted : 22 May 2024
Published : 29 May 2024
DOI : https://doi.org/10.1186/s12885-024-12411-6
Share this article
Anyone you share the following link with will be able to read this content:
Sorry, a shareable link is not currently available for this article.
Provided by the Springer Nature SharedIt content-sharing initiative
ISSN: 1471-2407
- Submission enquiries: [email protected]
- General enquiries: [email protected]
Thank you for visiting nature.com. You are using a browser version with limited support for CSS. To obtain the best experience, we recommend you use a more up to date browser (or turn off compatibility mode in Internet Explorer). In the meantime, to ensure continued support, we are displaying the site without styles and JavaScript.
- View all journals
- My Account Login
- Explore content
- About the journal
- Publish with us
- Sign up for alerts
- Open access
- Published: 27 May 2024
Acute and post-acute respiratory complications of SARS-CoV-2 infection: population-based cohort study in South Korea and Japan
- Yujin Choi ORCID: orcid.org/0009-0002-4131-712X 1 , 2 na1 ,
- Hyeon Jin Kim 1 , 3 na1 ,
- Jaeyu Park 1 , 3 na1 ,
- Myeongcheol Lee 1 , 3 ,
- Sunyoung Kim 4 ,
- Ai Koyanagi 5 ,
- Lee Smith ORCID: orcid.org/0000-0002-5340-9833 6 ,
- Min Seo Kim ORCID: orcid.org/0000-0003-2115-7835 7 ,
- Masoud Rahmati ORCID: orcid.org/0000-0003-4792-027X 8 , 9 , 10 ,
- Hayeon Lee ORCID: orcid.org/0009-0000-2403-6241 1 na2 ,
- Jiseung Kang ORCID: orcid.org/0000-0002-3734-7572 11 , 12 na2 &
- Dong Keon Yon ORCID: orcid.org/0000-0003-1628-9948 1 , 3 , 13 na2
Nature Communications volume 15 , Article number: 4499 ( 2024 ) Cite this article
1664 Accesses
79 Altmetric
Metrics details
- Epidemiology
- Infectious diseases
- Respiratory tract diseases
Considering the significant burden of post-acute COVID-19 conditions among patients infected with SARS-CoV-2, we aimed to identify the risk of acute respiratory complications or post-acute respiratory sequelae. A binational population-based cohort study was conducted to analyze the risk of acute respiratory complications or post-acute respiratory sequelae after SARS-CoV-2 infection. We used a Korean nationwide claim-based cohort (K-COV-N; n = 2,312,748; main cohort) and a Japanese claim-based cohort (JMDC; n = 3,115,606; replication cohort) after multi-to-one propensity score matching. Among 2,312,748 Korean participants (mean age, 47.2 years [SD, 15.6]; 1,109,708 [48.0%] female), 17.1% (394,598/2,312,748) were infected with SARS-CoV-2. The risk of acute respiratory complications or post-acute respiratory sequelae is significantly increased in people with SARS-CoV-2 infection compared to the general population (acute respiratory complications: HR, 8.06 [95% CI, 6.92-9.38]; post-acute respiratory sequelae: 1.68 [1.62-1.75]), and the risk increased with increasing COVID-19 severity. We identified COVID-19 vaccination as an attenuating factor, showing a protective association against acute or post-acute respiratory conditions. Furthermore, while the excess post-acute risk diminished with time following SARS-CoV-2 infection, it persisted beyond 6 months post-infection. The replication cohort showed a similar pattern in the association. Our study comprehensively evaluates respiratory complications in post-COVID-19 conditions, considering attenuating factors such as vaccination status, post-infection duration, COVID-19 severity, and specific respiratory conditions.
Similar content being viewed by others
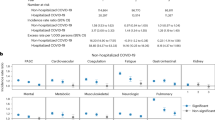
Three-year outcomes of post-acute sequelae of COVID-19
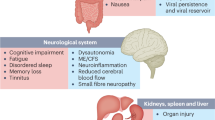
Long COVID: major findings, mechanisms and recommendations
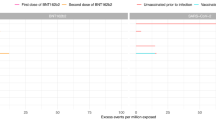
Safety outcomes following COVID-19 vaccination and infection in 5.1 million children in England
Introduction.
Severe acute respiratory syndrome coronavirus 2 (SARS-CoV-2), responsible for the COVID-19 pandemic, has caused global attention not only due to its immediate symptoms derived from the virus itself but also owing to subsequent physical and mental health sequelae 1 . Since 2020, there have been over 761 million reported cases of SARS-CoV-2 infections, with ~6.8 million deaths among infected individuals 2 . Despite its relatively low fatality rate of ~1.3% 3 , ~10% of patients with SARS-CoV-2 infection report persistent, long-lasting comorbidities after infection termed post-acute COVID-19 condition 4 . Post-acute COVID-19 condition refers to persistent or new-onset health outcomes that last more than a month since SARS-CoV-2 infection, including both short-term (4–12 weeks) and long-term (>12 weeks) symptoms and sequelae 5 .
Given the nature of the SARS-CoV-2, the infection can trigger adverse effects on the respiratory system and post-acute COVID-19 respiratory sequelae. One previous study highlighted the association between acute respiratory complication or post-acute respiratory sequelae and COVID-19 6 . Acute respiratory complication is an umbrella term that describes illnesses that affect the respiratory system in a sudden onset 7 , 8 . Post-acute respiratory sequelae refers to a broad category of long-term non-infectious respiratory diseases that affect the lungs and airways 7 , 8 . For perspective, the influenza virus is also a well-known viral inducer of respiratory failure 9 . However, insufficient attention has been given to the impact of SARS-CoV-2 infection on acute respiratory complications or post-acute respiratory sequelae in comparison with the influenza infection as a common respiratory viral infection.
Therefore, by using a binational, large-scale, long-term, population-based database with more than 22 million participants in South Korea and Japan, we aimed to investigate the impact of SARS-CoV-2 infection on pathological developments of acute respiratory complication or post-acute respiratory sequelae. We also examined whether COVID-19 vaccinations offer protection against COVID-19-related respiratory outcomes. Furthermore, we analyze the comparison of complications following SARS-CoV-2 infection versus following influenza infection.
In the main cohort, there were a total of 10,027,506 participants with a mean age of 48.4 (standard deviation [SD], 13.4) years, of which 49.9% (5,000,621/10,027,506) were female ( Table S1 ) . The replication cohort includes 4,909,861 participants with a mean age of 46.8 (SD, 11.9) years and 38.3% (1,882,174/4,909,861) females ( Table S2 ) . Table 1 shows the baseline characteristics of the 1:5 propensity score matched cohort of South Korea. After 1:5 propensity score matching based on SARS-CoV-2 infection, we identified 82.9% (1,918,150/2,312,748) of participants without SARS-CoV-2 infection and 17.1% (394,598/2,312,748) of participants with SARS-CoV-2 infection, respectively.
In the 1:3 propensity score-matched replication cohort, 74.4% (2,318,505/3,115,606) of participants without SARS-CoV-2 infection and 25.6% (797,101/3,115,606) of participants with SARS-CoV-2 infection were included in our final analyses (Table S3 ). The standardized mean differences (SMD) of all matching covariates in both multi-to-one propensity score-matched main and replication cohorts were smaller than 0.1 (Table 1 ).
In the main and replication cohorts, individuals with SARS-CoV-2 infection had a higher adjusted hazard ratio (HR) for post-acute respiratory sequelae compared to the general population (main: HR, 1.68 [95% confidence interval (CI), 1.62–1.75]; replication: HR, 3.32 [95% CI, 3.27–3.37]) in Table 2 . Furthermore, patients with SARS-CoV-2 infection had an increased risk for acute respiratory complication compared to non-infected controls (main: HR, 8.06 [95% CI, 6.92–9.38]; replication: HR, 4.17 [95% CI, 3.90–4.45]). When directly comparing the risk for acute respiratory complication between SARS-CoV-2 and influenza infections, SARS-CoV-2 infection was significantly associated with an increased risk (main: HR, 4.32 [95% CI, 2.73–6.83]; replication: HR, 6.51 [95% CI, 5.38–7.87]) in Tables S4 – S6 .
Relative to the general population, patients with SARS-CoV-2 infection had significantly increased risk for several subtypes of post-acute respiratory sequelae, including chronic respiratory failure (main: HR, 8.92 [95% CI, 4.92-16.17]; replication: HR, 7.55 [95% CI, 6.35-8.97]), chronic obstructive pulmonary disease (COPD), emphysema, asthma, pulmonary sarcoidosis, and interstitial lung disease (main: HR, 10.38 [95% CI, 8.75-12.31]; replication: HR, 4.75 [95% CI, 4.54-4.97]) in Table 3 . Notably, the risk for acute respiratory complication, including aspergillosis pneumonia (main: HR, 6.85 [95% CI, 3.48-13.50]; replication: HR, 4.97 [95% CI, 4.26-5.79]), pneumothorax, acute respiratory failure (main: HR, 112.04 [95% CI, 64.00-196.16]; replication: HR, 6.49 [95% CI, 6.32-6.65]) showed an increase in patients with SARS-CoV-2 infection compared to the general population. This tendency of increased risk for several subtypes of respiratory diseases was also shown when compared to patients with influenza infection and the overlap-weighted cohort. (Tables S7 – S10 ). Estimates of marginal prevalence showed that patients with COVID-19 had a higher prevalence compared to the general population (Tables S11 and S12 ).
The risk of acute respiratory complication showed decreasing trends according to the number of SARS-CoV-2 vaccinations from individuals after once receiving vaccination (HR, 0.51 [95% CI, 0.38-0.68]) to those with two or more vaccinations (HR, 0.24 [95% CI, 0.19-0.30]). Interestingly, mixed types of vaccination showed the lowest risk of developing post-acute respiratory sequelae of all SARS-CoV-2 vaccination methods (HR, 0.18 [95% CI, 0.08-0.38]). The risks of acute respiratory complications were higher in patients with moderate to severe COVID-19 symptoms (HR, 39.54 [95% CI, 33.54-46.62]). Both the original strain and the delta variant of SARS-CoV-2 were shown to have a higher risk of acute respiratory complications (original strain: HR, 9.21 [95% CI, 7.19-11.80]; delta strain: HR, 7.44 [95% CI, 6.13-9.03]). In addition, the risk for post-acute respiratory sequelae also exhibited a similar pattern (Table 4 and S 13 ).
Table 5 shows the risk of developing acute respiratory complications or post-acute respiratory sequelae based on how long it has been since the participant was infected with SARS-CoV-2 compared to the general population. The analysis of post-acute respiratory sequelae did not include the data of the first month of SARS-CoV-2 infection. The first 3 months after infection with SARS-CoV-2 had the highest risk of developing post-acute respiratory sequelae (main: HR, 2.51 [95% CI, 2.38-2.64]; replication: HR, 4.40 [95% CI, 4.30-4.51]). With increasing duration post-SARS-CoV-2 infection, the risk of post-acute respiratory sequelae significantly decreased, but the risk remained even after 6 months (main: HR, 1.10 [95% CI, 1.01-1.19]; replication: HR, 2.67 [95% CI, 2.61-2.73]). HR of time attenuation effect after SARS-CoV-2 infection showed significance compared to influenza infection likewise (Table S14 ). Similar associations were observed in the stratification analysis according to sex, age, household income, Charlson comorbidity index (CCI), body mass index (BMI), alcohol consumption, physical activity, region of residence, and income level and polymerase chain reaction (PCR) test in the propensity score-matched cohorts (Tables S15 – S24 ).
Findings of this study
This is the first study to use population-based, binational large-scale cohort study databases from South Korean and Japanese nationwide cohorts that expresses the association of SARS-CoV-2 infection with acute respiratory complication or post-acute respiratory sequelae. First, the risk of acute respiratory complication or post-acute respiratory sequelae is significantly increased in participants with SARS-CoV-2 infection, compared to the general population. Second, SARS-CoV-2 infection induced a significantly increased risk for several specific post-acute respiratory sequelae, including chronic respiratory failure, COPD, emphysema, asthma, and interstitial lung disease, compared to the general population. In addition, several acute respiratory complications, including aspergillosis pneumonia, pneumothorax, acute respiratory failure, and pulmonary embolism, also depicted a notable increase in risk after SARS-CoV-2 infection compared to the general population. Third, people who were vaccinated, especially multiple vaccinations and mixed vaccinations, had a lower risk of developing post-acute respiratory sequelae than infected patients of SARS-CoV-2 without vaccination. Fourth, the risk of post-acute respiratory sequelae and acute respiratory complications increased with the severity of COVID-19. Fifth, infection of SARS-CoV-2 was associated with an increase of post-acute respiratory sequelae and acute respiratory complications regardless of the strain type. Lastly, the risk of post-acute respiratory sequelae diminished with time following SARS-CoV-2 infection yet persisted beyond 6 months post-infection.
Comparisons with previous studies
Some previous studies have examined the relationship between COVID-19 and respiratory complications. However, previous research encompassed countries such as Brazil ( n = 88) 10 , Palestine ( n = 705) 11 , and Netherlands ( n = 257) 12 , with small cohorts and without a general population group as controls or influenza cohort as an external comparator. In addition, previous studies did not consider the subtypes of specific respiratory diseases, vaccinations, and severity of COVID-19 on the association between respiratory outcomes and SARS-CoV-2 infections 13 . Therefore, our study is distinct from other studies in that we compared the association of COVID-19 and respiratory diseases with that of influenza by using population-based binational cohorts with a generalizable scale (main cohort, total N = 10,027,506; replication cohort, total N = 4,909,861).
Possible explanations
Influenza and COVID-19 have similar symptoms such as fever, cough, shortness of breath, and sore throat 14 . However, it is known that people who are infected with SARS-CoV-2 may have more severe symptoms and take longer to recover than those infected with influenza 15 . The longer time and severity of COVID-19 may have had a greater influence on the patients and the overall immune system 16 , 17 . Severe COVID-19 can lead to lasting changes in hematopoietic stem and progenitor cells and immune cell phenotypes in individuals while they are recovering from COVID-19 16 . Also, T cells can be impaired in severe disease and can be associated with intense activation and lymphopenia 18 . Therefore, SARS-CoV-2 infection can make the patients more vulnerable to developing other respiratory diseases. This aligns with our findings that indicate that infection of SARS-CoV-2 has a greater influence on developing acute respiratory complications or post-acute respiratory sequelae compared to influenza.
Unlike influenza, SARS-CoV-2 infection induces a fibrosis-associated transcriptional profile in pulmonary macrophages, characterized by elevated levels of transforming growth factor beta 1 and transforming growth factor beta induced, as well as other proteins like macrophage mannose receptor 1 and cluster of differentiation 163 19 , 20 . This gene expression pattern enhances the profibrotic functions of macrophages 20 , potentially leading to acute respiratory distress syndrome. Aspergillosis pneumonia, an infection caused by inhaling spores of the fungus Aspergillus, prevalent in the natural environment 21 , does not usually develop. However, there are many countries that use antibiotics for COVID-19 treatment 22 , 23 , 24 , and overuse of antibiotics can heighten the risk of aspergillosis pneumonia development since the antibiotics may cause a disturbance to the immune system 25 , 26 . This explains our finding that specific diseases of acute respiratory complication or post-acute respiratory sequelae depicted a notable increase of risk after SARS-CoV-2 infection compared to influenza virus infection.
Many studies have confirmed that vaccination significantly reduces the infection rate of SARS-CoV-2 and the severity of COVID-19 symptoms 27 , 28 . The efficacy of vaccination is more profound in preventing severe cases and deaths 29 . The reduced severity due to vaccination may positively affect immune resilience 30 , thereby decreasing the incidence of acute respiratory complication or post-acute respiratory sequelae. Given that the efficacy of the vaccination drops in the first 6 months, booster vaccination might be essential to sustain protective effects 29 . This is consistent with the result of this study in that multiple vaccinations decreases the development of acute respiratory complications or post-acute respiratory sequelae. Furthermore, many studies showed that mixing types of vaccination for COVID-19 may lower the risk of SARS-CoV-2 infection 31 . This approach could also mitigate the development of future respiratory complications.
Post-recovery from COVID-19, the immune system undergoes reconstruction 32 . However, the elevated interferon responsive genes in monocytes can still be found after 4 months since the infection 33 , which implies that the immune system is not fully recovered after 4 months, and constant attention must be paid to the patients. Our findings show that as time passes after initial infection with SARS-CoV-2, the risk of developing acute respiratory complications or post-acute respiratory sequelae gradually decreased. However, the risk for respiratory sequelae in post-acute COVID-19 condition persisted beyond 6 months post-infection.
Limitations and strengths
This is the first study to utilize binational, large-scale, population-based databases to examine risk for respiratory sequelae in acute or post-acute COVID-19 conditions in patients with SARS-CoV-2 infection. However, some limitations must be taken into consideration. First, although the database used is a highly credible database that covers 98% of the Korean population and 40% of the Japanese population, individuals who could be vulnerable to influenza and COVID-19, such as immigrants and undocumented immigrants, are left out of the database 34 , 35 , 36 . Likewise, the JMDC database does not include the entire Japanese population and may have potential bias. Second, our data is limited to the East Asian population, specifically South Korea and Japan. Therefore, our study is difficult to generalize to other ethnic groups. Third, the K-COV-N and JMDC datasets are heterogeneous. Therefore, we opted against merging the datasets, using the K-COV-N data for the main cohort and the JMDC data for the replication cohort. In addition, we used different lists of the covariates for each main and replication cohort due to the difference in data structure. Fourth, the dataset we utilized has a risk of underdiagnoses of SARS-CoV-2 and influenza infection. There is a possibility of overlooking patients who were infected with SARS-CoV-2 or influenza but did not take the PCR test or visit a hospital to receive treatment. However, to assess the potential underdiagnoses, we analyzed the HR of post-acute respiratory sequelae and short-term acute respiratory complications with participants after PCR tests. Fifth, the HR and its 95% CI for the risk of asthma may differ from previous research due to the difference in experimental designs, including study population or definition of exposure. Sixth, the propensity score-matched cohort had differential missingness between those infected with SARS-CoV-2 and the general population for national health examination information variables (BMI, blood pressure, fasting blood glucose, glomerular filtration rate, smoking status, alcohol consumption, and aerobic physical activity; >40% versus <1%), due to their exclusion from matching criteria.
Policy implications
This binational, large-scale, population-based cohort study further emphasizes risks in relation to SARS-CoV-2 infection, the importance of vaccination, efficient vaccination methods, and post-acute COVID-19 conditions with an emphasis on acute respiratory complications or post-acute respiratory sequelae. These findings depict a need for different health policies to manage social health. To minimize adverse respiratory outcomes after being infected with SARS-CoV-2, the government should make policies to mix and match the vaccine types to individuals. Individuals should be investigated even after full recovery from COVID-19 to resolve post-acute COVID-19 conditions.
In conclusion, this study emphasizes that the risk of developing acute respiratory complications or post-acute respiratory sequelae in post-COVID-19 condition is associated with infection of SARS-CoV-2, and the risk was more pronounced with increasing COVID-19 severity. People who were vaccinated had a lower risk of developing acute respiratory complications or post-acute respiratory sequelae than those without vaccination. While the risk of acute respiratory complications or post-acute respiratory sequelae decreases with time post-SARS-CoV-2 infection, it remains evident beyond 6 months. Therefore, our findings suggest that the potential risk of respiratory sequelae in acute or post-acute COVID-19 conditions accentuates the imperative for continued vigilance and response to SARS-CoV-2.
Data source
Utilizing large-scale, population-based binational cohorts, this study incorporated a South Korean nationwide claim-based cohort (K-COV-N cohort; total N = 10,027,506) for the main cohort and a Japanese claim-based cohort (JMDC cohort; total N = 4,909,861) for the replication cohort (Fig. 1 ) 37 . Both the K-COV-N and the JMDC cohorts were constructed through data derived from a universal health insurance claims system. This study received approvals from the Korea Disease Control and Prevention Agency (KDCA), National Health Insurance Service (NHIS; KDCA-NHIS-2022-1-632), JMDC (PHP-00002201-04), and the Institutional Review Board of Kyung Hee University (KHSIRB-23-241). Under the terms of the approval, patient consent was not required to use routine health records for our study.
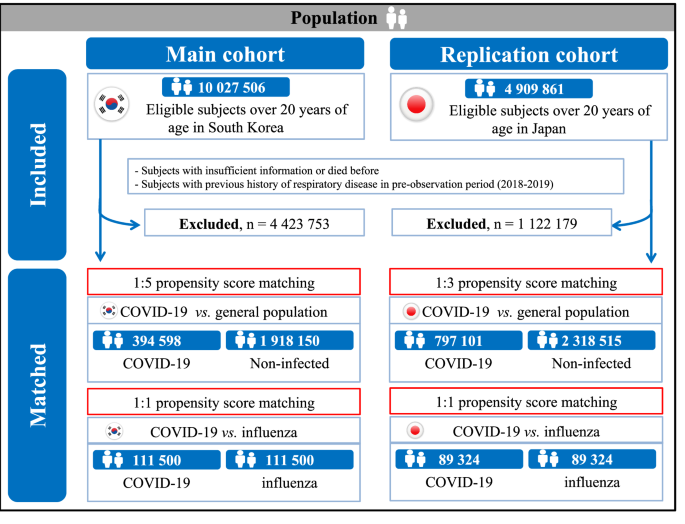
Study population in the main cohort (South Korea) and replication cohort (Japan).
K-COV-N cohort for main cohort
We utilized the NHIS database, which is a large-scale, nationwide, general, population-based cohort in South Korea, covering 98% of the population for the main cohort 34 . The NHIS and the KDCA provided data for the cohort constructed for study purposes, which includes participants ≥20 years old with a record of medical examination from January 1, 2018, to December 31, 2021 (total N = 10,027,506). The dataset consists of national health examination information, death records, health insurance data including individual demographic information, outpatient/inpatient records, and pharmaceutical data from the NHIS and COVID-19 vaccination data, SARS-CoV-2 test results, and COVID-19-related outcomes from the KDCA. The constructed K-COV-N database embodies the following characteristics, thereby affirming its significance: (1) the Korean government has established an extensive healthcare system to provide coverage for individuals infected with SARS-CoV-2; (2) all patient-related data was anonymized by the Korean government 34 , 36 ; and (3) according to the prior study, the diagnostic records from the NHIS had a predictive accuracy of 82% 38 .
The previous diagnostic history was assessed during the pre-observation period from 2018 to 2019, the follow-up observation period was between 2020 and 2021. The follow-up date ended on December 31, 2021, at death, or at development of primary outcomes (Fig. S1 ). We excluded participants with the following criteria: (1) insufficient demographic information and those who died before (excluded n = 3,967,482); and (2) previous history of chronic respiratory disease in the pre-observation period (excluded n = 710,468).
Exposure to SARS-CoV-2 is defined as an infection validated using a real-time reverse transcriptase polymerase chain reaction (RT-PCR) assay or antigen test on nasal and pharyngeal swabs, as approved by the KDCA 39 . Patients necessitating intensive care, oxygen therapy, extracorporeal membrane oxygenation, renal replacement, or cardio resuscitation were classified as having moderate to severe COVID-19 40 . All other cases were categorized as having mild COVID-19 41 . COVID-19 vaccination was classified according to the number of vaccinations (unvaccinated, 1, and ≥2 times) and vaccine type (unvaccinated, mRNA vaccinated [Pfizer-BioNTech and Moderna], viral vector vaccinated [Oxford-AstraZeneca and Johnson & Johnson/Janssen], and vaccinated with both types) 36 . Only vaccination status before SARS-CoV-2 infection was considered in our analysis. In South Korea, SARS-CoV-2 infection from January 2020 to July 31, 2021, was defined as the original stain, and the SARS-CoV-2 infection from August 1, 2021, to December 31, 2021, was defined as the delta 36 , 37 , 42 . In Japan, diagnosis of SARS-CoV-2 infection was categorized as infection with the original strain until May 31, 2021, and delta variants from June 1, 2021, to December 31, 2021 36 , 43 . To examine the relative severity of COVID-19 in comparison with another contagious viral respiratory disease, additional exposure to influenza infection was defined. It refers to cases diagnosed through an RT-PCR assay or antigen test on nasal and pharyngeal swabs during the observation period. For individuals infected with both SARS-CoV-2 and influenza, it includes instances of influenza infection developing after the SARS-CoV-2 infection.
To investigate the impact of SARS-CoV-2 infection on acute respiratory complication and post-acute respiratory sequelae, respectively, our experimental designs included two distinct ‘primary outcomes’. First, we used the incidence of various post-acute respiratory sequelae after 30 days of SARS-CoV-2 as the ‘primary outcome’ for respiratory sequelae in post-acute COVID-19 conditions. Second, the ‘primary outcome’ for acute respiratory complication is the incidence of various respiratory diseases within 1 month following a diagnosis of SARS-CoV-2 44 , 45 , 46 , 47 . Outcomes were defined based on appropriate International Classification of Diseases 10 th (ICD-10) codes for the new-onset of the specific diagnosis with at least one claim within one year. Post-acute respiratory sequelae were defined as chronic respiratory failure, pulmonary hypertension, sleep apnea, COPD, emphysema, asthma, pulmonary sarcoidosis, and interstitial lung disease 48 . In addition, acute respiratory complication was defined as pneumocystis pneumonia, aspergillosis pneumonia, pleural empyema, lung abscess, pneumothorax, acute respiratory failure, and pulmonary embolism (Table S25 ) 48 .
Participant demographic data was sourced from the insurance database, which included age (20–39, 40–59, and ≥60 years), sex (male and female), household income percentiles (low [0–39], middle [40–79], high [80–100]), and region of residence (urban and rural) 34 . CCI (0, 1, and ≥2), histories of cardiovascular disease and chronic kidney disease, and previous use of medication for diabetes, hyperlipidemia, and hypertension were identified using the ICD-10 codes, combined with results of general health examinations and personal medical interview 34 , 37 , 41 . From the health examination, BMI (underweight [<18.5 kg/m 2 ], normal [18.5–22.9 kg/m 2 ], overweight [23.0–24.9 kg/m 2 ], obese [≥25.0 kg/m 2 ], and unknown), blood pressure (systolic blood pressure < 140 mmHg and diastolic blood pressure < 90 mmHg, systolic blood pressure ≥ 140 mmHg or diastolic blood pressure ≥ 90 mmHg, and unknown), fasting blood glucose (<100, ≥100 mg/dL, and unknown), serum total cholesterol (<200, 200–239, ≥240 mg/dL, and unknown), glomerular filtration rate (<60, 60–89, ≥90 mL/min/1.73 m 2 , and unknown), smoking status (never, former, current smoker, and unknown), alcoholic drinks (<1, 1–2, 3–4, ≥5 days per week, and unknown), aerobic physical activity (sufficient [≥150 min/week of moderate-intensity activity or ≥75 min/week of vigorous-intensity activity or greater than an equivalent combination], insufficient, and unknown), and type of SARS-CoV-2 (original and delta) were obtained 39 .
Propensity score matching
To enhance the robustness and generalizability of our primary findings and balance baseline covariates, we employed exposure-driven propensity score matching. This approach compared individuals with SARS-CoV-2 infection to those without infection as a general population 49 . The propensity score was calculated by using a logistic regression model, adjusted for age (20–39, 40–59, and ≥60 years), sex (male and female), region of residence (urban and rural), history of cardiovascular and chronic disease, and medication use for diabetes, hyperlipidemia, and hypertension. Individuals were paired in a 1:5 ratio between the exposure group (SARS-CoV-2) and the non-exposure group. Through the prior procedures, we generated multi-to-one matched cohorts utilizing a ‘greedy nearest-neighbour’ algorithm, maintaining a caliper width of 0.001 standard deviations. The quality of the match was evaluated through the SMD, with an SMD <0.1, indicating minimal imbalances between the groups 49 . In addition, to investigate the relative severity of COVID-19 compared to other infectious viral respiratory diseases, an influenza group within the general population was utilized as another control group, directly matching SARS-CoV-2 infections at a 1:1 ratio.
Replication cohort in Japan
We employed the same definition of ICD-10 codes, exposure, and outcome assessments, general health examination, follow-up duration, and propensity score matching for the JMDC cohort (total N = 4,909,861) as we did with the main cohort. However, due to the lack of COVID-19 vaccination data in the JMDC cohort, we utilized this cohort primarily to validate the findings from the main cohort. Supplementary material was provided to address more detailed information about the validation cohort, which was caused by the different components and structures of the main cohort (Supplementary Material).
Statistical analysis
To estimate the HRs with 95% CIs, we applied the Cox proportional hazards model. Therefore, we assigned the ‘individual index date’ by following the criterion. For the exposed group, it is the date of the first diagnosis of SARS-CoV-2. For the individuals in the non-exposed group, the index date was allocated to match the index date of the corresponding exposed case. This approach was implemented to mitigate immortal time bias, ensuring an equitable comparison between groups.
In the matched COVID-19 cohort, we conducted various statistical analyses, detailed in Table S26 . These analyses involved respiratory disease (post-acute respiratory sequelae and acute respiratory complication) and its subtypes, number of vaccinations (without, 1 time, and ≥2 times), type of previous vaccination (mRNA, viral vector, and both) 36 , and strain of SARS-CoV-2 (original and delta). We further assessed the time attenuation effect of respiratory diseases after SARS-CoV-2 (<3, 3–6, and ≥6 months) to reduce reverse causation. To minimize the impact of potential confounders, the following variables were used for the adjusted model: age, sex, household income, region of residence, CCI score, obesity, blood pressure, fasting blood glucose, serum total cholesterol, glomerular filtration rate, smoking status, alcoholic drinks, aerobic physical activity, previous history of cardiovascular and chronic kidney diseases, history of medication use for diabetes mellitus, dyslipidemia, and hypertension, and strain of SARS-CoV-2 (original and delta). All statistical analyses were conducted using SAS (version 9.4; SAS Institute Inc., Cary, NC, USA) 50 , 51 . A two-sided P -value < 0.05 was considered statistically significant.
Sensitivity analysis
Several sensitivity analyses were conducted to enhance the credibility of the manuscript and our primary analyses. First, to identify detection bias and validate the results of our cohort, we performed a negative control analysis by exploring the association between tympanic membrane perforation disease and SARS-CoV-2 infection (Table S27 ). Second, we conducted an exposure-driven propensity score matching analysis based on the claim record of individuals who tested positive for SARS-CoV-2 within 2 weeks following an RT-PCR test and those who did not, for a more sophisticated analysis of the association between SARS-CoV-2 infection and respiratory symptoms (Table S15 ). Third, stratification analyses were further employed in two matched cohorts stratified by factors, including sex, age group, household income, CCI, BMI status, smoking status, alcoholic drinks, region of residence, and aerobic physical activity. Fourth, to thoroughly evaluate the marginal risks and prevalence of acute and post-acute respiratory conditions post-COVID-19, we utilized the average treatment effect in the overlap method, calculating overlap-weighted hazard ratios (Tables S7 – S9 ) 52 , 53 , 54 .
Patient and public involvement
The Korean government and JMDC anonymized patient data by excluding patient-related data such as personal identification numbers or names for confidentiality. While direct identification of individuals was rendered impossible due to the removal of names, all other pertinent data remained intact and accessible for our analyses. Research questions and outcome measures were autonomously determined without the intervention of individuals. The research design and implementation proceeded without external consultation. However, it can be extended to include contributions from other qualified public participants who are able to offer valuable insights into the research design, analysis, and interpretation. Upon request, the researchers intend to disseminate the results of this research to all research participants and relevant communities.
Reporting summary
Further information on research design is available in the Nature Portfolio Reporting Summary linked to this article.
Data availability
The datasets analyzed during the current study are available in the National Health Insurance Service in South Korea ( https://nhiss.nhis.or.kr/bd/ab/bdaba000eng.do ) and the JMDC in Japan ( https://www.jmdc.co.jp/en/jmdc-claims-database/ ). This protects the confidentiality of the data and ensures that Information Governance is robust. Applications to access health data in South Korea are submitted to the National Health Insurance Service in South Korea. Information can be found at https://nhiss.nhis.or.kr/bd/ab/bdaba000eng.do . Applications to access health data in Japan are submitted to the JMDC, Japan. Information can be found at https://www.jmdc.co.jp/en/jmdc-claims-database/ .
Code availability
This study did not generate new or customized code/algorithm. Statistical analyses were performed using SAS (version 9.4; SAS Institute Inc., Cary, NC, USA) for analysis of big data. The codes utilized in the analysis are available from the corresponding author.
Huang, C. et al. 6-month consequences of COVID-19 in patients discharged from hospital: a cohort study. Lancet (London, England) 397 , 220–232 (2021).
Article CAS PubMed Google Scholar
Kim, S. Y. & Yeniova, A. Ö. Global, regional, and national incidence and mortality of COVID-19 in 237 countries and territories, January 2022: a systematic analysis for world health organization COVID-19 dashboard. Life Cycle 2 , e10 (2022).
Article Google Scholar
Chen, C. et al. Global prevalence of post-coronavirus disease 2019 (COVID-19) condition or long COVID: a meta-analysis and systematic review. J. Infect. Dis. 226 , 1593–1607 (2022).
Subramanian, A. et al. Symptoms and risk factors for long COVID in non-hospitalized adults. Nat. Med. 28 , 1706–1714 (2022).
Article CAS PubMed PubMed Central Google Scholar
Hodgson, C. L. & Broadley, T. Long COVID-unravelling a complex condition. Lancet Respir. Med. 11 , 667–668 (2023).
Article PubMed Google Scholar
Al-Jahdhami, I., Al-Mawali, A. & Bennji, S. M. Respiratory complications after COVID-19. Oman Med. J. 37 , e343 (2022).
Prevalence and attributable health burden of chronic respiratory diseases, 1990-2017: a systematic analysis for the global burden of disease Study 2017. Lancet. Resp. Med. 8 , 585–596 (2020).
Bowe, B., Xie, Y. & Al-Aly, Z. Acute and postacute sequelae associated with SARS-CoV-2 reinfection. Nat. Med. 28 , 2398–2405 (2022).
Kalil, A. C. & Thomas, P. G. Influenza virus-related critical illness: pathophysiology and epidemiology. Crit. Care 23 , 258 (2019).
Article PubMed PubMed Central Google Scholar
Visconti, N. et al. Long-term respiratory outcomes after COVID-19: a Brazilian cohort study. Rev. Panam. Salud. Publica. 46 , e187 (2022).
Abu Hamdh, B. & Nazzal, Z. A prospective cohort study assessing the relationship between long-COVID symptom incidence in COVID-19 patients and COVID-19 vaccination. Sci. Rep. 13 , 4896 (2023).
Article ADS CAS PubMed PubMed Central Google Scholar
Krueger, T. et al. Pulmonary function three to five months after hospital discharge for COVID-19: a single centre cohort study. Sci. Rep. 13 , 681 (2023).
Guan, W. J. et al. Chronic respiratory diseases and the outcomes of COVID-19: a nationwide retrospective cohort study of 39,420 cases. J. Allergy Clin. Immunol. Pract. 9 , 2645–2655.e2614 (2021).
Li, P., Wang, Y., Peppelenbosch, M. P., Ma, Z. & Pan, Q. Systematically comparing COVID-19 with the 2009 influenza pandemic for hospitalized patients. Int. J. Infect. Dis. 102 , 375–380 (2021).
Atamna, A. et al. Clinical outcomes of hospitalized patients with SARS-CoV-2 omicron variant vs. influenza a during influenza season 2021 to 2022: a retrospective observational study. Isr. Med. Assoc. J. IMAJ 25 , 585–589 (2023).
PubMed Google Scholar
Cheong, J. G. et al. Epigenetic memory of coronavirus infection in innate immune cells and their progenitors. Cell 186 , 3882–3902.e3824 (2023).
Gao, F. et al. Spheromers reveal robust T cell responses to the Pfizer/BioNTech vaccine and attenuated peripheral CD8(+) T cell responses post SARS-CoV-2 infection. Immunity 56 , 864–878.e864 (2023).
Moss, P. The T cell immune response against SARS-CoV-2. Nat. Immunol. 23 , 186–193 (2022).
Mehta, P., Rosas, I. O. & Singer, M. Understanding post-COVID-19 interstitial lung disease (ILD): a new fibroinflammatory disease entity. Intens. Care Med. 48 , 1803–1806 (2022).
Wendisch, D. et al. SARS-CoV-2 infection triggers profibrotic macrophage responses and lung fibrosis. Cell 184 , 6243–6261.e6227 (2021).
Mousavi, B., Hedayati, M. T., Hedayati, N., Ilkit, M. & Syedmousavi, S. Aspergillus species in indoor environments and their possible occupational and public health hazards. Curr. Med. Mycol. 2 , 36–42 (2016).
Hossain, M. J. et al. Irrational use of antibiotics and factors associated with antibiotic resistance: findings from a cross-sectional study in Bangladesh. Health Sci. Rep. 6 , e1465 (2023).
Thapa, B., Pathak, S. B., Jha, N., Sijapati, M. J. & Shankar, P. R. Antibiotics use in hospitalised COVID-19 patients in a tertiary care centre: a descriptive cross-sectional study. JNMA . J. Nepal Med. Assoc. 60 , 625–630 (2022).
Popp, M. et al. Antibiotics for the treatment of COVID-19. Cochrane Database Syst. Rev. 10 , Cd015025 (2021).
Seelbinder, B. et al. Antibiotics create a shift from mutualism to competition in human gut communities with a longer-lasting impact on fungi than bacteria. Microbiome 8 , 133 (2020).
Scott, N. A. et al. Antibiotics induce sustained dysregulation of intestinal T cell immunity by perturbing macrophage homeostasis. Sci. Transl. Med. 10 , eaao 4755 (2018).
Feikin, D. R. et al. Duration of effectiveness of vaccines against SARS-CoV-2 infection and COVID-19 disease: results of a systematic review and meta-regression. Lancet (London, England) 399 , 924–944 (2022).
Wu, N. et al. Long-term effectiveness of COVID-19 vaccines against infections, hospitalisations, and mortality in adults: findings from a rapid living systematic evidence synthesis and meta-analysis up to december, 2022. Lancet Resp. Med. 11 , 439–452 (2023).
Yang, Z. R. et al. Efficacy of SARS-CoV-2 vaccines and the dose-response relationship with three major antibodies: a systematic review and meta-analysis of randomised controlled trials. Lancet Microbe. 4 , e236–e246 (2023).
Hermens, J. M. & Kesmir, C. Role of T cells in severe COVID-19 disease, protection, and long term immunity. Immunogenetics 75 , 295–307 (2023).
Chen, Y. C. et al. Risk reduction analysis of mix-and-match vaccination strategy in healthcare workers during SARS-CoV-2 omicron variant predominant period: a multi-center cohort study in Taiwan. Hum. Vaccines Immunother. 19 , 2237387 (2023).
Tiyo, B. T. et al. What happens to the immune system after vaccination or recovery from COVID-19? Life (Basel, Switzerland). 11 , 1152 (2021).
Hoffmann, A. D. et al. Unique molecular signatures sustained in circulating monocytes and regulatory T cells in convalescent COVID-19 patients. Clin. Immunol. 252 , 109634 (2023).
Shin, Y. H. et al. Autoimmune inflammatory rheumatic diseases and COVID-19 outcomes in South Korea: a nationwide cohort study. Lancet Rheumatol. 3 , e698–e706 (2021).
Sato, M. et al. Effect of periodontal therapy on glycaemic control in type 2 diabetes. J. Clin. Periodontol . 51 , 380–389 (2024).
Oh, J. et al. Incident allergic diseases in post-COVID-19 condition: multinational cohort studies from South Korea, Japan and the UK. Nat. Commun. 15 , 2830 (2024).
Kim, M. S. et al. Long-term autoimmune inflammatory rheumatic outcomes of COVID-19: a binational cohort study. Ann. Intern. Med . 117 , 3 (2024).
Park, B. J., et al. Report of the evaluation for validity of discharged diagnoses in Korean Health Insurance database. Seoul National University 2003 , 19–52 (2003).
Lee, S. W. et al. Physical activity and the risk of SARS-CoV-2 infection, severe COVID-19 illness and COVID-19 related mortality in South Korea: a nationwide cohort study. Br. J. Sports Med. 56 , 901–912 (2022).
Lee, S. W. et al. Association between mental illness and COVID-19 in South Korea: a post-hoc analysis. Lancet Psychiatry 8 , 271–272 (2021).
Lee, S. W. et al. Association between mental illness and COVID-19 susceptibility and clinical outcomes in South Korea: a nationwide cohort study. Lancet. Psychiatry 7 , 1025–1031 (2020).
Ryu, B. H. et al. Clinical features of adult COVID-19 patients without risk factors before and after the nationwide SARS-CoV-2 B.1.617.2 (Delta)-variant outbreak in Korea: experience from gyeongsangnam-do. J. Korean Med. Sci. 36 , e341 (2021).
Ito, K. et al. Predicted dominance of variant delta of SARS-CoV-2 before Tokyo Olympic games, Japan. Euro Surveill. 26 , 2100570 (2021).
Vilar, F., Khoo, S. & Walley, T. The management of pneumocystis carinii pneumonia. Br. J. Clin. Pharmacol. 47 , 605 (1999).
Ampofo, K. & Byington, C. Management of parapneumonic empyema. Pediatric Infect. Dis. J. 26 , 445–446 (2007).
Toufen, C. Jr. et al. Follow-up after acute respiratory distress syndrome caused by influenza a (H1N1) virus infection. Clinics (Sao Paulo, Brazil) 66 , 933–937 (2011).
Jin, J. JAMA PATIENT PAGE. Treatment duration for pulmonary embolism. Jama 314 , 98 (2015).
Beltramo, G. et al. Chronic respiratory diseases are predictors of severe outcome in COVID-19 hospitalised patients: a nationwide study. Eur. Respir. J. 58 , 2004474 (2021).
Lee, S. W. & Acharya, K. P. Propensity score matching for causal inference and reducing the confounding effects: statistical standard and guideline of. Life Cycle 2 , e18 (2022).
Lee, S. W. Regression analysis for continuous independent variables in medical research: statistical standard and guideline of. Life Cycle 2 , e3 (2022).
Lee, J. S. et al. Breastfeeding and impact on childhood hospital admissions: a nationwide birth cohort in South Korea. Nat. Commun. 14 , 5819 (2023).
Thomas, L. E., Li, F. & Pencina, M. J. Overlap weighting: a propensity score method that mimics attributes of a randomized clinical trial. JAMA 323 , 2417–2418 (2020).
Li, Z., Yan, M. & Liu, Y. Prevalence of body mass index categories among adults living alone in China: observational study. PLoS One 19 , e0297096 (2024).
Dumont, R. et al. A population-based serological study of post-COVID syndrome prevalence and risk factors in children and adolescents. Nat. Commun. 13 , 7086 (2022).
Article ADS PubMed PubMed Central Google Scholar
Download references
Acknowledgements
This study used the database of the Korea Disease Control and Prevention Agency (KDCA) and the National Health Insurance Service (NHIS) for policy and academic research. The research number of this study is KDCA-NHIS-2022-1-632. This research was supported by grants from National Research Foundation of Korea (NRF) funded by the Korea government (MSIT; RS-2023-00248157; D.K.Y.), Ministry of Food and Drug Safety in 2024 (21153MFDS601; D.K.Y.), and the Korea Health Technology R&D Project through the Korea Health Industry Development Institute (KHIDI), funded by the Ministry of Health & Welfare, Republic of Korea (HV22C0233; D.K.Y.) and Cosmax BTI. We also thank Dong-Geol Lee (Cosmax BTI) and Seunghyun Kang (Cosmax BTI) for helpful advice and comments. The funders had no role in study design, data collection, data analysis, data interpretation, or writing of the report.
Author information
These authors contributed equally: Yujin Choi, Hyeon Jin Kim, Jaeyu Park.
These authors jointly supervised this work: Hayeon Lee, Jiseung Kang, Dong Keon Yon.
Authors and Affiliations
Center for Digital Health, Medical Science research Institute, Kyung Hee University College of Medicine, Seoul, South Korea
Yujin Choi, Hyeon Jin Kim, Jaeyu Park, Myeongcheol Lee, Hayeon Lee & Dong Keon Yon
Department of Korean Medicine, Kyung Hee University College of Korean Medicine, Seoul, South Korea
Department of Regulatory Science, Kyung Hee University, Seoul, South Korea
Hyeon Jin Kim, Jaeyu Park, Myeongcheol Lee & Dong Keon Yon
Department of Family Medicine, Kyung Hee University Medical Center, Kyung Hee University College of Medicine, Seoul, South Korea
Sunyoung Kim
Research and Development Unit, Parc Sanitari Sant Joan de Deu, Barcelona, Spain
Ai Koyanagi
Centre for Health, Performance and Wellbeing, Anglia Ruskin University, Cambridge, UK
Cardiovascular Disease Initiative, Broad Institute of MIT and Harvard, Cambridge, MA, USA
Min Seo Kim
CEReSS Health Service Research and Quality of Life Center, Assistance Publique-Hôpitaux de Marseille, Aix-Marseille University, Marseille, France
Masoud Rahmati
Department of Physical Education and Sport Sciences, Faculty of Literature and Humanities, Vali-E-Asr University of Rafsanjan, Rafsanjan, Iran
Department of Physical Education and Sport Sciences, Faculty of Literature and Human Sciences, Lorestan University, Khoramabad, Iran
Division of Sleep Medicine, Harvard Medical School, Boston, MA, USA
Jiseung Kang
Department of Anesthesia, Critical Care and Pain Medicine, Massachusetts General Hospital, Boston, MA, USA
Department of Pediatrics, Kyung Hee University Medical Center, Kyung Hee University College of Medicine, Seoul, South Korea
Dong Keon Yon
You can also search for this author in PubMed Google Scholar
Contributions
D.K.Y. had full access to all of the data in the study and took responsibility for the integrity of the data and the accuracy of the data analysis. Y.C., H.J.K., J.P., M.L., S.K., A.K., L.S., M.S.K., M.R., H.L., J.K., and D.K.Y. approved the final version before submission. Study concept and design: Y.C., H.J.K., J.P., H.L., J.K., and D.K.Y.; Acquisition, analysis, or interpretation of data: Y.C., H.J.K., J.P., H.L., J.K., and D.K.Y.; Drafting of the manuscript: Y.C., H.J.K., J.P., H.L., J.K., and D.K.Y.; Critical revision of the manuscript for important intellectual content: Y.C., H.J.K., J.P., M.L., S.K., A.K., L.S., M.S.K., M.R., H.L., J.K., and D.K.Y.; Statistical analysis: Y.C., H.J.K., J.P., H.L., J.K., and D.K.Y.; Study supervision: D.K.Y. D.K.Y. is a guarantor for this study. The corresponding author attests that all listed authors meet authorship criteria and that no others meeting the criteria have been omitted.
Corresponding authors
Correspondence to Hayeon Lee , Jiseung Kang or Dong Keon Yon .
Ethics declarations
Competing interests.
The authors declare no competing interests.
Peer review
Peer review information.
Nature Communications thanks Jing Sun and the other, anonymous, reviewer(s) for their contribution to the peer review of this work. A peer review file is available.
Additional information
Publisher’s note Springer Nature remains neutral with regard to jurisdictional claims in published maps and institutional affiliations.
Supplementary information
Supplementary information, peer review file, reporting summary, rights and permissions.
Open Access This article is licensed under a Creative Commons Attribution 4.0 International License, which permits use, sharing, adaptation, distribution and reproduction in any medium or format, as long as you give appropriate credit to the original author(s) and the source, provide a link to the Creative Commons licence, and indicate if changes were made. The images or other third party material in this article are included in the article’s Creative Commons licence, unless indicated otherwise in a credit line to the material. If material is not included in the article’s Creative Commons licence and your intended use is not permitted by statutory regulation or exceeds the permitted use, you will need to obtain permission directly from the copyright holder. To view a copy of this licence, visit http://creativecommons.org/licenses/by/4.0/ .
Reprints and permissions
About this article
Cite this article.
Choi, Y., Kim, H.J., Park, J. et al. Acute and post-acute respiratory complications of SARS-CoV-2 infection: population-based cohort study in South Korea and Japan. Nat Commun 15 , 4499 (2024). https://doi.org/10.1038/s41467-024-48825-w
Download citation
Received : 14 November 2023
Accepted : 13 May 2024
Published : 27 May 2024
DOI : https://doi.org/10.1038/s41467-024-48825-w
Share this article
Anyone you share the following link with will be able to read this content:
Sorry, a shareable link is not currently available for this article.
Provided by the Springer Nature SharedIt content-sharing initiative
By submitting a comment you agree to abide by our Terms and Community Guidelines . If you find something abusive or that does not comply with our terms or guidelines please flag it as inappropriate.
Quick links
- Explore articles by subject
- Guide to authors
- Editorial policies
Sign up for the Nature Briefing newsletter — what matters in science, free to your inbox daily.

ORIGINAL RESEARCH article
The effectiveness of booster vaccination of inactivated covid-19 vaccines against susceptibility, infectiousness, and transmission of omicron ba.2 variant: a retrospective cohort study in shenzhen, china.

- 1 Office of Emergency, Shenzhen Center for Disease Control and Prevention, Shenzhen, China
- 2 Department of Biochemistry, Changzhi Medical College, Changzhi, China
- 3 Department of Infectious Disease, Heping Hospital Affiliated to Changzhi Medical College, Changzhi, China
- 4 Class of 2002 of the Department of Preventive Medicine, Changzhi Medical College, Changzhi, China
- 5 Institute of Evidence-Based Medicine, Heping Hospital Affiliated to Changzhi Medical College, Changzhi, China
Little studies evaluated the effectiveness of booster vaccination of inactivated COVID-19 vaccines against being infected (susceptibility), infecting others (infectiousness), and spreading the disease from one to another (transmission). Therefore, we conducted a retrospective cohort study to evaluate the effectiveness of booster vaccination of inactivated COVID-19 vaccines against susceptibility, infectiousness, and transmission in Shenzhen during an Omicron BA.2 outbreak period from 1 February to 21 April 2022. The eligible individuals were classified as four sub-cohorts according to the inactivated COVID-19 vaccination status of both the close contacts and their index cases: group 2-2, fully vaccinated close contacts seeded by fully vaccinated index cases (reference group); group 2-3, booster-vaccinated close contacts seeded by fully vaccinated index cases; group 3-2, fully vaccinated close contacts seeded by booster-vaccinated index cases; and group 3-3, booster-vaccinated close contacts seeded by booster-vaccinated index cases. Univariate and multivariate logistic regression analyses were applied to estimate the effectiveness of booster vaccination. The sample sizes of groups 2-2, 2-3, 3-2, and 3-3 were 846, 1,115, 1,210, and 2,417, respectively. We found that booster vaccination had an effectiveness against infectiousness of 44.9% (95% CI: 19.7%, 62.2%) for the adults ≥ 18 years, 62.2% (95% CI: 32.0%, 78.9%) for the female close contacts, and 60.8% (95% CI: 38.5%, 75.1%) for the non-household close contacts. Moreover, booster vaccination had an effectiveness against transmission of 29.0% (95% CI: 3.2%, 47.9%) for the adults ≥ 18 years, 38.9% (95% CI: 3.3%, 61.3%) for the female close contacts, and 45.8% (95% CI: 22.1%, 62.3%) for the non-household close contacts. However, booster vaccination against susceptibility did not provide any protective effect. In summary, this study confirm that booster vaccination of the inactivated COVID-19 vaccines provides low level of protection and moderate level of protection against Omicron BA.2 transmission and infectiousness, respectively. However, booster vaccination does not provide any protection against Omicron BA.2 susceptibility.
Introduction
In December 2019, an emerging infectious disease named coronavirus disease 2019 (COVID-19) caused by severe acute respiratory syndrome (SARS-CoV-2) virus was first found in Wuhan, China, and then, this virus is widespread all over the world and it has existed for nearly four years ( 1 – 3 ). Moreover, SARS-CoV-2 is constantly undergoing various variants, which have been categorized as variants of concern, variants of interest, and variants under monitoring by World Health Organization (WHO) ( 4 ). Variants of concern mainly involved Alpha, Beta, Gamma, Delta, and Omicron ( 4 ). The Omicron (B.1.1.529) variant was first identified in South Africa on November 24, 2021 and it quickly removed of all other strains and became the dominant strain worldwide because it had stronger transmissibility and immune escape ability ( 5 – 8 ).
COVID-19 vaccination is still regarded as one of the most effective methods for achieving herd immunity to control the disease and as an indispensable part of the long-term management of SARS-CoV-2 pandemic ( 9 – 12 ). COVID-19 vaccines mainly included inactivated virus vaccines (CoronaVac, WIV04, HB02, and BBV152), protein subunit vaccines (NVX-CoV2373 and SCB-2019), RNA-based vaccines (BNT162b2 and mRNA-1273), and Viral vector (non-replicating) vaccines (AZD1222 [ChAdOx1 nCoV-19], Sputnik V, Ad26.COV2.S, and Ad5-nCoV) ( 13 ). Large-scale phase III randomized clinical trials (RCTs) indicated that the full vaccination of the above COVID-19 vaccines had good protective effect against symptomatic COVID-19, especially severe COVID-19, COVID-19-related hospitalization, and COVID-19-related death ( 14 – 23 ). However, the results of RCTs concerning the COVID-19 vaccines efficacy were reported before the pandemic of the Omicron variant. It is an essential complement applying real-world data to evaluate the COVID-19 vaccines effectiveness (VE) because real-world studies have a larger and wider range of population and consider various background factors beyond the experimental conditions ( 24 – 28 ).
Although primary series of COVID-19 vaccines had adequate protective effect against symptomatic COVID-19, especially severe COVID-19 ( 13 ). However, the VE was rapidly waning over time ( 29 ). Therefore, many countries launched a campaign of booster vaccination by applying either homologous or heterologous COVID-19 vaccines. Booster vaccination was usually administered as a third dose when at least five-six months after full vaccination of COVID-19 vaccines. Currently, most real-world studies mainly assessed the effectiveness of COVID-19 booster vaccination of two mRNA vaccines against different outcomes of Omicron infection ( 13 ). In mainland China, since early 2021, two inactivated COVID-19 vaccines (HB02 [Sinopharm] and CoronaVac [Sinovac]) were most frequently used. Although several studies have assessed the effectiveness of booster vaccination of inactivated COVID-19 vaccines made in China against different outcomes of Omicron BA.2 infection ( 30 – 35 ). However, their studies ( 30 – 35 ) have only focused on the VE in preventing being infected or severe COVID-19. To our knowledge, evaluating the effectiveness of a vaccine includes both measure indicators: preventing infection and preventing infecting others. VE in preventing infection is the most common and basic indicator. In this study, this measure indicator is specifically defined as VE against susceptibility, which refers to the extent to which the vaccine decreasing secondary infection. It is a new concept on the VE in preventing infecting others, which has only attracted attention in recent years ( 36 – 38 ). VE against infectiousness was also named as VE against infectivity in some studies and may be interpreted as the reduction in transmission risk from a primary to secondary infection, which refers to the extent to which the vaccine reduces infectivity among individuals already infected. Moreover, vaccination can prevent transmission by providing protection against infection and simultaneously decreasing the infectivity of vaccinated individuals who become infected. Therefore, VE against transmission is defined as the combination of VE against susceptibility and VE against infectiousness in this study, which refers to the vaccine’s ability to break the chain of virus transmission and interrupt community spread.
We conducted a retrospective cohort study to assess the effectiveness of booster vaccination of inactivated COVID-19 vaccines against be infected (susceptibility), infecting others (infectiousness), and disease transmission among individuals (transmission) in Shenzhen during an Omicron BA.2 outbreak period from 1 February to 21 April 2022. Of note, the inactivated COVID-19 vaccines used were the initial SARS-CoV-2 strain vaccines in this study.
Study setting
This article was reported applying the S1 STROBE Checklist. With an Omicron BA.2 sub-lineage outbreak background in Shenzhen, Guangdong, China, reported the first COVID-19 case on February 1, 2022 and reached a peak of infections on March 15, 2022. On the basis of the “zero COVID-19” policy, the local government quickly took a series of control measures mainly including massive nucleic acid testing, contact tracing, isolation of COVID-19 cases, and quarantine of close contacts. Therefore, this major epidemic was controlled on April 21, 2022.
Study design and participants
This retrospective cohort study was conducted to analyze the close contacts of all confirmed COVID-19 cases by reverse transcription polymerase chain reaction (RT-PCR) tests in Shenzhen, China from February 1 to April 21, 2022. Close contacts referred to the individuals who were in the same exposure settings within close proximity without any effective protection as COVID-19 cases or SARS-CoV-2 positive asymptomatic infections two days before. In this study, individuals at risk of exposure were regarded as close contacts of confirmed cases. Contact tracing measures were conducted by Shenzhen Center for Disease Control and Prevention allows for matching the close contacts with their index cases. All the close contacts were traced, compulsorily quarantined, and tested every 2-3 days by RT-PCR tests for SARS-CoV-2 to monitor whether they were infected with SARS-CoV-2. All the secondary SARS-CoV-2 infections were the close contacts of their index cases before they became infected. Notably, all the secondary SARS-CoV-2 infections and uninfected close contacts made up a cohort together. For individuals with BA.2 sub-lineage infection, we extracted data, which mainly included age, sex, history of exposure, contact setting (i.e., household and non- household settings), onset date of clinical symptoms, first positive test date, and history of COVID-19 vaccination. Those close contacts who eventually tested positive for COVID-19 were treated as infected individuals (infectees) and their index cases (who were originally confirmed to have COVID-19) as infectors. Of note, close contacts became index cases when testing positive, so this study may include some individuals both as close contacts and as index cases. Furthermore, the matching involved creating multiple pairs of index-exposed individuals in this study. Overall, 8466 close contacts were matched with 644 index cases in the present study. Then, close contacts were excluded if both the close contacts and their index cases received non-inactivated COVID-19 vaccines or aged 0-17 years. Moreover, close contacts were excluded if both the close contacts and their index cases were unvaccinated, received partial vaccination, and those who were infected or exposed within 14 days of their last vaccination. The eligible individuals were classified as four sub-cohorts according to the inactivated COVID-19 vaccination status of both the close contacts and their index cases: fully vaccinated close contacts seeded by fully vaccinated index cases (group 2-2 [reference group]), booster-vaccinated close contacts seeded by fully vaccinated index cases (group 2-3), fully vaccinated close contacts seeded by booster-vaccinated index cases (group 3-2), and booster-vaccinated close contacts seeded by booster-vaccinated index cases (group 3-3).
Vaccination status and outcome
In this study, full vaccination and booster vaccination were defined as ≥ 14 days after second-dose and third-dose vaccination of inactivated COVID-19 vaccines, respectively. The primary outcome was Omicron BA.2 infection within close contacts.
Statistical analyses
Baseline characteristics were analyzed using the number (%) for categorical data. Differences in proportions were analyzed with the chi-squared test. The secondary attack rate was calculated by dividing the number of individuals with secondary infections by the overall number of close contacts related to index cases.
In this study, we applied the odds ratio (OR) to evaluate the association between the inactivated COVID-19 vaccination status (i.e., four sub-cohorts) and the risk of Omicron BA.2 secondary infection. The crude OR was calculated by applying a univariate logistic regression analysis, and the adjusted OR (aOR) was calculated using a multivariable ordinary logistic regression analysis by adjusting for potential confounding variables, including sex and age of close contacts and their index cases, contact settings, and exposure to index cases before or after symptom onset of the indexes. The crude or adjusted VE (aVE) was calculated as (1−OR) × 100%, and the OR is the odds ratio for the rate of secondary infection. In this study, the VE of booster vaccination of inactivated COVID-19 vaccines against susceptibility, infectiousness, and transmission were calculated through group 2-3 versus group 2-2, group 3-2 versus group 2-2, and group 3-3 versus group 2-2, respectively. Moreover, subgroup analyses were conducted according to sex and contact setting. All statistical analyses were conducted applying IBM SPSS Statistics 25.0.
General information of the close contacts
8,466 close contacts associated with index cases were identified from February 1 to April 21, 2022. Among them, 1,052 individuals were excluded because both these close contacts and their index cases received non-inactivated COVID-19 vaccines or aged 0-17 years. Moreover, we excluded 1,826 individuals who were unvaccinated, received partial vaccination, or were infected or exposed within 14 days of last dose. Therefore, 5,588 close contacts were included to analyze the effectiveness of booster vaccination of inactivated COVID-19 vaccines against susceptibility, infectiousness, and transmission ( Figure 1 ).
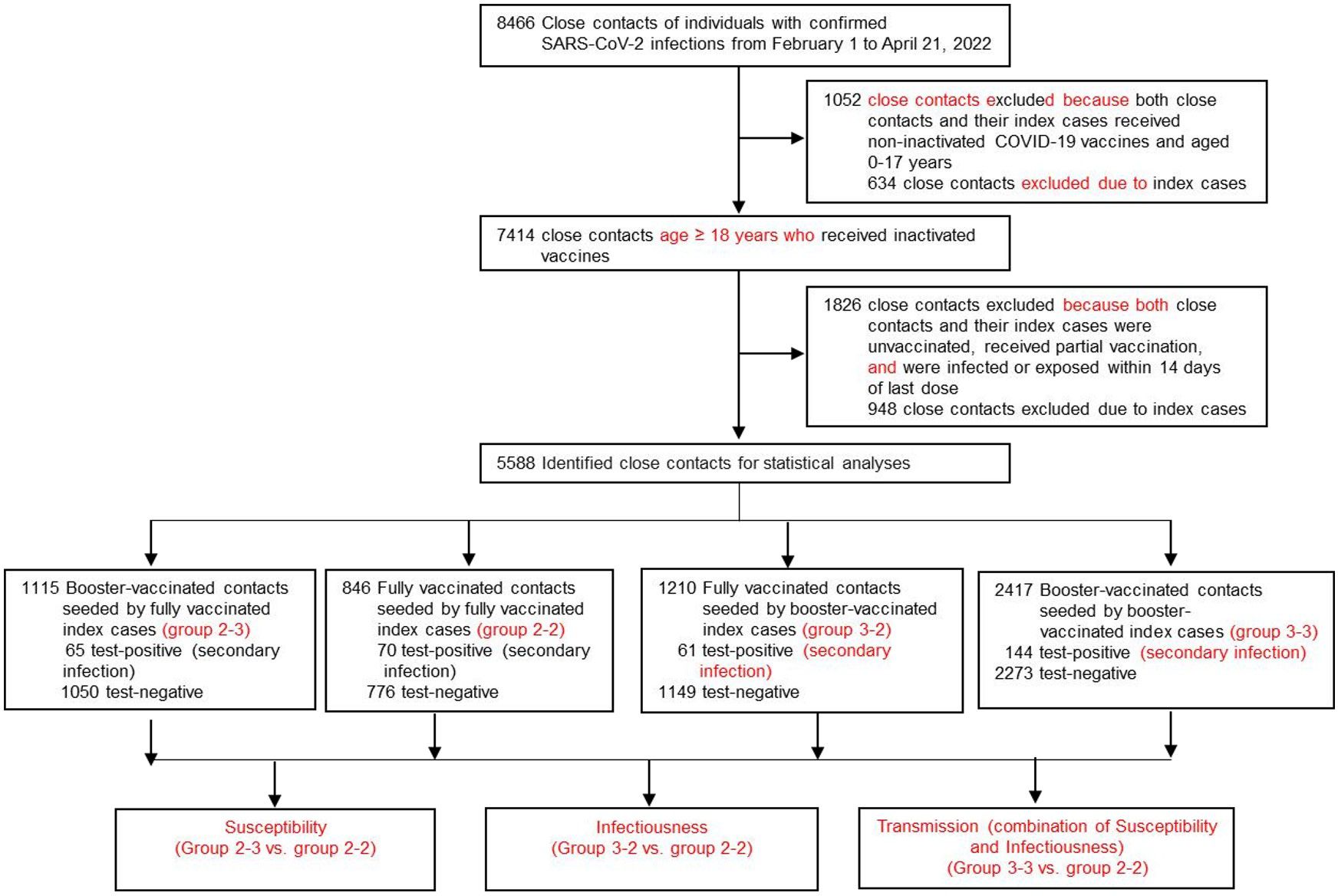
Figure 1 Flow chart of sample selection and grouping.
According to the inactivated COVID-19 vaccination status of both the close contacts and their index cases, the close contacts cohort was classified as four subgroups: group 2-2, group 2-3, group 3-2, and group 3-3, as stated earlier. The sample sizes of groups 2-2, 2-3, 3-2, and 3-3 were 846, 1,115, 1,210, and 2,417, respectively ( Figure 1 , Table 1 ), respectively. Among the 5,588 close contacts, 3,472 (62.1%) were male and 5,372 (96.1%) individuals aged 18-59 years old ( Table 1 ). Moreover, for index cases, 3,481 (62.3%) were male and 5,434 (97.2%) individuals aged 18-59 years old ( Table 1 ). In terms of contact setting, there were 888 (15.9%) and 4700 (84.1%) household and non-household settings ( Table 1 ). Moreover, there were 2,605 (46.6%) and 2,983 (53.1%) individuals with exposure to index cases after or before onset of indexes, respectively, ( Table 1 ). Furthermore, there were significantly different ( P < 0.001) for age, age and gender of index cases, and contact settings among the four sub-cohorts ( Table 1 ).
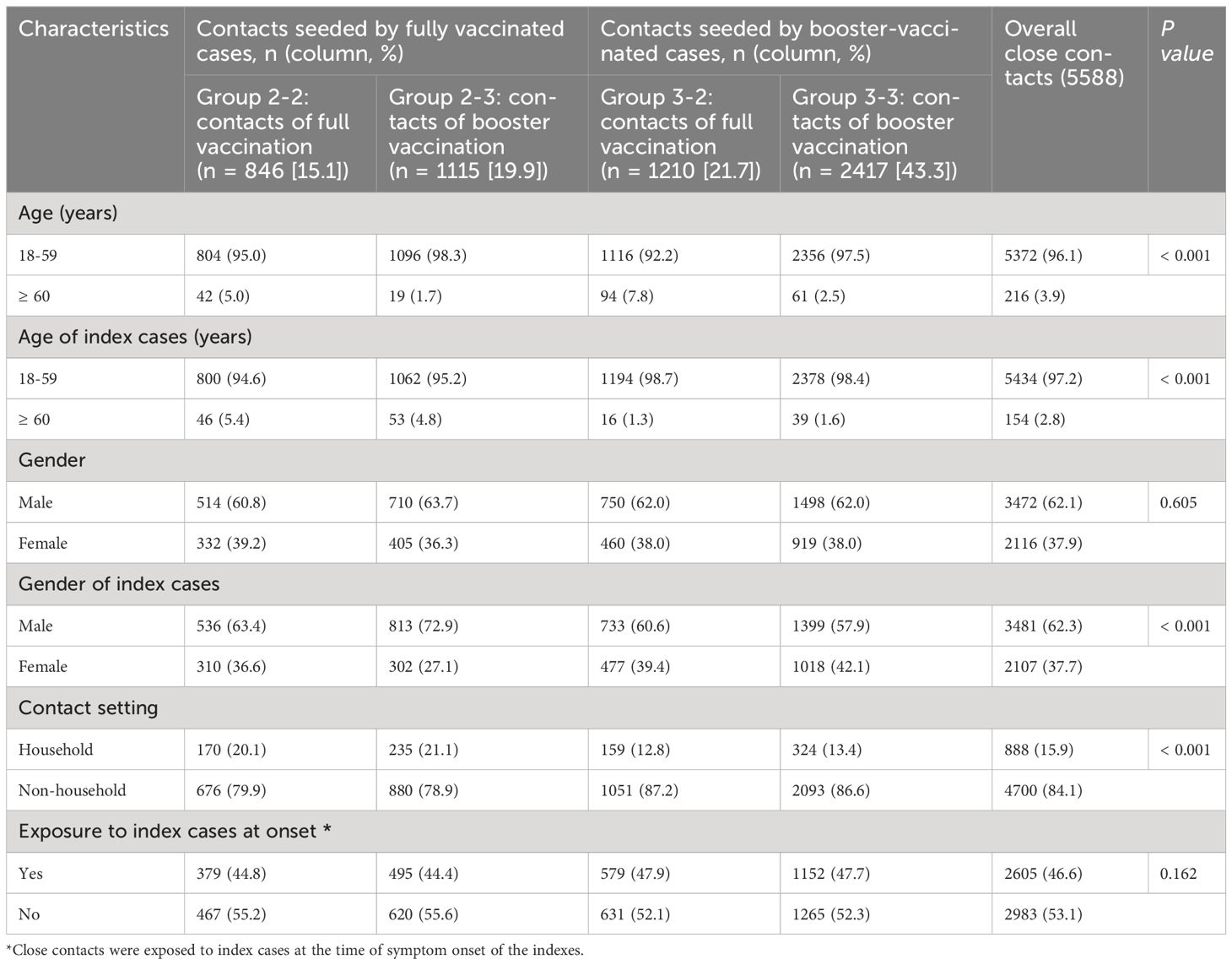
Table 1 Baseline characteristics of close contacts of Omicron BA.2 infections who received full vaccination and booster vaccination.
Effectiveness of booster vaccination of inactivated COVID-19 vaccines against susceptibility, infectiousness, and transmission
The overall effectiveness of booster vaccination of inactivated COVID-19 vaccines against susceptibility of Omicron BA.2 variant was 30.0% (95% CI: −1.9%, 51.9%, Table 2 , Figure 2 ) and did not provide any protective effect. Moreover, we found that booster vaccination of inactivated COVID-19 vaccines against susceptibility also did not provide any protective effect in the subgroups according to gender and contact setting ( Table 2 , Figure 2 ).
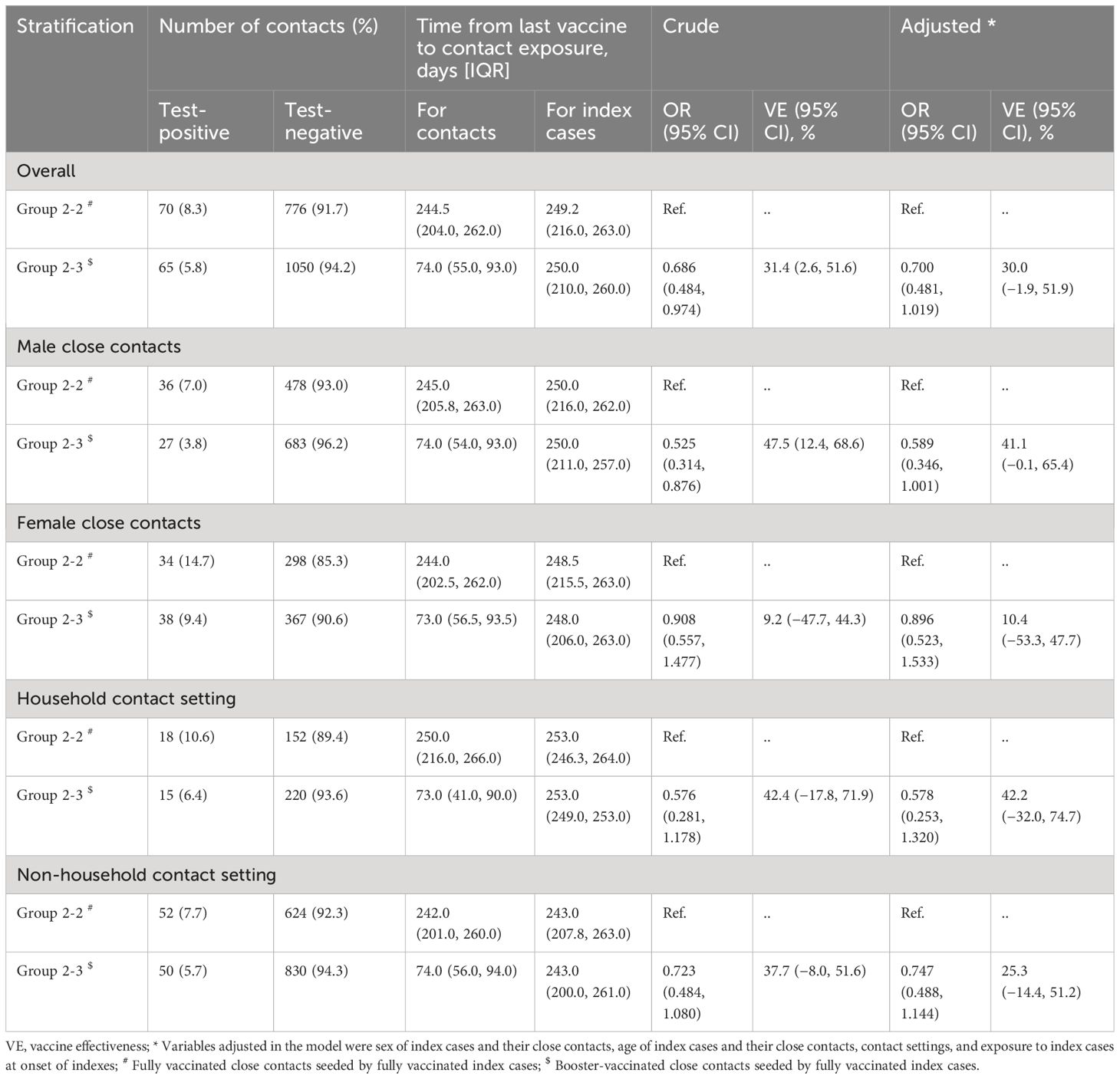
Table 2 The effectiveness of booster Vaccination of inactivated COVID-19 vaccines against susceptibility of Omicron BA.2 variant, in Shenzhen, China.
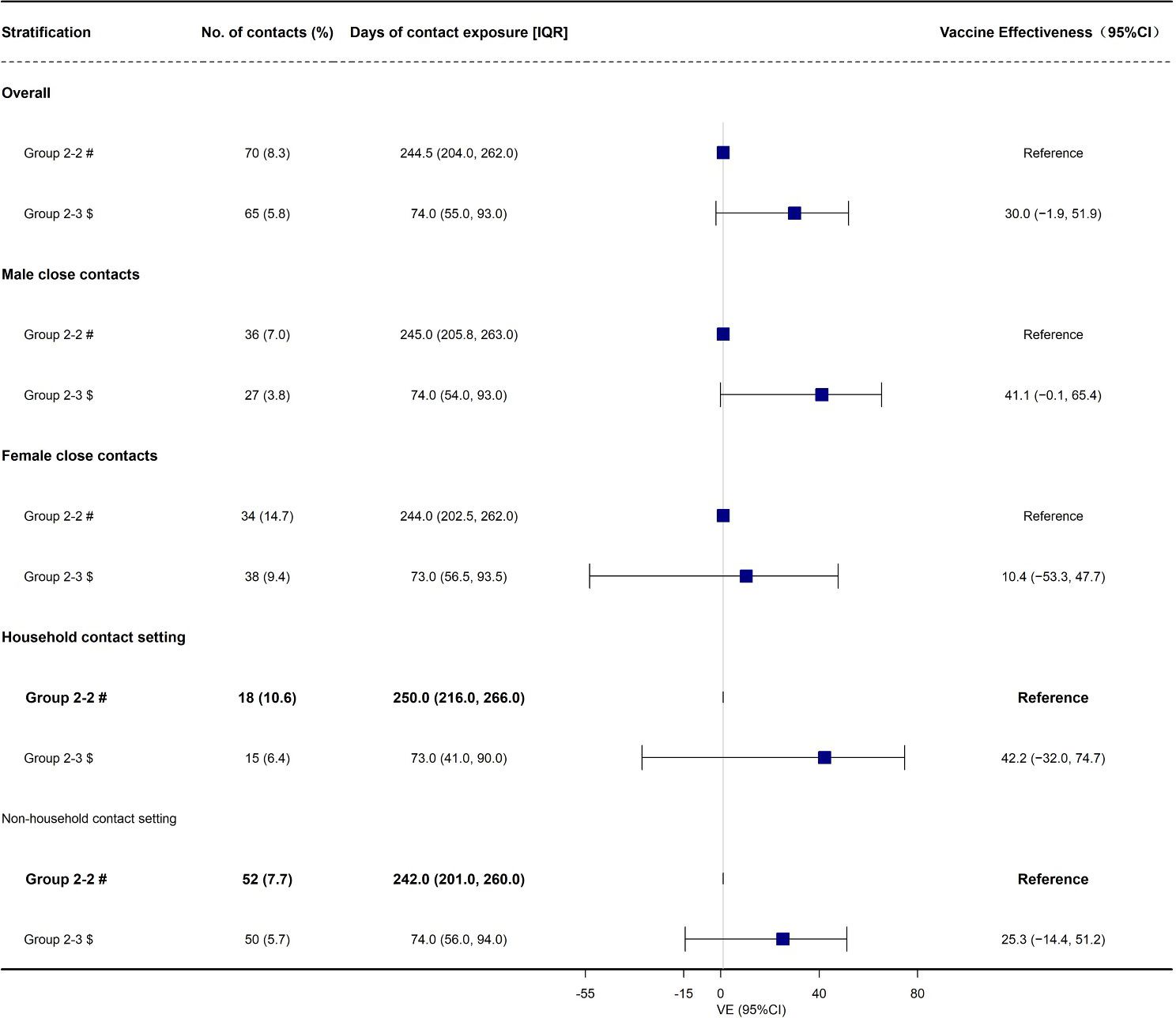
Figure 2 The effectiveness of booster Vaccination of inactivated COVID-19 vaccines against be infected (susceptibility) duo to Omicron BA.2 variant, in Shenzhen, China VE, vaccine effectiveness. # Fully vaccinated close contacts seeded by fully vaccinated index cases; $ Booster-vaccinated close contacts seeded by fully vaccinated index cases.
Then, we found that the overall effectiveness of booster vaccination of inactivated COVID-19 vaccines against infectiousness of Omicron BA.2 variant was 44.9% (95% CI: 19.7%, 62.2%, Table 3 , Figure 3 ). Moreover, we found that the effectiveness of booster vaccination of inactivated COVID-19 vaccines against infectiousness was 62.2% (95% CI: 32.0%, 78.9%) and 60.8% (95% CI: 38.5%, 75.1%) for the female close contacts and the non-household close contacts, respectively ( Table 3 , Figure 3 ). However, booster vaccination of inactivated COVID-19 vaccines against infectiousness did not provide any protective effect for the male close contacts and household close contacts ( Table 3 , Figure 3 ).
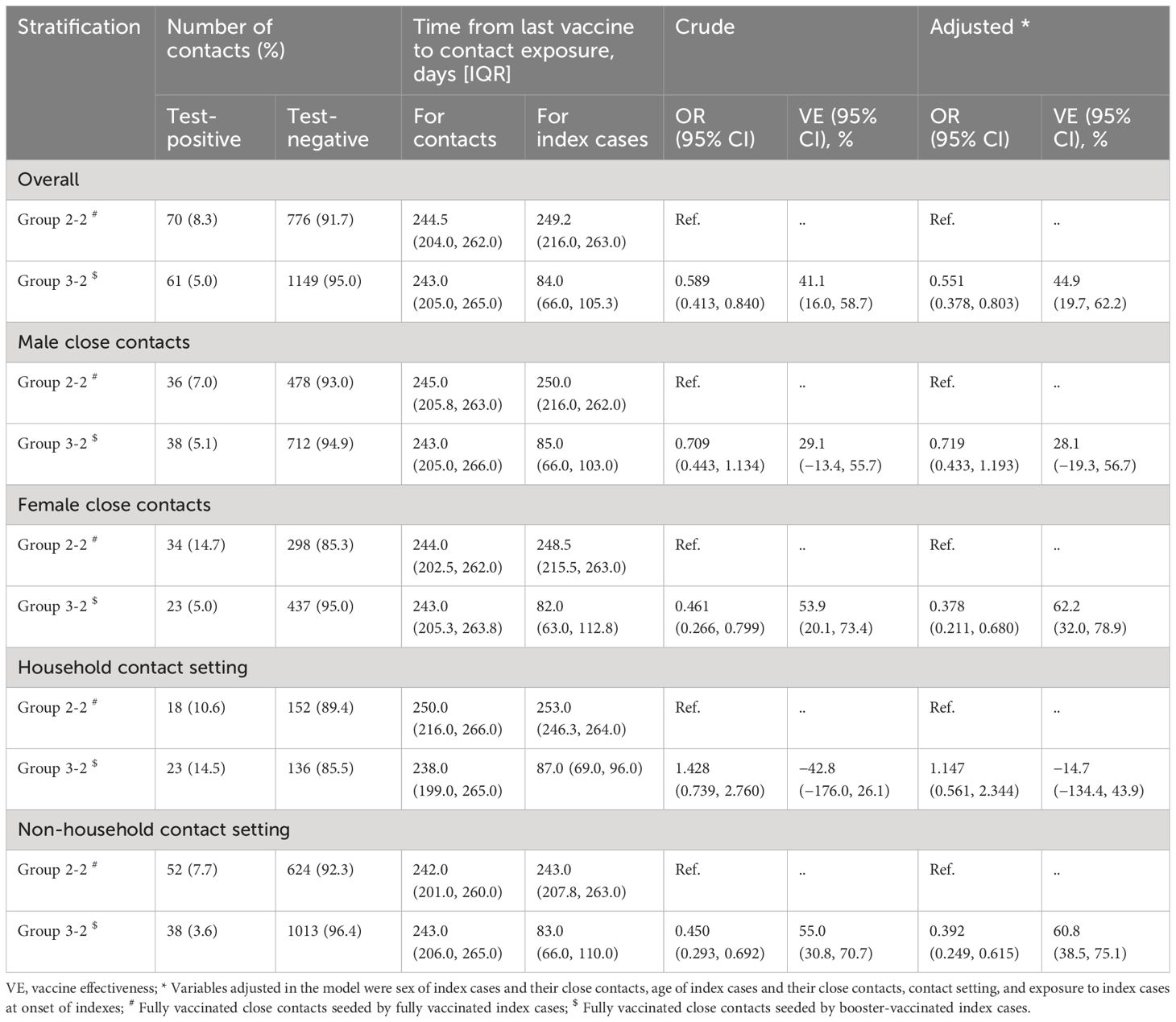
Table 3 The effectiveness of booster vaccination of inactivated COVID-19 vaccines against infectiousness of Omicron BA.2 variant, in Shenzhen, China.
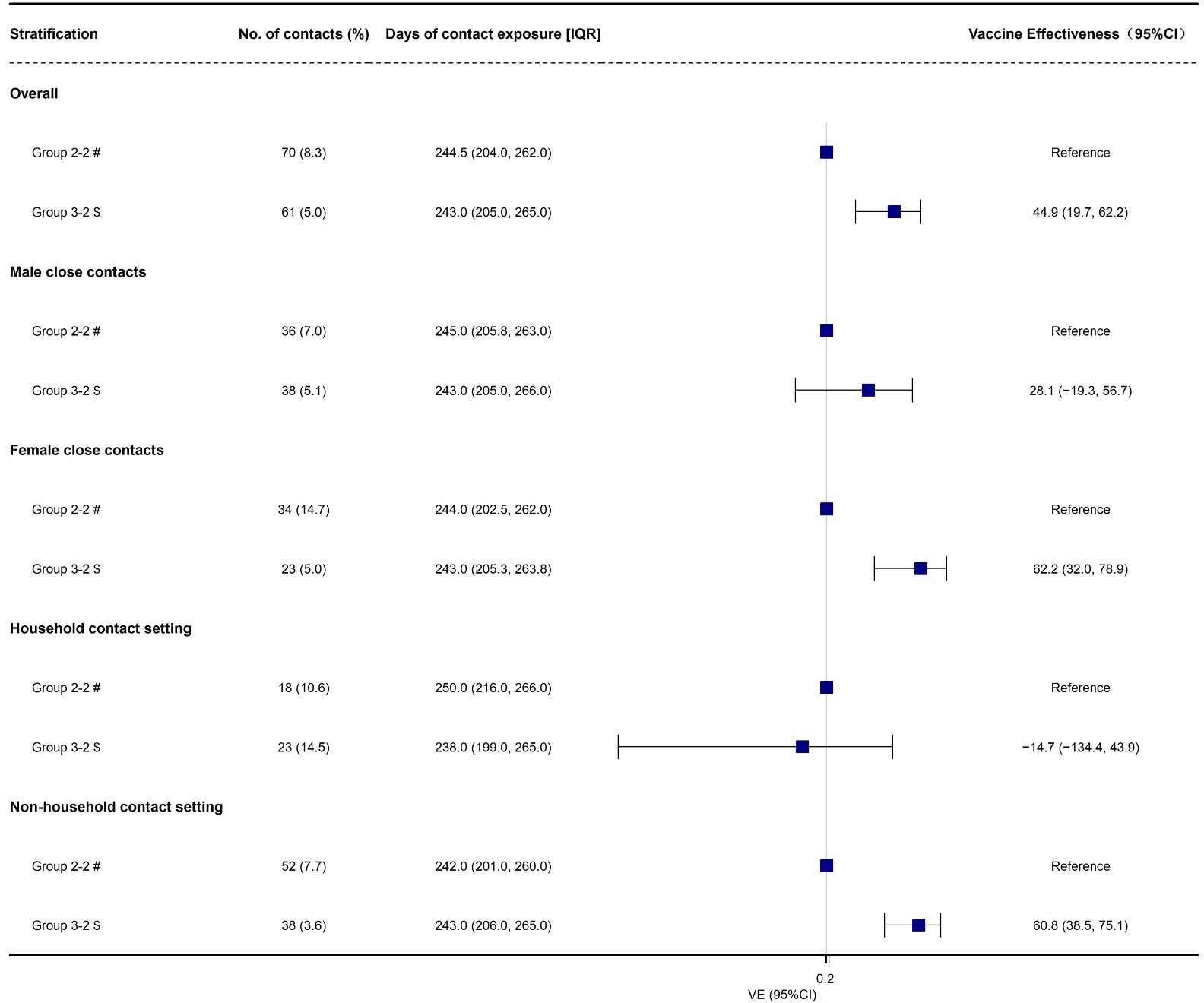
Figure 3 The effectiveness of booster vaccination of inactivated COVID-19 vaccines against infectiousness due to Omicron BA.2 variant, in Shenzhen, China VE, vaccine effectiveness. # Fully vaccinated close contacts seeded by fully vaccinated index cases; $ Fully vaccinated close contacts seeded by booster-vaccinated index cases.
Finally, we found that the overall effectiveness of booster vaccination of inactivated COVID-19 vaccines against transmission of Omicron BA.2 variant was 29.0% (95% CI: 3.2%, 47.9%, Table 4 , Figure 4 ). Moreover, we found that the effectiveness of booster vaccination of inactivated COVID-19 vaccines against transmission was 38.9% (95% CI: 3.3%, 61.3%) and 45.8% (95% CI: 22.1%, 62.3%) for the female close contacts and the non-household close contacts, respectively ( Table 4 , Figure 4 ). However, booster vaccination of inactivated COVID-19 vaccines against transmission did not provide any protective effect for the male close contacts and household close contacts ( Table 4 , Figure 4 ).
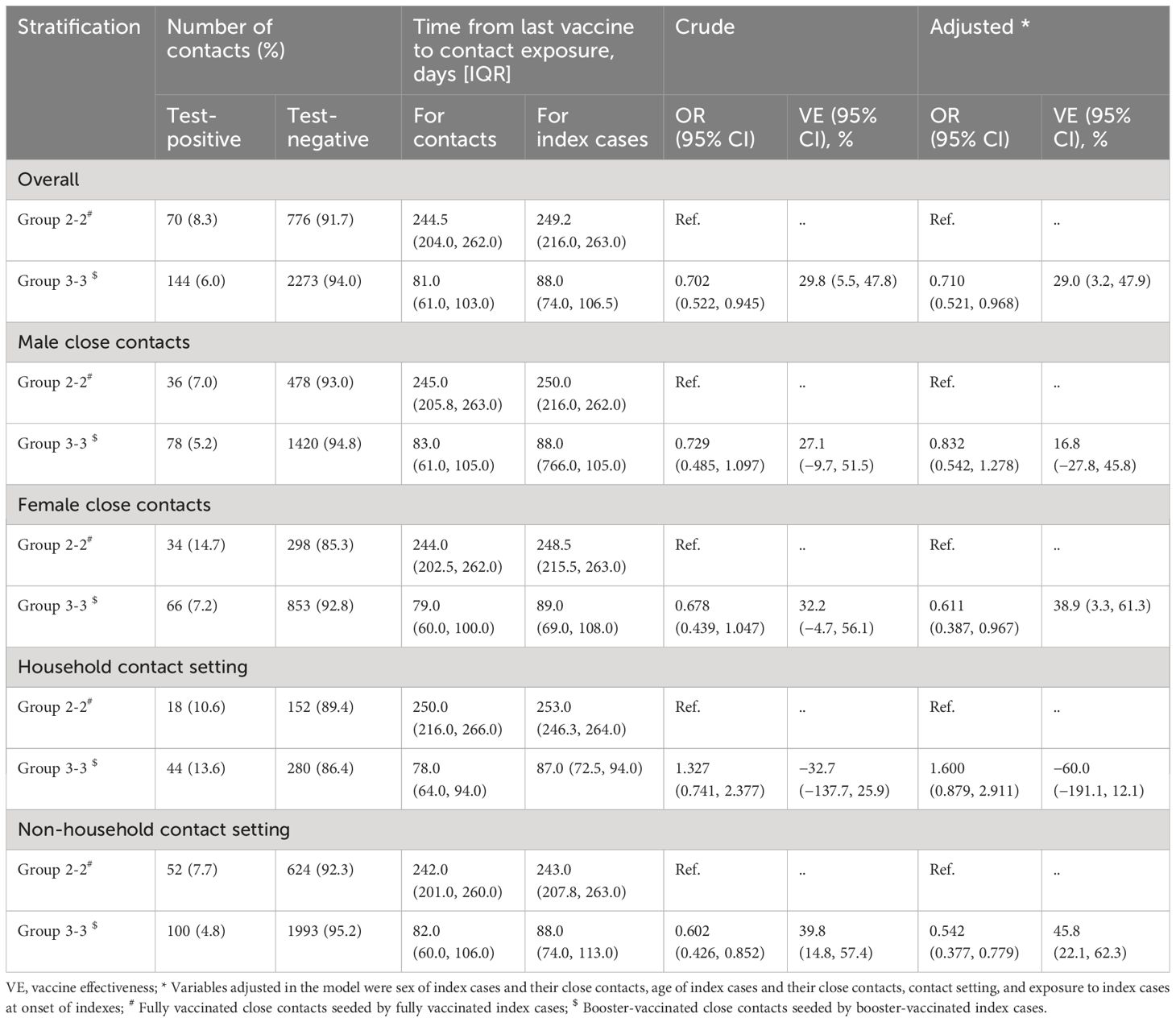
Table 4 The Effectiveness of booster vaccination of Inactivated COVID-19 Vaccines Against transmission of Omicron BA.2, in Shenzhen, China.
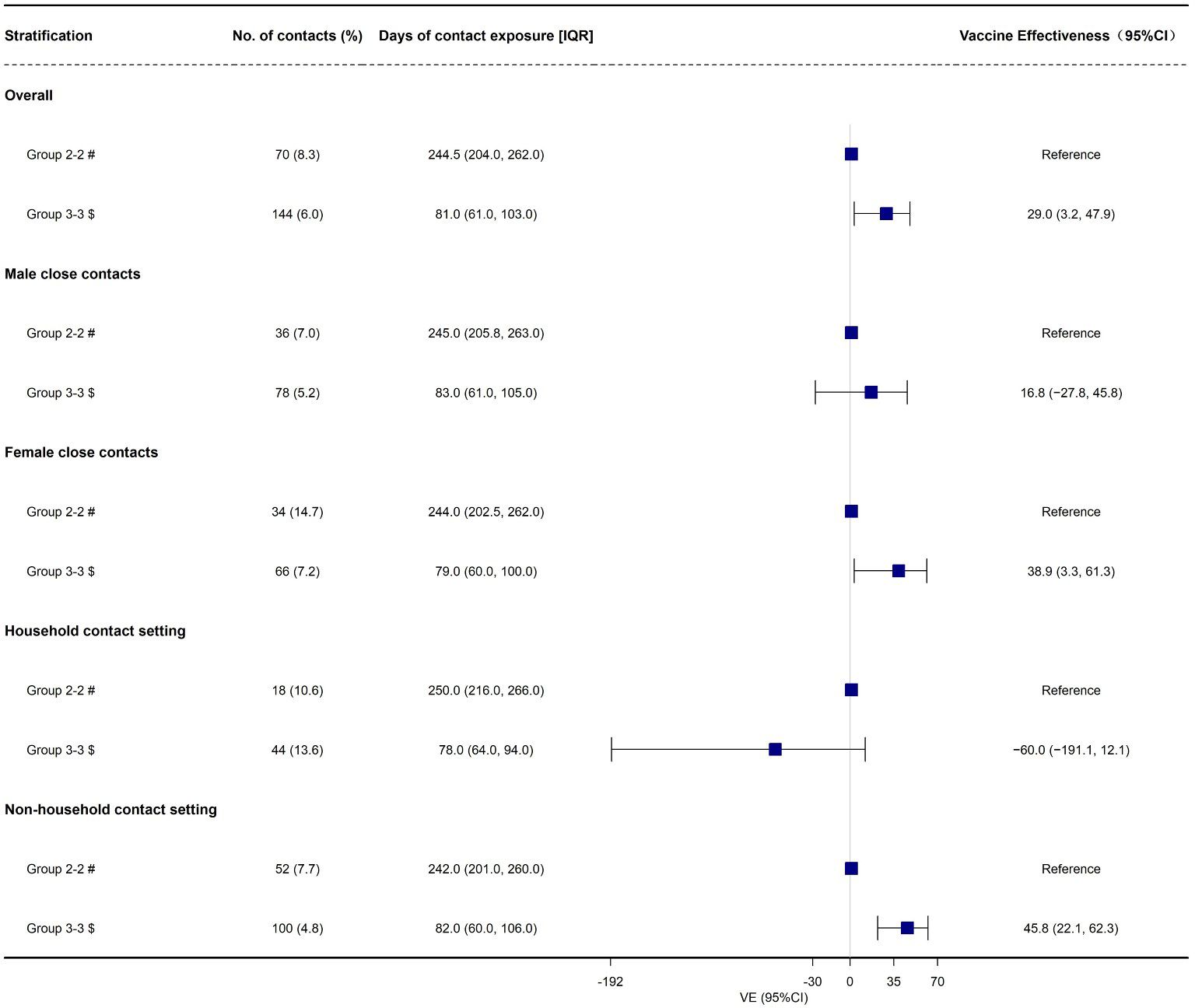
Figure 4 The effectiveness of booster vaccination of inactivated COVID-19 vaccines against transmission of Omicron BA.2, in Shenzhen, China. # Fully vaccinated close contacts seeded by fully vaccinated index cases; $ Booster-vaccinated close contacts seeded by booster-vaccinated index cases.
In this study, we conducted a retrospective cohort study to evaluate the effectiveness of booster vaccination of inactivated COVID-19 vaccines against being infected (susceptibility), infecting others (infectiousness), and spreading the COVID-19 among individuals (transmission) during an Omicron BA.2 sub-lineage outbreak in Shenzhen, China. Under the terms of the study, the stringent restriction measures were still active to prevent Omicron BA.2 infection and all the close contacts and their index cases were carefully traced and monitored. Consequently, we could assess the effectiveness of booster vaccination of inactivated COVID-19 vaccines against susceptibility, infectiousness, and transmission.
Several studies indicated that Omicron infection was less severe compared with previous SARS-CoV-2 waves, leading to a relatively low risk of COVID-19-related hospitalization and COVID-19-related death ( 39 – 42 ). However, this difference might be due to population natural immunity as well as COVID-19 vaccine-induced immunity. Therefore, researchers have great interest in estimating the VE against the Omicron different sub-lineages. An important issue is to evaluate the role of inactivated COVID-19 vaccine boosters against Omicron BA.2 infection caused by Omicron BA.2 sub-lineage.
In this study, our results indicated that booster vaccination of inactivated COVID-19 vaccines did not provide any protection against Omicron BA.2 infection. As far as we know, compared with full vaccination, only two published studies estimated the relative effectiveness of inactivated COVID-19 vaccine boosters against infection caused by Omicron BA.2 variant ( 35 , 43 ). Consistent with our results, a test-negative case-control study in Guangzhou, China found that booster immunization of inactivated COVID-19 vaccines did not provide any protective effect against Omicron BA.2 infection ( 35 ). Another retrospective cohort study found that the inactivated COVID-19 vaccine boosters only provided low level of protection against Omicron BA.2 infection ( 43 ). Although the present study found that booster vaccination of inactivated COVID-19 vaccines cannot offer effective protection against susceptibility caused by Omicron BA.2 variant. However, several published studies ( 24 , 30 – 34 , 44 ) indicated that the importance of COVID-19 vaccine boosters to mitigate the severity of COVID-19, especially in old adults, which is consistent with WHO recommendation about administration of COVID-19 vaccines for this group ( 45 ). Moreover, several previous studies ( 24 , 30 – 34 , 44 ) and meta-analyses ( 13 , 46 , 47 ) confirmed that COVID-19 vaccine boosters only provided low level of protection against infection caused by Omicron variant. These results indicated that the inactivated COVID-19 vaccine boosters were inefficient in preventing Omicron BA.2 epidemic. However, published studies were not comprehensive enough because the VE measured in their studies was restricted to evaluating against susceptibility (infection).
Another important aim of this study is to estimate the effectiveness of inactivated COVID-19 vaccine boosters against infectiousness and transmission caused by Omicron BA.2 variant. We found that the overall effectiveness of booster vaccination of inactivated COVID-19 vaccines against infectiousness was 44.9% and indicating that booster vaccination can reduce the infectivity among the infected individuals for adults aged ≥ 18 years older. The reduced infectiousness among booster-vaccinated COVID-19 cases can be because vaccination shortens the duration of time of high transmission potential, minimizes symptom duration, and furthermore may restrict tissue dissemination of active virus ( 48 – 50 ). VE against transmission which refers to the ability of COVID-19 vaccine to block the virus transmission chain and cut off community transmission. Overall, the inactivated vaccine boosters only provided a low level of protection in preventing transmission. Our results indicated that the individuals who received the booster vaccination had a 29.0% decreased risk of spreading the Omicron BA.2 virus from one to another. Then, we conducted subgroups according to gender and contact setting. We found that the inactivated COVID-19 vaccines boosters provided effective protection against infectiousness and transmission for the female and non-household close contacts. Further analysis observed that females had a higher secondary infection rate (14.7%) than males (7.0%) among group 2-2, which may be attributed to females having more frequent household contact with COVID-19 cases. Previous studies have indicated that the household setting is the main route of transmission due to more frequent and longer unprotected exposure to SARS-CoV-2 ( 51 , 52 ). However, it is needed to further confirm the differences of VE for gender.
Although we previously applied same data to evaluate the effectiveness of inactivated COVID-19 vaccine booster immunization against transmission in Shenzhen during a BA.2 outbreak period from 1 February to 21 April 2022 ( 53 ). However, we only considered the COVID-19 vaccination status of index cases. In this study, to further distinguishing the effectiveness of booster vaccination of inactivated COVID-19 vaccines, we applied four sub-cohorts according to the inactivated COVID-19 vaccination status of both the close contacts and their index cases to evaluate the effectiveness of inactivated vaccine booster immunization against susceptibility), infectiousness, and transmission. Therefore, the present study adds unique contributions to the scientific literature. First, it expanded on previous studies on the effectiveness of inactivated vaccine boosters only against infection (susceptibility). Second, it offered preliminary evidence of the effectiveness of inactivated vaccine boosters against susceptibility, infectiousness, and transmission caused by Omicron BA.2 subvariant using a cohort study design. Third, it was the first study to comprehensively assess the effectiveness of inactivated COVID-19 vaccine boosters against susceptibility, infectiousness, and transmission of Omicron BA.2 subvariant using contact tracing data. However, there were also several limitations. Firstly, this study did not assess the COVID-19-related hospitalization because all SARS-CoV-2 positive people must be isolated and hospitalized in China regardless of the severity of COVID-19 during the study period. Therefore, the current results cannot be extended to a more severe clinical range of COVID-19. Secondly, unmeasured exposure factors (e.g., conversation, shared room) might compromise the validity of our results although we had tried to control the known covariates. Thirdly, some behaviors (e.g., social distancing and personal protection) that also may affect our results. Fourthly, we did not consider mixing observations from different indexes and repeating observations from the same index due to data limitation in this study. Lastly, due to the short period of observation, we did not analyze the VE of inactivated COVID-19 vaccines from different vendors. Moreover, we also did not evaluate the duration of booster dose compared with full vaccination in this study.
In summary, booster vaccination of the inactivated COVID-19 vaccines provides low level of protection and moderate level of protection against Omicron BA.2 transmission and infectiousness, respectively. However, booster vaccination does not provide any protective effect against Omicron BA.2 susceptibility. These findings indicate that it is continuously needed assessing VE against the Omicron variant and may offer enough information to help develop COVID-19 vaccination strategies.
Data availability statement
The original contributions presented in the study are included in the article/supplementary material. Further inquiries can be directed to the corresponding authors.
Ethics statement
The studies involving humans were approved by China CDC Ethical Review Committee (approval number 202210). The studies were conducted in accordance with the local legislation and institutional requirements. Written informed consent for participation was not required from the participants or the participants’ legal guardians/next of kin in accordance with the national legislation and institutional requirements.
Author contributions
YuL: Data curation, Formal analysis, Investigation, Methodology, Project administration, Validation, Writing – original draft, Writing – review & editing. JS: Formal analysis, Writing – original draft, Writing – review & editing. JZ: Formal analysis, Writing – original draft, Writing – review & editing. ZQ: Investigation, Writing – review & editing. ZA: Investigation, Writing – review & editing. WG: Data curation, Investigation, Writing – review & editing. JW: Data curation, Investigation, Writing – review & editing. YiL: Data curation, Investigation, Writing – review & editing. XZ: Conceptualization, Investigation, Project administration, Supervision, Writing – review & editing. XH: Conceptualization, Data curation, Project administration, Supervision, Writing – original draft, Writing – review & editing.
The author(s) declare that no financial support was received for the research, authorship, and/or publication of this article.
Acknowledgments
We thank all staffs involved in the prevention and control of COVID-19 at the Shenzhen Center for Disease Control and Prevention.
Conflict of interest
The authors declare that the research was conducted in the absence of any commercial or financial relationships that could be construed as a potential conflict of interest.
Publisher’s note
All claims expressed in this article are solely those of the authors and do not necessarily represent those of their affiliated organizations, or those of the publisher, the editors and the reviewers. Any product that may be evaluated in this article, or claim that may be made by its manufacturer, is not guaranteed or endorsed by the publisher.
1. Li Q, Guan X, Wu P, Wang X, Zhou L, Tong Y, et al. Early transmission dynamics in Wuhan, China, of novel coronavirus-infected pneumonia. N Engl J Med . (2020) 382:1199–207. doi: 10.1056/NEJMoa2001316
PubMed Abstract | CrossRef Full Text | Google Scholar
2. Umakanthan S, Sahu P, Ranade AV, Bukelo MM, Rao JS, Abrahao-MaChado LF, et al. Origin, transmission, diagnosis and management of coronavirus disease 2019 (COVID-19). Postgrad Med J . (2020) 96:753–8. doi: 10.1136/postgradmedj-2020-138234
3. Habas K, Nganwuchu C, Shahzad F, Gopalan R, Haque M, Rahman S, et al. Resolution of coronavirus disease 2019 (COVID-19). Expert Rev Anti Infect Ther . (2020) 18:1201–11. doi: 10.1080/14787210.2020.1797487
4. WHO. Coronavirus disease (COVID-19) SARS-CoV-2-variants . World Health Organization (2023). Available at: https://www.who.int/en/activities/tracking-SARS-CoV-2-variants/ .
Google Scholar
5. Fan Y, Li X, Zhang L, Wan S, Zhang L, Zhou F. SARS-CoV-2 Omicron variant: recent progress and future perspectives. Signal Transduct Target Ther . (2022) 7:141. doi: 10.1038/s41392-022-00997-x
6. Thakur V, Ratho RK. OMICRON (B.1.1.529): A new SARS-CoV-2 variant of concern mounting worldwide fear. J Med Virol . (2022) 94:1821–4. doi: 10.1002/jmv.27541
7. Farahat RA, Abdelaal A, Umar TP, El-Sakka AA, Benmelouka AY, Albakri K, et al. The emergence of SARS-CoV-2 Omicron subvariants: current situation and future trends. Infez Med . (2022) b30:480–94. doi: 10.53854/liim-3004-2
CrossRef Full Text | Google Scholar
8. Farahat RA, Baklola M, Umar TP. Omicron B.1.1.529 subvariant: Brief evidence and future prospects. Ann Med Surg (Lond) . (2022) 83:104808. doi: 10.1016/j.amsu.2022.104808
9. Griffin M, Sohrabi C, Alsafi Z, Nicola M, Kerwan A, Mathew G, et al. Preparing for COVID-19 exit strategies. Ann Med Surg (Lond) . (2021) 61:88–92. doi: 10.1016/j.amsu.2020.12.012
10. Moore S, Hill EM, Tildesley MJ, Dyson L, Keeling MJ. Vaccination and non-pharmaceutical interventions for COVID-19: a mathematical modelling study. Lancet Infect Dis . (2021) 21:793–802. doi: 10.1016/S1473-3099(21)00143-2
11. Hodgson SH, Mansatta K, Mallett G, Harris V, Emary KRW, Pollard AJ. What defines an efficacious COVID-19 vaccine? A review of the challenges assessing the clinical efficacy of vaccines against SARS-CoV-2. Lancet Infect Dis . (2021) 21:e26–35. doi: 10.1016/S1473-3099(20)30773-8
12. Dagotto G, Yu J, Barouch DH. Approaches and challenges in SARS-coV-2 vaccine development. Cell Host Microbe . (2020) 28:364–70. doi: 10.1016/j.chom.2020.08.002
13. He X, Su J, Ma Y, Zhang W, Tang S. A comprehensive analysis of the efficacy and effectiveness of COVID-19 vaccines. Front Immunol . (2022) 13:945930. doi: 10.3389/fimmu.2022.945930
14. Al Kaabi N, Zhang Y, Xia S, Yang Y, Al Qahtani MM, Abdulrazzaq N, et al. Effect of 2 inactivated SARS-CoV-2 vaccines on symptomatic COVID-19 infection in adults: A randomized clinical trial. JAMA . (2021) 326:35–45. doi: 10.1001/jama.2021.8565
15. El Sahly HM, Baden LR, Essink B, Doblecki-Lewis S, Martin JM, Anderson EJ, et al. Efficacy of the mRNA-1273 SARS-CoV-2 vaccine at completion of blinded phase. N Engl J Med . (2021) 385:1774–85. doi: 10.1056/NEJMoa2113017
16. Frenck RW Jr, Klein NP, Kitchin N, Gurtman A, Absalon J, Lockhart S, et al. Safety, immunogenicity, and efficacy of the BNT162b2 covid-19 vaccine in adolescents. N Engl J Med . (2021) 385:239–50. doi: 10.1056/NEJMoa2107456
17. Heath PT, Galiza EP, Baxter DN, Boffito M, Browne D, Burns F, et al. Safety and efficacy of NVX-CoV2373 covid-19 vaccine. N Engl J Med . (2021) 385:1172–183. doi: 10.1056/NEJMoa2107659
18. Sadoff J, Gray G, Vandebosch A, Caírdenas V, Shukarev G, Grinsztejn B, et al. Safety and efficacy of single-dose Ad26.COV2.S vaccine against covid-19. N Engl J Med . (2021) 384:2187–201. doi: 10.1056/NEJMoa2101544
19. Tanriover MD, Doğanay HL, Akova M, Güner HR, Azap A, Akhan S, et al. Efficacy and safety of an inactivated whole-virion SARS-CoV-2 vaccine (CoronaVac): interim results of a double-blind, randomised, placebo-controlled, phase 3 trial in Turkey. Lancet . (2021) 398:213–22. doi: 10.1016/S0140-6736(21)01429-X
20. Thomas SJ, Moreira ED Jr, Kitchin N, Absalon J, Gurtman A, Lockhart S, et al. Safety and efficacy of the BNT162b2 mRNA covid-19 vaccine through 6 months. N Engl J Med . (2021) 385:1761–73. doi: 10.1056/NEJMoa2110345
21. Fadlyana E, Rusmil K, Tarigan R, Rahmadi AR, Prodjosoewojo S, Sofiatin Y, et al. A phase III, observer-blind, randomized, placebo-controlled study of the efficacy, safety, and immunogenicity of SARS-CoV-2 inactivated vaccine in healthy adults aged 18-59 years: An interim analysis in Indonesia. Vaccine . (2021) 39:6520–8. doi: 10.1016/j.vaccine.2021.09.052
22. Clemens SAC, Folegatti PM, Emary KRW, Weckx LY, Ratcliff J, Bibi S, et al. Efficacy of ChAdOx1 nCoV-19 (AZD1222) vaccine against SARS-CoV-2 lineages circulating in Brazil. Nat Commun . (2021) 12:5861. doi: 10.1038/s41467-021-25982-w
23. Bravo L, Smolenov I, Han HH, Li P, Hosain R, Rockhold F, et al. Efficacy of the adjuvanted subunit protein COVID-19 vaccine, SCB-2019: a phase 2 and 3 multicentre, double-blind, randomised, placebo-controlled trial. Lancet . (2022) 399:461–72. doi: 10.1016/S0140-6736(22)00055-1
24. Andeweg SP, de Gier B, Eggink D, van den Ende C, van Maarseveen N, Ali L, et al. Protection of COVID-19 vaccination and previous infection against Omicron BA.1, BA.2 and Delta SARS-CoV-2 infections. Nat Commun . (2022) 13:4738. doi: 10.1038/s41467-022-31838-8
25. Lauring AS, Tenforde MW, Chappell JD, Gaglani M, Ginde AA, McNeal T, et al. Clinical severity of, and effectiveness of mRNA vaccines against, covid-19 from omicron, delta, and alpha SARS-CoV-2 variants in the United States: prospective observational study. BMJ . (2022) 376:e069761. doi: 10.1136/bmj-2021-069761
26. Powell AA, Kirsebom F, Stowe J, Ramsay ME, Lopez-Bernal J, Andrews N, et al. Protection against symptomatic infection with delta (B.1.617.2) and omicron (B.1.1.529) BA.1 and BA.2 SARS-CoV-2 variants after previous infection and vaccination in adolescents in England, August, 2021-March, 2022: a national, observational, test-negative, case-control study. Lancet Infect Dis . (2023) 23:435–44. doi: 10.1016/S1473-3099(22)00729-0
27. Price AM, Olson SM, Newhams MM, Halasa NB, Boom JA, Sahni LC, et al. BNT162b2 protection against the omicron variant in children and adolescents. N Engl J Med . (2022) 386:1899–909. doi: 10.1056/NEJMoa2202826
28. Tseng HF, Ackerson BK, Luo Y, Sy LS, Talarico CA, Tian Y, et al. Effectiveness of mRNA-1273 against SARS-CoV-2 Omicron and Delta variants. Nat Med . (2022) 28:1063–071. doi: 10.1038/s41591-022-01753-y
29. Solante R, Alvarez-Moreno C, Burhan E, Chariyalertsak S, Chiu NC, Chuenkitmongkol S, et al. Further implications on the global real-world vaccine effectiveness against SARS-CoV-2. Expert Rev Vaccines . (2022) 21:1355–7. doi: 10.1080/14760584.2022.2110073
30. Huang Z, Xu S, Liu J, Wu L, Qiu J, Wang N, et al. Effectiveness of inactivated and Ad5-nCoV COVID-19 vaccines against SARS-CoV-2 Omicron BA. 2 variant infection, severe illness, and death. BMC Med . (2022) 20:400. doi: 10.1186/s12916-022-02606-8
31. McMenamin ME, Nealon J, Lin Y, Wong JY, Cheung JK, Lau EHY, et al. Vaccine effectiveness of one, two, and three doses of BNT162b2 and CoronaVac against COVID-19 in Hong Kong: a population-based observational study. Lancet Infect Dis . (2022) 22:1435–43. doi: 10.1016/S1473-3099(22)00345-0
32. Wan EYF, Mok AHY, Yan VKC, Wang B, Zhang R, Hong SN, et al. Vaccine effectiveness of BNT162b2 and CoronaVac against SARS-CoV-2 Omicron BA.2 infection, hospitalisation, severe complications, cardiovascular disease and mortality in patients with diabetes mellitus: a case control study. J Infect . (2022) 85:e140–4. doi: 10.1016/j.jinf.2022.08.008
33. Cheng FWT, Fan M, Wong CKH, Chui CSL, Lai FTT, Li X, et al. The effectiveness and safety of mRNA (BNT162b2) and inactivated (CoronaVac) COVID-19 vaccines among individuals with chronic kidney diseases. Kidney Int . (2022) 102:922–5. doi: 10.1016/j.kint.2022.07.018
34. Tang L, Wang FZ, Rodewald LE, Wang XY, Liu SY, Liu QQ, et al. Real-world effectiveness of primary series and booster doses of inactivated COVID-19 vaccine against Omicron BA.2 variant infection in China: a retrospective cohort study. J Infect Dis . (2023) 228:261–9. doi: 10.1093/infdis/jiad090
35. Zhang D, Zhong J, Xiong H, Li Y, Guo T, Peng B, et al. Protective effect of inactivated COVID-19 vaccines against omicron BA.2 infection in Guangzhou: A test-negative case-control real-world study. Vaccines (Basel) . (2023) 11:566. doi: 10.3390/vaccines11030566
36. de Gier B, Andeweg S, Joosten R, Ter Schegget R, Smorenburg N, van de Kassteele J, et al. Vaccine effectiveness against SARS-CoV-2 transmission and infections among household and other close contacts of confirmed cases, the Netherlands, February to May 2021. Euro Surveill . (2021) 26:2100640. doi: 10.2807/1560-7917.ES.2021.26.31.2100640
37. Lyngse FP, Kirkeby CT, Denwood M, Christiansen LE, Mølbak K, Møller CH, et al. Household transmission of SARS-CoV-2 omicron varian of concern subvariants BA.1 and BA.2 in Denmark. Nat Commun . (2022) 13:5760. doi: 10.1038/s41467-022-33498-0
38. Wang K, Guo Z, Zeng T, Sun S, Lu Y, Wang J, et al. Transmission characteristics and inactivated vaccine effectiveness against transmission of SARS-coV-2 omicron BA.5 variants in Urumqi, China. JAMA Netw Open . (2023) 6:e235755. doi: 10.1001/jamanetworkopen.2023.5755
39. Abdullah F, Myers J, Basu D, Tintinger G, Ueckermann V, Mathebula M, et al. Decreased severity of disease during the first global omicron variant COVID-19 outbreak in a large hospital in Tshwane, South Africa. Int J Infect Dis . (2022) 116:38–42. doi: 10.1016/j.ijid.2021.12.357
40. Houhamdi L, Gautret P, Hoang VT, Fournier P-E, Colson P, Raoult D. Characteristics of the first 1119 SARS-CoV-2 omicron variant cases, in Marseille, France, November-December 2021. J Med Virol . (2022) 94:2290–5. doi: 10.1002/jmv.27613
41. Petersen MS, Kongsstovu S Í, Eliasen EH, Larsen S, Hansen JL, Vest N, et al. Clinical characteristics of the Omicron variant - results from a Nationwide Symptoms Survey in the Faroe Islands. Int J Infect Dis . (2022) 122:636–43. doi: 10.1016/j.ijid.2022.07.005
42. Ulloa AC, Buchan SA, Daneman N, Brown KA. Estimates of SARS-CoV-2 omicron variant severity in Ontario, Canada. JAMA . (2022) 327:1286–8. doi: 10.1001/jama.2022.2274
43. He X, Zeng B, Wang Y, Pang Y, Zhang M, Hu T, et al. Effectiveness of booster vaccination with inactivated COVID-19 vaccines against SARS-CoV-2 Omicron BA.2 infection in Guangdong, China: a cohort study. Front Immunol . (2023) 14:1257360. doi: 10.3389/fimmu.2023.1257360
44. Tartof SY, Slezak JM, Puzniak L, Hong V, Frankland TB, Xie F, et al. Effectiveness and durability of BNT162b2 vaccine against hospital and emergency department admissions due to SARS-CoV-2 omicron sub-lineages BA.1 and BA.2 in a large health system in the USA: a test-negative, case-control study. Lancet Respir Med . (2023) 11:176–87. doi: 10.1016/S2213-2600(22)00354-X
45. WHO. The Sinovac-CoronaVac COVID-19 vaccine: what you need to know . World Health Organization (2021). Available at: https://www.who.int/news-room/feature-stories/detail/the-sinovac-covid-19-vaccine-what-you-need-to-know .
46. Xu S, Li J, Wang H, Wang F, Yin Z, Wang Z. Real-world effectiveness and factors associated with effectiveness of inactivated SARS-CoV-2 vaccines: a systematic review and meta-regression analysis. BMC Med . (2023) 21:160. doi: 10.1186/s12916-023-02861-3
47. Mohammed H, Pham-Tran DD, Yeoh ZYM, Wang B, McMillan M, Andraweera PH, et al. A systematic review and meta-analysis on the real-world effectiveness of COVID-19 vaccines against infection, symptomatic and severe COVID-19 disease caused by the omicron variant (B.1.1.529). Vaccines (Basel) . (2023) 11:224. doi: 10.3390/vaccines11020224
48. Tian D, Song Y, Zhang M, Pan Y, Ge Z, Zhang Y, et al. Genomic, immunological, and clinical analysis of COVID-19 vaccine breakthrough infections in Beijing, China. J Med Virol . (2022) 94:2237–49. doi: 10.1002/jmv.27636
49. Li D, Li AE, Li ZQ, Bao Y, Liu T, Qin XR, et al. SARS-coV-2 delta variant in Jingmen City, Hubei Province, China, 2021: children susceptible and vaccination breakthrough infection. Front Microbiol . (2022) 13:856757. doi: 10.3389/fmicb.2022.856757
50. Ke R, Martinez PP, Smith RL, Gibson LL, Achenbach CJ, McFall S, et al. Longitudinal analysis of SARS-coV-2 vaccine breakthrough infections reveals limited infectious virus shedding and restricted tissue distribution. Open Forum Infect Dis . (2022) 9:ofac192. doi: 10.1093/ofid/ofac192
51. Madewell ZJ, Yang Y, Longini IM Jr, Halloran ME, Dean NE. Household secondary attack rates of SARS-coV-2 by variant and vaccination status: an updated systematic review and meta-analysis. JAMA Netw Open . (2022) 5:e229317. doi: 10.1001/jamanetworkopen.2022.9317
52. Madewell ZJ, Yang Y, Longini IM Jr, Halloran ME, Dean NE. Household transmission of SARS-coV-2: A systematic review and meta-analysis. JAMA Netw Open . (2020) 3:e2031756. doi: 10.1001/jamanetworkopen.2020.31756
53. He X, Liao Y, Liang Y, Yu J, Gao W, Wan J, et al. Transmission characteristics and inactivated vaccine effectiveness against transmission of the SARS-CoV-2 Omicron BA.2 variant in Shenzhen, China. Front Immunol . (2024) 14:1290279. doi: 10.3389/fimmu.2023.1290279
Keywords: COVID-19, vaccine effectiveness, BA.2 subvariant, susceptibility, infectiousness, transmission
Citation: Liao Y, Su J, Zhao J, Qin Z, Zhang Z’A, Gao W, Wan J, Liao Y, Zou X and He X (2024) The effectiveness of booster vaccination of inactivated COVID-19 vaccines against susceptibility, infectiousness, and transmission of omicron BA.2 variant: a retrospective cohort study in Shenzhen, China. Front. Immunol. 15:1359380. doi: 10.3389/fimmu.2024.1359380
Received: 21 December 2023; Accepted: 04 April 2024; Published: 31 May 2024.
Reviewed by:
Copyright © 2024 Liao, Su, Zhao, Qin, Zhang, Gao, Wan, Liao, Zou and He. This is an open-access article distributed under the terms of the Creative Commons Attribution License (CC BY) . The use, distribution or reproduction in other forums is permitted, provided the original author(s) and the copyright owner(s) are credited and that the original publication in this journal is cited, in accordance with accepted academic practice. No use, distribution or reproduction is permitted which does not comply with these terms.
*Correspondence: Xuan Zou, [email protected] ; Xiaofeng He, [email protected]
† These authors have contributed equally to this work and share first authorship
Disclaimer: All claims expressed in this article are solely those of the authors and do not necessarily represent those of their affiliated organizations, or those of the publisher, the editors and the reviewers. Any product that may be evaluated in this article or claim that may be made by its manufacturer is not guaranteed or endorsed by the publisher.
- Open access
- Published: 03 June 2024
Efficacy and safety of ultrasound-guided thermal ablation of graves’ disease: a retrospective cohort study
- Guangzhen Cai 1 na1 ,
- Beilin Luo 2 na1 ,
- Maolin Wang 1 ,
- Jiqin Su 1 ,
- Luping Lin 3 ,
- Guibin Li 1 ,
- Xiangru Chen 1 ,
- Zhishu Huang 1 ,
- Peiyi Lin 1 ,
- Shengwei Liu 1 ,
- Huidi Yan 1 &
- Lixin Zhou 1
Thyroid Research volume 17 , Article number: 10 ( 2024 ) Cite this article
Metrics details
Ultrasound-guided thermal ablation (TA) has emerged as a robust therapeutic approach for treating solid tumors in multiple organs, including the thyroid. Yet, its efficacy and safety profile in the management of Graves’ Disease (GD) remains to be definitively established.
A retrospective study was conducted on 50 GD patients treated with TA between October 2017 and December 2021. Key metrics like thyroid volume, volume reduction rate (VRR), thyroid hormones, and basal metabolic rate (BMR) were evaluated using paired Wilcoxon tests.
The intervention of ultrasound-guided TA yielded a statistically significant diminution in total thyroid volume across all postoperative follow-up intervals—1, 3, 6, and 12 months—relative to pre-intervention baselines ( p < 0.001). The median VRR observed at these time points were 17.5%, 26.5%, 34.4%, and 39.8%, respectively. Euthyroid status was corroborated in 96% of patients at the one-year follow-up milestone. Transient tachycardia and dysphonia were observed in three patients, while a solitary case of skin numbness was noted. Crucially, no instances of enduring injury to the recurrent laryngeal nerve (RLN) were documented.
Conclusions
Our investigation substantiates ultrasound-guided TA as a pragmatic, well-tolerated, and safe therapeutic modality for GD. It effectively improves symptoms of hyperthyroidism, engenders a substantial reduction in thyroid volume, and restores thyroid hormone and BMR to physiological levels. Given its favorable safety profile, enhanced cosmetic outcomes, and minimally invasive nature, ultrasound-guided TA is a compelling alternative to thyroidectomy for GD patients.
Introduction
Hyperthyroidism, characterized by the thyroid gland’s excessive secretion and synthesis of thyroid hormones, exerts detrimental effects on various physiological systems, including the nervous, circulatory, and digestive systems [ 1 ]. Such symptoms significantly impair patients’ quality of life. GD is the predominant cause of hyperthyroidism, accounting for up to 80% of cases in iodine-sufficient regions [ 2 ]. The estimated annual incidence of GD ranges from 20 to 50 per 100,000 individuals and continues to rise [ 3 , 4 ].
Currently, the primary therapeutic options for GD include antithyroid drugs (ATDs), radioactive iodine (RAI) therapy, and thyroidectomy [ 5 ]. While ATDs can alleviate symptoms and enhance life quality, they are associated with a high recurrence rate of 50–60% and potentially severe complications such as agranulocytosis, drug-induced liver injury, and even liver failure [ 6 ]. RAI therapy offers a lower recurrence rate but may exacerbate Graves’ ophthalmopathy (GO), induce acute thyroiditis, and increase cancer risk [ 7 ]. Thyroidectomy provides a high cure rate but carries the risk of long-term adverse effects like hypothyroidism, RLN paralysis, and scarring [ 8 , 9 ].
Ultrasound-guided TA has emerged as a promising alternative to traditional treatments. This technique employs an internally cooled radiofrequency catheter inserted into the target nodule under ultrasound guidance to generate heat through alternating current. This heat induces irreversible tissue damage, resulting in coagulation necrosis [ 10 , 11 ]. TA offers several advantages over traditional treatments, including higher precision, minimal invasiveness, quicker recovery, and repeatability [ 12 ]. Compared to thyroidectomy, TA is less traumatic, more cosmetically appealing, and more cost-effective [ 13 ]. Additionally, TA avoids the complications associated with RAI therapy, such as worsening of GO and acute thyroiditis [ 14 ].
Despite these advantages, there is a paucity of research evaluating the efficacy and safety of ultrasound-guided TA specifically for GD. This study aims to fill this gap by conducting a retrospective cohort study of GD patients treated with TA at our center between October 2017 and December 2021. Our objective is to establish TA as a viable, safe, and effective treatment modality for GD, thereby providing a reference for personalized treatment plans.
Study design
This study was a single-center, retrospective investigation conducted in accordance with the Declaration of Helsinki and approved by the Institutional Review Board of Xiamen Medical University (Ethical Approval No. 2,023,052). All participants or their legal guardians provided written informed consent. Our study protocol entailed treating patients with GD using ultrasound-guided TA, with subsequent follow-up visits scheduled at 1, 3, 6, and 12 months after the procedure. The procedure was performed by Dr. Lixin Zhou, a thyroid surgeon with extensive experience in managing thyroid diseases.
Recruited cohort
GD diagnosis was based on clinical manifestations of hyperthyroidism, diffuse thyroid enlargement, suppressed serum thyroid Stimulating Hormone (TSH) levels, and elevated serum thyroid hormone concentrations [ 15 , 16 , 17 , 18 ]. Additional diagnostic criteria included exophthalmos, pretibial myxedema, and elevated thyroid-stimulating hormone receptor antibodies (TRAb) levels. In this study, the measurement of TRAb levels served as a substitute for thyroid stimulating antibodies (TSAb) levels, both for the diagnosis and for the prognostic evaluation of GD.
Patients who met these diagnostic criteria were included in the study. Those who declined ATDs, RIT, or surgical intervention for various reasons—such as ATD treatment failure, reluctance for long-term medication, concerns about radioactivity, cosmetic considerations, or risk of postoperative hypothyroidism—were also eligible. In total, 50 out of 57 patients who met these criteria between October 2017 and December 2021 were included in the study, with seven lost to follow-up.
Monitoring and follow-up
Clinical characteristics, preoperative symptoms, and postoperative complications were meticulously recorded. Thyroid volume was assessed post-procedure using ultrasound imaging, employing the ellipsoid volume formula [ 19 ]. The VRR was calculated using the formula [(V0-V1)/V0] x 100, where V0 and V1 represent baseline and post-ablation thyroid volumes, respectively. Descriptive statistics were used to summarize changes in various clinical parameters, including thyroid hormone levels and BMR, calculated using the Gale method [ 20 ].
Ultrasound-guided thermal ablation (TA) can be executed utilizing various modalities, including microwave ablation (MWA), radiofrequency ablation (RFA), or laser techniques. Microwave ablation (MWA) therapy is characterized by rapid inactivation of the target tissue with a larger scope, shorter ablation time, less operator fatigue, and a more manageable goal of near-total thyroid ablation. Radiofrequency ablation (RFA) is also used, but the time required to ablate the same volume of target tissue is longer than that of microwave, and laser ablation requires even more time. At our center, microwave and radiofrequency techniques are primarily used for TA. The portable color ultrasound M-Turb (Sono Sound) is employed for ultrasonic image acquisition and TA guidance. The probe for detecting external organs is a 4–9 MHz linear array probe. RFA uses an S-1500 radiofrequency therapy system (Shanghai Meide Medical Co., Ltd.); a radiofrequency catheter model 10-131181 is used, with an active tip of 5, 7, 10, or 15 mm according to the baseline nodule volume. MWA is performed using an ECO-100A1 microwave treatment instrument (YIGAO Microwave System Engineering Co., Ltd., Nanjing, Jiangsu Province, China) and an ECO-100AI3 superficial organ ablation needle (16 G, total length: 10 cm, microwave transmitter away from the shaft tip: 3 mm). The power is set at 35 W, and the adjustment range is 30–40 W. In this study, the selection between MWA and RFA was determined by the technological advancements and equipment availability at our facility during different time periods, rather than by patient-specific factors. This approach was integral to our methodology and is critical for understanding the context of our results. Specifically, from October 2017 to December 2019, our facility was equipped with and hence utilized MWA for treating patients. As our technological capabilities evolved, we transitioned to using RFA from January 2020 to December 2021.
Procedural details
Before undergoing ultrasound-guided TA for hyperthyroidism, patients were treated with ATD to control symptoms of thyrotoxicosis. For patients with a heart rate above 90 beats/min, add β-adrenergic blockers. For patients with difficulty controlling thyrotoxicosis and taking ATD treatment and patients with second-degree thyroid enlargement and above, potassium iodide oral solution is added (Start taking Luger’s solution 14 days before the procedure: 3 drops/time, three times/day, add one drop daily; continue to take it for seven days after the procedure, reduce one drop daily to 9 drops/time and stop the drug) [ 16 , 17 , 21 ].
To ensure that patients are fully informed and able to choose the treatment plan independently, the procedure consent form must be signed by the patient prior to the procedure. Patients were advised to abstain from food and drink for at least six hours prior to the procedure. Prior to the procedure, a routine ultrasound measurement of thyroid size and Color Doppler Flow Imaging (CDFI) to show thyroid blood perfusion is performed, and the puncture route for TA is planned.
The patient is positioned supine with hyperextended neck. The surgical area is routinely disinfected and draped. Conventional ultrasound is used to determine the location, size, and vascular distribution of the thyroid. Layered infiltration anesthesia under ultrasound guidance using 0.5% lidocaine is performed to separate the thyroid from the pre-thyroid muscles, forming an isolation zone to block heat conduction and prevent damage to surrounding structures. The space behind the thyroid capsule is usually not isolated to avoid tearing and bleeding caused by forced separation due to adhesion of the thyroid to the surrounding large blood vessels. A surgical incision is made in the midline of the neck at the projection of the thyroid isthmus. Under ultrasound guidance, the tip of the puncture needle is inserted into the thyroid, penetrating one side of the gland through the isthmus. The path is from the anterior and inner side next to the trachea, about 0.3 to 0.5 cm, puncturing to the deep part of the thyroid. The needle tip approaches the dorsal capsule of the gland but does not penetrate the thyroid capsule. The gland is ablated from inside to outside in a stepwise manner. During puncture, the needle tip is always kept within the ultrasound-visible range. The preset power range is 30 to 40 W. The thyroid is considered as multiple sections, starting from the middle part of the thyroid and ablating layer by layer upwards and downwards. Each layer uses an ablating sequence from inside to outside, deep to shallow. During ablation, a normal gland thickness of about 0.3 to 0.5 cm near the trachea should be preserved to avoid ablating the danger triangle area and protect the recurrent laryngeal nerve. The Moving-shot ablation technique is used, which can achieve near-total ablation of the hyperthyroid gland. The Moving-shot technique is used to ablate the glands sequentially from the inside to the outside until a gas response with hyperechoic gas is observed [ 22 , 23 ]. After satisfactory ablation on one side, communication with the patient is made to confirm that there is no change in voice, then the same anesthesia and ablation treatment is applied to the other side of the thyroid. For patients with thick isthmus tissue, ablation of the thyroid isthmus is performed to complete near-total ablation treatment of both lobes and the isthmus of the thyroid in one go.
The ablation range is equivalent to the range of near-total thyroidectomy, only preserving a thin layer of glandular tissue on the inner posterior sides of the trachea, namely the danger triangle area [ 24 ], with a thickness of about 0.3 cm, measuring approximately 1.0 cm × 1.5 cm, about 3 g of thyroid tissue. This ensures the safety of important organs such as neck nerves, blood vessels, trachea, and parathyroid glands while achieving near-total thyroid ablation.
Following the TA procedure, the patient’s neck is protected from burns by applying compression ice while pressure is applied to stop bleeding and alleviate discomfort. On the first day after the procedure, the patient is closely monitored for complications such as fever, bleeding, dyspnea, cough, hoarseness, and thyroid storm. Additionally, thyroid function is tested during this time. After successful observation, the patient is discharged on the second day after the procedure.
To maintain thyroid function and manage any residual thyrotoxicosis, patients are treated with ATD, β-adrenergic blockers, and potassium iodide oral solution after the procedure [ 25 ]. It is important to note that the potassium iodide solution should be discontinued within ten days after the procedure [ 26 ]. Treatment with these drugs is typically maintained for approximately three months, after which they are gradually discontinued once symptoms of thyrotoxicosis have resolved and thyroid function has returned to normal levels. Three months after the TA, patients continued exhibiting elevated thyroid hormone levels, paired with suppressed thyroid-stimulating hormone levels. Even upon cessation of ATDs, these individuals maintained high levels of thyroid hormones and diminished TSH levels. The tapering of ATD dosage presented a challenge in preserving normal thyroid hormone levels. The above patients necessitate a secondary TA of the residual thyroid tissue.
In patients co-diagnosed with GD and active GO, prophylactic glucocorticoids are prescribed to curtail the advancement of GO post-TA and to address any ophthalmic complications that may arise postoperatively.
Statistical analysis
Enumeration data were presented as frequencies and rates, while measurement data were expressed as medians ± one quartile. The Fisher exact test was employed to evaluate dichotomous variables between populations. The significance of the difference in the means of continuous variables between patients who underwent RFA and MWA was assessed via a two-tailed unpaired Wilcoxon test. A two-tailed paired Wilcoxon test was performed to compare the measurements before and after the procedure. The ggplot package was utilized to generate visualizations of thyroid volume changes. A p-value less than 0.05 was considered statistically significant. Statistical analysis was conducted using R statistics software (version 4.2.1).
Clinical information
In our retrospective cohort study, we assessed a total of 50 patients diagnosed with GD, all of whom underwent ultrasound-guided TA. The patients were divided into two treatment groups: 29 received RFA, accounting for 58% of the cohort, and 21 underwent MWA, making up the remaining 42%. Notably, the majority of the study population was female, with 41 out of 50 patients (82%), aligning with the gender distribution reported in recent literature on GD [ 27 ]. Prior to the TA procedure, various measures were taken to stabilize the patient’s cardiac rhythm. Specifically, 12 patients (24%) were prescribed propranolol. Additionally, the ATD methimazole (MMI) was administered to 34 patients (68%) as a preoperative measure (Table 1 ).
In terms of procedure duration, the median time for the RFA group was 47 min (IQR: 40-53.25), whereas for the MWA group, it was 43 min (IQR: 39.5–48). Upon statistical analysis, we identified a significant variance between the RFA and MWA groups concerning procedure duration ( p = 0.02). However, the length of hospital stay did not significantly differ between the two groups, with a median of 2 days (IQR: 2–3; p = 0.31). Multiple procedures were performed on 19 patients in the RFA group (65.5%) and 11 in the MWA group (52.4%). Fisher’s exact test showed no significant difference between the two groups in the incidence of multiple procedures ( p = 0.39). Similarly, the time interval between the first and last TA procedures did not significantly differ between the two groups, averaging 8.4 months (IQR: 4.8–14.1) for RFA and 7.2 months (IQR: 4-12.3) for MWA ( p = 0.21) (Table 1 ).
Within the complete cohort, a subset of three patients underwent three TA sessions, whereas a larger group of 30 patients participated in two sessions. The most frequently observed symptoms following the procedure included palpitations in 8 patients (16%), weight loss in 5 patients (10%), and fatigue in 3 patients (6%). During the study period, the overall incidence of post-procedure complications was 20% ( n = 10). Among the subgroups, the RFA group had a lower incidence of complications at 13.8% ( n = 4), compared to 28.6% ( n = 6) in the MWA group. The primary complications manifested as tachycardia in five patients (10%), transient dysphonia in another five (10%), and skin numbness around the oral and limb areas in two patients (4%). Post-procedure tachycardia was successfully managed with propranolol and generally resolved within a week. By the time of the one-year follow-up, 96% of participants had achieved a euthyroid state, substantiating the efficacy of the TA procedure. Among the five patients presenting with GO, only two exhibited active GO and were pre-emptively administered prophylactic glucocorticoids prior to undergoing TA. Subsequent to the TA procedure, no further progression of GO was observed in these patients (Tables 2 and 3 ).
Thyroid volume
The study monitored 50 patients over a 12-month period, carefully tracking the changes in thyroid gland volume. At baseline, the median thyroid volume stood at 23.7 cm³ (IQR: 14.5–36.7). Following the ultrasound-guided TA, we observed a substantial decrease in thyroid volume at multiple time points. Specifically, the median volume reduced to 18.8 cm³ (IQR: 11.8–29.5), 16.3 cm³ (IQR: 10.6–24.1), 14.8 cm³ (IQR: 9.1–22.9), and 13.3 cm³ (IQR: 8.6–18.9) at 1-, 3-, 6-, and 12-months post-procedure, respectively. Furthermore, we calculated the post-procedure thyroid VRR to quantify the effectiveness of the treatment. The VRR was 17.5% (IQR: 13.0–23.0), 26.5% (IQR: 21.3–32.9), 34.4% (IQR: 28.1–41.2), and 39.8% (IQR: 33.2–47.1) at 1-, 3-, 6-, and 12-months post-procedure, respectively (Fig. 1 and Tables S1 and S2 ). The data clearly illustrate a consistent and statistically significant reduction in thyroid volume over successive time points, substantiating the efficacy of TA in treating GD. Importantly, all patients reported relief from neck compression symptoms during the follow-up period, further validating the therapeutic benefits of the procedure. Additional radial data relating to thyroid volume can be found in Supplementary Tables S3 - S5 .
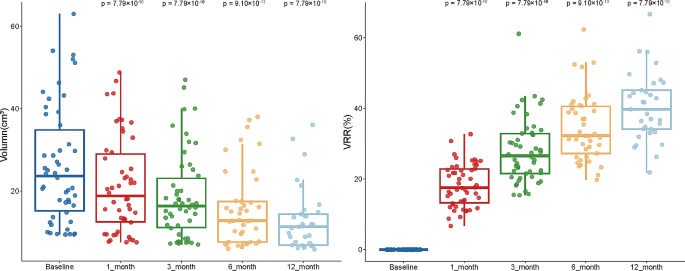
Volumetric reduction after thyroid thermal ablation. Box plots showing the variation of thyroid volume ( A ) and VRR ( B ) over pre-procedure and follow-up as medians ± one quartile, with the whiskers extending from the hinge to the smallest or largest value within 1.5 IQR from the box boundaries. The sample sizes of TA are 50, 50, 41, and 33, respectively. Differences between the two groups are tested using paired Wilcoxon test
Thyroid hormones
Pre-procedure assessments revealed elevated levels of T3, T4, and TRAb in all patients, with median values of free triiodothyronine (FT3): 13.500 pmol/L (IQR: 11.688–16.248), free triiodothyronine (FT4): 31.380 pmol/L (IQR: 27.118–35.637), and TRAb: 30.855 IU/L (IQR: 20.970-36.573). Concurrently, the TSH levels were markedly lower than those in healthy individuals, with a median value of TSH: 0.003 mIU/L (IQR: 0.003–0.003). Likewise, there were considerable reductions in thyroid hormone levels, supporting that TA effectively modulates thyroid function. One-month post-procedure, we observed a significant increase in TSH levels to a median of 2.439 mIU/L (IQR: 0.531–3.805; p = 7.75 × 10 − 10 ). By the one-year mark, TSH levels had normalized, with a median value of 3.011 mIU/L (IQR: 2.130–4.291; p = 7.77 × 10 − 10 ). Nevertheless, we observed a marked elevation in TRAb levels one month following the surgical intervention ( p = 3.80 × 10 − 10 ). Subsequently, both TRAb and thyroid hormone levels declined, reaching the normal physiological level ( p = 9.93 × 10 − 10 ). This confirms that ultrasound-guided TA has a long-term positive impact on thyroid function (Table 4 , S6 , and S7 ).
Basal metabolic rate
To ensure patient safety during the procedure, medications such as ATDs, propranolol, and potassium iodide were administered to regulate heart rate and cardiac pressure. The Basal Metabolic Rate (BMR) was deliberately lowered to below 20% prior to the procedure as a precautionary measure against peri-procedural risks. Our data showed a significant drop in BMR levels at 1, 3, 6, and, 12 months post-procedure, aligning with changes in thyroid hormones and corroborating the therapeutic efficacy of TA for managing GD. In summary, our study provides compelling evidence for the efficacy and safety of ultrasound-guided TA in the treatment of GD, offering a promising alternative to traditional surgical interventions (Table 5 and S8 ).
Hyperthyroidism is a complex medical condition characterized by excessive secretion and synthesis of thyroid hormones that manifests through the thyroid gland’s excessive secretion and synthesis of thyroid hormones. This hormonal imbalance has far-reaching implications, affecting a multitude of physiological systems, such as the nervous, circulatory, and digestive systems. A wide array of symptoms manifest, including but not limited to tachycardia, tremors in the extremities, significant weight loss, and specialized conditions like GO and thyroid dermopathy. These symptoms present not just medical challenges but also severely hamper the patient’s overall quality of life [ 27 ]. Within this complex landscape, GD stands out as a particularly challenging autoimmune form of hyperthyroidism. In GD, TSAb bind to the TSH receptor, setting off a cascade of events that result in uncontrolled synthesis and secretion of thyroid hormones [ 17 ].
The traditional therapeutic landscape for managing hyperthyroidism has largely been dominated by long-term treatments involving ATDs. Another longstanding approach has been RAI therapy, which uses radiation to selectively damage thyroid follicular cells, thereby inhibiting excessive hormone synthesis. Our research posits ultrasound-guided TA as a groundbreaking, minimally invasive alternative to conventional surgical thyroidectomy. One of the most striking findings was the significantly shorter duration of the TA procedure, with a median time of 47 min (IQR: 40-53.25). This stands in stark contrast to the traditional thyroidectomy, which requires a considerably longer average duration of around 123.2 ± 43.0 min [ 21 ]. It is worth mentioning that by the end of the one-year follow-up period, 96% of the participants had achieved a euthyroid state, with no patients exhibiting hypothyroidism or requiring levothyroxine replacement, further substantiating the long-term efficacy of the TA procedure. This demonstrates its potential as an effective and enduring alternative treatment for hyperthyroidism.
Our findings indicate that TA procedures are correlated with a reduced incidence of postoperative complications when contrasted with traditional surgical techniques [ 21 ]. This is likely due to the procedure being performed within the confines of the thyroid capsule, thus limiting the risk of injury to the parathyroid glands and other adjacent tissues. Although patients reported transient soft tissue edema, likely due to the use of 1% lidocaine, these symptoms generally resolved within 48 h, making them a manageable side effect.
Although the TA procedure successfully reduced thyroid gland volume and increased the VRR, certain patients expressed lingering aesthetic concerns, as outlined in Fig. 1 and Tables S1 and S2 . Unlike surgical interventions, which remove the enlarged thyroid gland entirely, TA only reduces the gland’s size, leaving some patients dissatisfied with the immediate aesthetic outcome. Nevertheless, TA treatment significantly reduced the levels of thyroid hormones in patients, bringing them to normal levels. For instance, our data revealed an initial surge in TRAb levels following the surgical procedure, which was subsequently followed by a statistically significant decline. This suggests that the necrotic tissue resulting from TA may have a lower immunogenicity, potentially reducing the chances of exacerbated autoimmune responses (Table 4 , S6 and S7 ). Furthermore, TA stabilized the patients’ BMR, which was consistent with the changes observed in thyroid hormones (Tables 4 and 5 ).
Ultrasound-guided TA offers several advantages in terms of safety, particularly in minimizing the risk of injury to surrounding tissues. One advantage of ultrasound guidance is that it effectively prevents direct damage to the surrounding skin, trachea, blood vessels, and nerves by the active tip. Another benefit is that the isolation zone established by 0.5% lidocaine in the interstitial space can avoid thermal damage caused by heat conduction to adjacent tissues [ 28 ]. However, it is crucial to acknowledge that TA, like any medical procedure, comes with its own set of risks, including potential post-procedure complications such as bleeding and voice changes due to RLN injury.
Compared with ATD and RAI, TA has numerous advantages, including no need for long-term medication, low recurrence rate, no blood cell deficiency, and no drug-induced liver injury. In addition, pregnant or breastfeeding patients can also use TA without the risk of exacerbating GO. However, there are still some disadvantages of TA, such as a broader range of injuries and the risk of post-procedure complications, including bleeding, voice changes caused by recurrent laryngeal nerve injury, parathyroid injury, and thyroid storm.
Compared with surgery, TA has several advantages, including cosmetic benefits, minor trauma, quick post-procedure recovery, low cost, fewer post-procedure complications, and a low incidence of hypothyroidism [ 29 ]. However, there may be a higher recurrence rate of hyperthyroidism. Additionally, TA still has some limitations and issues, such as the lack of medium and long-term follow-up data from large cohorts, the inability to address the cosmetic issue of an enlarged thyroid gland immediately, and the specific risk of adjacent tissue damage during the procedure. The amalgamation of imaging data from diverse sources like CT scans, MRI, contrast-enhanced ultrasonography, and (CDFI) could provide a more comprehensive evaluation of TA’s effectiveness and scope [ 30 ], thus reducing the incidence of multiple ablations. MRI assessment of thyroid volume changes before and after TA helps evaluate thyroid volume changes. Contrast-enhanced ultrasonography can better assess the perfusion of thyroid tissue before and after the procedure to evaluate the efficacy of TA. However, our department has yet to carry out a contrast-enhanced ultrasound project, an area for future research.
Despite the excellent short-term efficacy of TA, the long-term efficacy remains unknown. There is still a lack of large-sample, multi-center, and prospective clinical studies on TA treatment. Furthermore, the inclusion and diagnosis criteria and treatment standards need to be standardized to improve the accuracy and reliability of the research.
Our study accentuates the high efficacy, safety, and tolerability of ultrasound-guided TA as a therapeutic approach for managing GD-induced hyperthyroidism. Notably, TA demonstrates marked efficacy in symptom alleviation, a significant reduction in thyroid gland volume, and the successful normalization of thyroid hormone levels and BMR. When juxtaposed against conventional surgical thyroidectomy, TA appears to offer several advantages, including reduced physical trauma, fewer post-procedure complications, and enhanced aesthetic outcomes. As such, ultrasound-guided TA emerges as a compelling alternative to surgical interventions for hyperthyroidism patients. Nevertheless, it is crucial to recognize that a knowledge gap still exists concerning the long-term efficacy of TA treatment. Therefore, future research should prioritize large-scale, multi-center, prospective clinical trials aimed at standardizing diagnostic criteria and treatment protocols for TA in hyperthyroidism management.
Data availability
The data used in this study will be made available upon request to the corresponding author. Access to the data will be subject to signing a data use agreement and approval from the institutional review board. Requests for data should be directed to [email protected].
Milo T, Korem Kohanim Y, Toledano Y, Alon U. Autoimmune thyroid diseases as a cost of physiological autoimmune surveillance. Trends Immunol. 2023;44(5):365–71.
Article CAS PubMed Google Scholar
Song Y, Wang X, Ma W, Yang Y, Yan S, Sun J, et al. Graves’ disease as a driver of depression: a mechanistic insight. Front Endocrinol (Lausanne). 2023;14:1162445.
Article PubMed Google Scholar
Smith TJ, Hegedüs L. Graves’ Disease. N Engl J Med. 2016;375(16):1552–65.
McLeod DS, Cooper DS. The incidence and prevalence of thyroid autoimmunity. Endocrine. 2012;42(2):252–65.
Sjölin G, Holmberg M, Törring O, Byström K, Khamisi S, de Laval D, et al. The long-term outcome of treatment for Graves’ hyperthyroidism. Thyroid. 2019;29(11):1545–57.
El Kawkgi OM, Ross DS, Stan MN. Comparison of long-term antithyroid drugs versus radioactive iodine or surgery for Graves’ disease: a review of the literature. Clin Endocrinol (Oxf). 2021;95(1):3–12.
Ciarallo A, Rivera J. Radioactive Iodine Therapy in differentiated thyroid Cancer: 2020 update. AJR Am J Roentgenol. 2020;215(2):285–91.
Zhang D, Park D, Sun H, Anuwong A, Tufano R, Kim HY, et al. Indications, benefits and risks of transoral thyroidectomy. Best Pract Res Clin Endocrinol Metab. 2019;33(4):101280.
Guo Z, Yu P, Liu Z, Si Y, Jin M. Total thyroidectomy vs bilateral subtotal thyroidectomy in patients with Graves’ diseases: a meta-analysis of randomized clinical trials. Clin Endocrinol (Oxf). 2013;79(5):739–46.
Chu KF, Dupuy DE. Thermal ablation of tumours: biological mechanisms and advances in therapy. Nat Rev Cancer. 2014;14(3):199–208.
Han W, Liu F, Liu G, Li H, Xu Y, Sun S. Research progress of physical transdermal enhancement techniques in tumor therapy. Chem Commun (Camb). 2023;59(23):3339–59.
Kim JH, Baek JH, Lim HK, Ahn HS, Baek SM, Choi YJ, et al. 2017 thyroid radiofrequency ablation Guideline: Korean society of thyroid Radiology. Korean J Radiol. 2018;19(4):632–55.
Article PubMed PubMed Central Google Scholar
Chen Z, Zhang W, He W. Ultrasound-guided thermal ablation for papillary thyroid microcarcinoma: a systematic review. Clin Endocrinol (Oxf). 2023;98(3):296–305.
Li HX, Xiang N, Hu WK, Jiao XL. Relation between therapy options for Graves’ disease and the course of Graves’ ophthalmopathy: a systematic review and meta-analysis. J Endocrinol Invest. 2016;39(11):1225–33.
Bautista-Orduno KG, Dorsey-Trevino EG, Gonzalez-Gonzalez JG, Castillo-Gonzalez DA, Garcia-Leal M, Raygoza-Cortez K et al. American thyroid association guidelines are inconsistent with Grading of Recommendations Assessment, Development, and Evaluations-A meta-epidemiologic study. J Clin Epidemiol. 2020;123:180-8.e2.
Ross DS, Burch HB, Cooper DS, Greenlee MC, Laurberg P, Maia AL, et al. 2016 American Thyroid Association Guidelines for Diagnosis and management of hyperthyroidism and other causes of thyrotoxicosis. Thyroid. 2016;26(10):1343–421.
Kahaly GJ, Bartalena L, Hegedüs L, Leenhardt L, Poppe K, Pearce SH. 2018 European thyroid Association Guideline for the management of Graves’ hyperthyroidism. Eur Thyroid J. 2018;7(4):167–86.
Article CAS PubMed PubMed Central Google Scholar
National Institute for Health and Care Excellence. Guidelines. Thyroid disease: assessment and management. London: National Institute for Health and Care Excellence (NICE) Copyright © NICE 2019.; 2019.
Brunn J, Block U, Ruf G, Bos I, Kunze WP, Scriba PC. [Volumetric analysis of thyroid lobes by real-time ultrasound (author’s transl)]. Deutsche medizinische Wochenschrift (1946). 1981;106(41):1338-40.
Henry CJ. Basal metabolic rate studies in humans: measurement and development of new equations. Public Health Nutr. 2005;8(7a):1133–52.
Frank ED, Park JS, Watson W, Chong E, Yang S, Simental AA. Total thyroidectomy: safe and curative treatment option for hyperthyroidism. Head Neck. 2020;42(8):2123–8.
Ha EJ, Baek JH, Lee JH. Moving-shot versus fixed electrode techniques for radiofrequency ablation: comparison in an ex-vivo bovine liver tissue model. Korean J Radiol. 2014;15(6):836–43.
Lin WC, Tai YF, Chen MH, Luo SD, Huang F, Chen WC et al. Ultrasound-guided moving Shot Radiofrequency ablation of Benign Soft tissue neoplasm. Med (Kaunas). 2021;57(8).
Park HS, Baek JH, Park AW, Chung SR, Choi YJ, Lee JH. Thyroid radiofrequency ablation: updates on innovative devices and techniques. Korean J Radiol. 2017;18(4):615–23.
Piantanida E. Preoperative management in patients with Graves’ disease. Gland Surg. 2017;6(5):476–81.
Calissendorff J, Falhammar H. Lugol’s solution and other iodide preparations: perspectives and research directions in Graves’ disease. Endocrine. 2017;58(3):467–73.
Wiersinga WM, Poppe KG, Effraimidis G. Hyperthyroidism: aetiology, pathogenesis, diagnosis, management, complications, and prognosis. Lancet Diabetes Endocrinol. 2023;11(4):282–98.
Chung SR, Baek JH, Choi YJ, Lee JH. Management strategy for nerve damage during radiofrequency ablation of thyroid nodules. Int J Hyperthermia: Official J Eur Soc Hyperthermic Oncol North Am Hyperth Group. 2019;36(1):204–10.
Article Google Scholar
Jin H, Lin W, Lu L, Cui M. Conventional thyroidectomy vs thyroid thermal ablation on postoperative quality of life and satisfaction for patients with benign thyroid nodules. Eur J Endocrinol. 2021;184(1):131–41.
Orloff LA, Noel JE, Stack BC Jr., Russell MD, Angelos P, Baek JH, et al. Radiofrequency ablation and related ultrasound-guided ablation technologies for treatment of benign and malignant thyroid disease: an international multidisciplinary consensus statement of the American Head and Neck Society Endocrine Surgery Section with the Asia Pacific Society of thyroid surgery, Associazione Medici Endocrinologi, British Association of Endocrine and thyroid surgeons, European Thyroid Association, Italian Society of endocrine surgery units, Korean Society of Thyroid Radiology, latin American thyroid Society, and Thyroid Nodules Therapies Association. Head Neck. 2022;44(3):633–60.
Download references
Acknowledgements
We sincerely thank our colleagues for their valuable contributions to this study: JS and LZ helped design this work. GL, XC, and ZH extracted the information from the databases and analyzed the data. PL, WL, MW, and HY supervised the entire study. Their dedication and expertise were crucial to the success of this project. We would also like to thank the patients who participated in this study for allowing us to collect their medical data. Without their cooperation and support, this research would not have been possible. This retrospective, single-institutional study was conducted with the approval of the Institutional Review Board of Xiamen Medical University.
There was no funding or foundation support for this research.
Author information
Guangzhen Cai and Beilin Luo contributed equally to this work.
Authors and Affiliations
Department of General Surgery, The Second Affiliated Hospital of Xiamen Medical College, 566#, Shengguang Road, 361021, Xiamen, P.R. China
Guangzhen Cai, Maolin Wang, Jiqin Su, Guibin Li, Xiangru Chen, Zhishu Huang, Peiyi Lin, Shengwei Liu, Huidi Yan & Lixin Zhou
The Graduate School of Fujian Medical University, The Second Affiliated Hospital of Xiamen Medical College, 88#, Jiaotong Road, 350005, Fuzhou, P.R. China
Department of Endocrinology, The Second Affiliated Hospital of Xiamen Medical College, 566#, Shengguang Road, 361021, Xiamen, P.R. China
You can also search for this author in PubMed Google Scholar
Contributions
GC, JS, and LZ designed this study. BL, GL, XC, and ZH extracted the information from the databases and analyzed the data. BL, and GC completed the data verification. PL, WL, MW, and HY supervised the entire study. BL, and GC wrote the manuscript. All authors revised the manuscript.
Corresponding author
Correspondence to Lixin Zhou .
Ethics declarations
Competing interests.
The authors declare no competing interests.
Additional information
Publisher’s note.
Springer Nature remains neutral with regard to jurisdictional claims in published maps and institutional affiliations.
Electronic supplementary material
Below is the link to the electronic supplementary material.
Supplementary Material 1
Rights and permissions.
Open Access This article is licensed under a Creative Commons Attribution 4.0 International License, which permits use, sharing, adaptation, distribution and reproduction in any medium or format, as long as you give appropriate credit to the original author(s) and the source, provide a link to the Creative Commons licence, and indicate if changes were made. The images or other third party material in this article are included in the article’s Creative Commons licence, unless indicated otherwise in a credit line to the material. If material is not included in the article’s Creative Commons licence and your intended use is not permitted by statutory regulation or exceeds the permitted use, you will need to obtain permission directly from the copyright holder. To view a copy of this licence, visit http://creativecommons.org/licenses/by/4.0/ . The Creative Commons Public Domain Dedication waiver ( http://creativecommons.org/publicdomain/zero/1.0/ ) applies to the data made available in this article, unless otherwise stated in a credit line to the data.
Reprints and permissions
About this article
Cite this article.
Cai, G., Luo, B., Wang, M. et al. Efficacy and safety of ultrasound-guided thermal ablation of graves’ disease: a retrospective cohort study. Thyroid Res 17 , 10 (2024). https://doi.org/10.1186/s13044-024-00198-4
Download citation
Received : 23 September 2023
Accepted : 13 April 2024
Published : 03 June 2024
DOI : https://doi.org/10.1186/s13044-024-00198-4
Share this article
Anyone you share the following link with will be able to read this content:
Sorry, a shareable link is not currently available for this article.
Provided by the Springer Nature SharedIt content-sharing initiative
- Graves' disease
- Thermal ablation
- Retrospective study
- Efficacy assessment
Thyroid Research
ISSN: 1756-6614
- General enquiries: [email protected]

COMMENTS
A matched cohort study involves pairs (or clusters in case several untreated subjects are matched with each of the treated individuals) formed to include individuals who differ with respect to treatment but may be matched on certain baseline characteristics.
Retrospective cohort study. Case-control study versus cohort on a timeline. "OR" stands for "odds ratio" and "RR" stands for "relative risk". A retrospective cohort study, also called a historic cohort study, is a longitudinal cohort study used in medical and psychological research. A cohort of individuals that share a common exposure factor ...
A retrospective cohort study is a type of observational study that focuses on individuals who have an exposure to a disease or risk factor in common.
The two study designs have similar statistical precision for addressing a singular research question, but case-cohort studies have broader efficiency and superior flexibility. Despite this, case-cohort designs are comparatively underutilized in the epidemiologic literature.
General Overview of Case-Control Studies In observational studies, also called epidemiologic studies, the primary objective is to discover and quantify an association between exposures and the outcome of interest, in hopes of drawing causal inference. Observational studies can have a retrospective study design, a prospective design, a cross-sectional design, or a combination of all three. In a ...
We conducted a matched retrospective cohort study of two cohorts to estimate inactivated vaccine effectiveness (VE) and its comparative effectiveness of booster dose among older people in Shanghai.
Chemoradiation versus cystectomy for locally advanced bladder cancer: A global, propensity-matched retrospective cohort study.
A retrospective, hospital-based cohort study was conducted to assess treatment response in ITP patients who were on follow-up in the hematology clinic of TASH and SPHMMC during the study period.
In this matched retrospective cohort study, respiratory outcomes were compared among patients with active asthma, resolved asthma, and non-asthma controls. Patients with resolved asthma had significantly fewer hospital and general practice-attended asthma exacerbations than those with active asthma.
An overview of Case-control and Cohort studies: what are they, how are they different, and what are the pros and cons of each study design.
A nested case-control study is an efficient design that can be embedded within an existing cohort study or randomised trial. It has a number of advantages compared to the conventional case-control design, and has the potential to answer important research questions using untapped prospectively collected data.
There are two common misconceptions about case-control studies: that matching in itself eliminates (controls) confounding by the matching factors, and that if matching has been performed, then a "matched analysis" is required. However, matching in a case-control study does not control for confounding by the matching factors; in fact it can introduce confounding by the matching factors even ...
General Overview of Case-Control Studies. In observational studies, also called epidemiologic studies, the primary objective is to discover and quantify an association between exposures and the outcome of interest, in hopes of drawing causal inference. Observational studies can have a retrospective study design, a prospective design, a cross ...
This self-controlled case series (SCCS) and matched cohort study was done in Sweden. The personal identification numbers of all patients with COVID-19 in Sweden from Feb 1 to Sept 14, 2020, were identified and cross-linked with national inpatient, outpatient, cancer, and cause of death registers.
This was a propensity score-matched, retrospective cohort study using linked population-based administrative health data for adults admitted to designated psychiatric facilities in Ontario, Canada, for more than 3 days with depression between April 1, 2007, to Feb 28, 2017. Electroconvulsive therapy exposure was defined as one or more physician billing procedure codes during hospitalisation ...
Matching is mainly used in observational studies, including case-control and cohort studies. Matching is a technique by which patients with and without an outcome of interest (in case-control studies) or patients with and without an exposure of interest (in cohort studies) are sampled from an underlying cohort to have the same or similar distributions of characteristics such as age and sex.
Purpose This study aims to compare treatment outcomes between neoadjuvant chemotherapy (NACT) followed by surgery and concurrent chemoradiotherapy (CCRT) in patients with stage IIB cervical squamous cell carcinoma (CSCC). Materials and methods We conducted a retrospective cohort study involving patients with stage IIB CSCC treated at Guangxi Medical University Cancer Hospital between June 2012 ...
This article reports on similarities and differences in findings of randomized controlled trials (RCTs), case-matched studies, and cohort studies, as revealed by meta-analyses of previously published...
A binational population-based cohort study was conducted to analyze the risk of acute respiratory complications or post-acute respiratory sequelae after SARS-CoV-2 infection.
Comparison of intranasal dexmedetomidine alone and dexmedetomidine-chloral hydrate combination sedation for electroencephalography in children: A large retrospective cohort study and propensity score-matched analysis
A matched cohort study involves pairs (or clusters in case several untreated subjects are matched with each of the treated individuals) formed to include individuals who differ with respect to treatment but may be matched on certain baseline characteristics.
We conducted a retrospective cohort study to assess the effectiveness of booster vaccination of inactivated COVID-19 vaccines against be infected (susceptibility), infecting others (infectiousness), and disease transmission among individuals (transmission) in Shenzhen during an Omicron BA.2 outbreak period from 1 February to 21 April 2022.
Case-control studies are one of the major observational study designs for performing clinical research. The advantages of these study designs over other study designs are that they are relatively quick to perform, economical, and easy to design and implement. Case-control studies are particularly appropriate for studying disease outbreaks, rare diseases, or outcomes of interest. This article ...
Despite these advantages, there is a paucity of research evaluating the efficacy and safety of ultrasound-guided TA specifically for GD. This study aims to fill this gap by conducting a retrospective cohort study of GD patients treated with TA at our center between October 2017 and December 2021.
This retrospective cohort study analyzes prospectively collected data from patients' medical records. Data on all individuals admitted at Clinical Hospital No. 1 of the President's Administration of the Russian Federation between 2012 and 2018 with confirmed DVT and implanted IVC filter were included.